Fig. 1.1
Mouse heart development. Cartoon showing the major events in mouse heart development. (a–d) From the cardiac crescent (E7.5, a), through linear (E8, b) and looping (E8.5, c) stages to the midgestation embryonic heart tube (E10.5, d). The cardiac crescent and its linear heart tube and left ventricular derivatives are pink and the second heart field and derivatives green (arterial pole) and blue (venous pole). At midgestation, atrial and ventricular chamber myocardium (red) expands by proliferation at the outer curvature of the heart tube (e). Cardiac septation is underway at E11.5 (f); the interventricular and aorticopulmonary septa and outflow tract cushions (asterisks) are evident and the outflow tract rotates in a counterclockwise direction (arrow). Cardiac septation is complete by E14.5 (g), including the division of the outflow tract into the ascending aorta and pulmonary trunk. Cardiac cushions have remodeled to form atrioventricular (tricuspid and mitral) and outlet valves (aortic and pulmonary). At this stage, the differentiation of the cardiac conduction system is underway (h); the arrow shows the direction of propagation of the electrical signal in atrial myocardium. Epicardium spreads over the ventricular surface from the pro-epicardial organ from E10.5 (i, right lateral view), and sinus venosus-derived cells contribute to coronary vascular endothelium (Cartoons a–i adapted from [1]). Abbreviations: CF cephalic folds, AO aorta, AP arterial pole, APS aorticopulmonary septum, AV aortic valve, AVB atrioventricular bundle, AVC atrioventricular canal, AVN atrioventricular node, CC cardiac crescent, EP epicardium, IC inner curvature, IVS interventricular septum, LA left atrium, LV left ventricle, MV mitral valve, HT heart tube, OC outer curvature, OFT outflow tract, PA1 first pharyngeal arch, PA2 second pharyngeal arch, PEO pro-epicardial organ, PF Purkinje fiber network, PT pulmonary trunk, PV pulmonary valve, RA right atrium, RV right ventricle, SAN sinoatrial node, SHF second heart field, SV sinus venosus-derived endothelial cells, T trabeculae, TV tricuspid valve, VP venous pole
These early differentiating myocytes form the linear heart tube, with a posterior venous pole and anterior arterial pole, surrounding an endocardial tube; the linear heart tube gives rise to the apex of the left ventricle in the definitive heart (Fig. 1.1b). Cardiac progenitor cells in pharyngeal mesoderm adjacent to the early heart tube subsequently add to the growing cardiac poles, giving rise to right ventricular and outflow tract myocardium at the arterial pole and atrial myocardium at the venous pole [7]. These progenitor cells, known as the second heart field (Chap. 3) and discovered in 2001, thus form the major part of the heart, including the entire outflow tract or conotruncal region, connected to the pharyngeal arch arteries. Progressive addition of second heart field cells is regulated by diverse signals from pharyngeal epithelia and neural crest-derived mesenchyme that are in turn integrated by transcription factors such as that encoded by the 22q11.2 deletion (or DiGeorge) syndrome gene T-box 1 (TBX1) [8–10]. Defective addition of second heart field cells results in a spectrum of common congenital heart defects, ranging from conotruncal anomalies such as tetralogy of Fallot and double outlet right ventricle to venous poles defects including atrial and atrioventricular septal defects. As the heart tube elongates, it loops to the right, the first morphological manifestation of the embryonic left-right axis (Fig. 1.1c). The left–right axis (Chap. 7) is established in the node during gastrulation and results in the activation of lateralized signaling information that is transmitted to asymmetrically developing organs such as the heart [11]. Dissection of the regulation of second heart field deployment and the establishment and transmission of laterality information are considered to be key steps in understanding the mechanisms underlying common forms of CHD [12].
1.3 Ballooning Morphogenesis and Cardiac Septation
By midgestation (E10.5), looping and heart tube extension are complete (Fig. 1.1d). Subsequent growth of the heart occurs by proliferation of atrial and ventricular cardiomyocytes in the outer curvature of the heart tube, a process known as the ballooning model of chamber morphogenesis (Fig. 1.1e). Patterned proliferation in the embryonic heart is regulated by transcription factors, in particular members of the T-box family, that are expressed in overlapping patterns in the early heart and define the atrioventricular canal and outflow tract as regions of low proliferation where endocardial cushions develop [13]. Cushions contain mesenchymal cells that are derived from the endocardium by a process of epithelial to mesenchymal transformation in response to overlying myocardial signals. The atrioventricular canal and outflow tract regulate directional blood flow through the early heart by valve-like cushion function and reduced myocardial electrical coupling. At this stage, cardiac septation initiates, dividing the heart into separate left and right chambers. The primary atrial septum converges with the developing endocardial cushions in the atrioventricular region. The addition of second heart field progenitor cells at the venous pole (Chap. 5) of the heart is essential for the formation of the muscular base of the primary atrial septum and plays a critical role in atrioventricular septation [14]. Atrioventricular cushions also connect with the interventricular septum, developing at the interface between linear heart tube and second heart field-derived myocardium, i.e., future left (systemic) and right (pulmonary) ventricles. At the arterial pole, the influx of cardiac neural crest cells (Chap. 4) is essential for the division of the outflow tract into the ascending aorta and pulmonary trunk (outlets of the left and right ventricles) through the formation of the aorticopulmonary septum and outflow tract cushion fusion (Fig. 1.1f). Defects in neural crest cell development result in a spectrum of conotruncal defects including common arterial trunk [15].
The endocardial cushions are remodeled during septation to form the definitive tricuspid, mitral, aortic, and pulmonary valves that regulate blood to and from the ventricular chambers (Fig. 1.1g). The conotruncal region rotates in a counterclockwise direction under the influence of the embryonic laterality cascade, and the aorta becomes aligned with the left ventricle through a process termed wedging. Perturbation of cardiac septation results in failure to make the exclusive connection between the ascending aorta and pulmonary trunk that is essential to separate the systemic and pulmonary circulatory systems at birth. Defects in either heart tube elongation that generates the template for septation or in the septation process itself thus result in a range of CHD. Systemic and pulmonary circulatory systems become fully isolated at birth by the closure of the oval foramen and ductus arteriosus.
1.4 Development of the Conduction System and Coronary Circulation
Chamber development is accompanied by establishment of the definitive cardiac conduction system (Chap. 8) that regulates cardiac rhythm (Fig. 1.1h). Electrical activity is triggered by the pacemaker, or sinoatrial node, in the sinus venous region. Conduction between the atria and ventricles is delayed by the atrioventricular node, ensuring sequential atrial and ventricular contraction. The electrical signal initiates apical ventricular contraction through the atrioventricular bundle, bundle branches, and Purkinje fiber network. Conductive cells are specialized myocytes and share common progenitor cells with nonconducting myocytes [16]. Defects in the establishment of the electrical wiring of the heart result in arrhythmias and conduction blocks. The Purkinje fiber system develops adjacent to the ventricular lumen in trabecular myocytes, transient projections of myocardium that form by signal exchange between the myocardial wall and endocardium. Trabeculae are present in the mouse heart from midgestation to fetal stages when a process of compaction generates the definitive ventricular wall. Failure of this step results in noncompaction cardiomyopathy.
From E10.5, a third cell layer, the epicardium (Chap. 6), covers the external surface of the heart (Fig. 1.1i). The epicardium originates in the pro-epicardial organ and forms an epithelium from which cells undergo a mesenchymal transition and invade the myocardium, giving rise to smooth muscle cells associated with coronary arteries and cardiac fibroblasts. Signal exchange between the epicardium, epicardially derived fibroblasts, and myocardium plays an important role in regulating myocardial proliferation and differentiation. Coronary vasculature, essential for the delivery of oxygen and nutrients to the myocardium, develops during fetal stages [17]. The origin of coronary endothelial cells is controversial; however, sinus venosus endothelium and endocardial trapping during compaction are thought to be two major sources. Coronary smooth muscle cells originate from the epicardium as well as neural crest-derived cells. Coronary arteries connect with the base of the ascending aorta to form right and left coronary ostia. Abnormal proximal coronary artery patterning, often associated with conotruncal CHD, is a cause of sudden cardiac death.
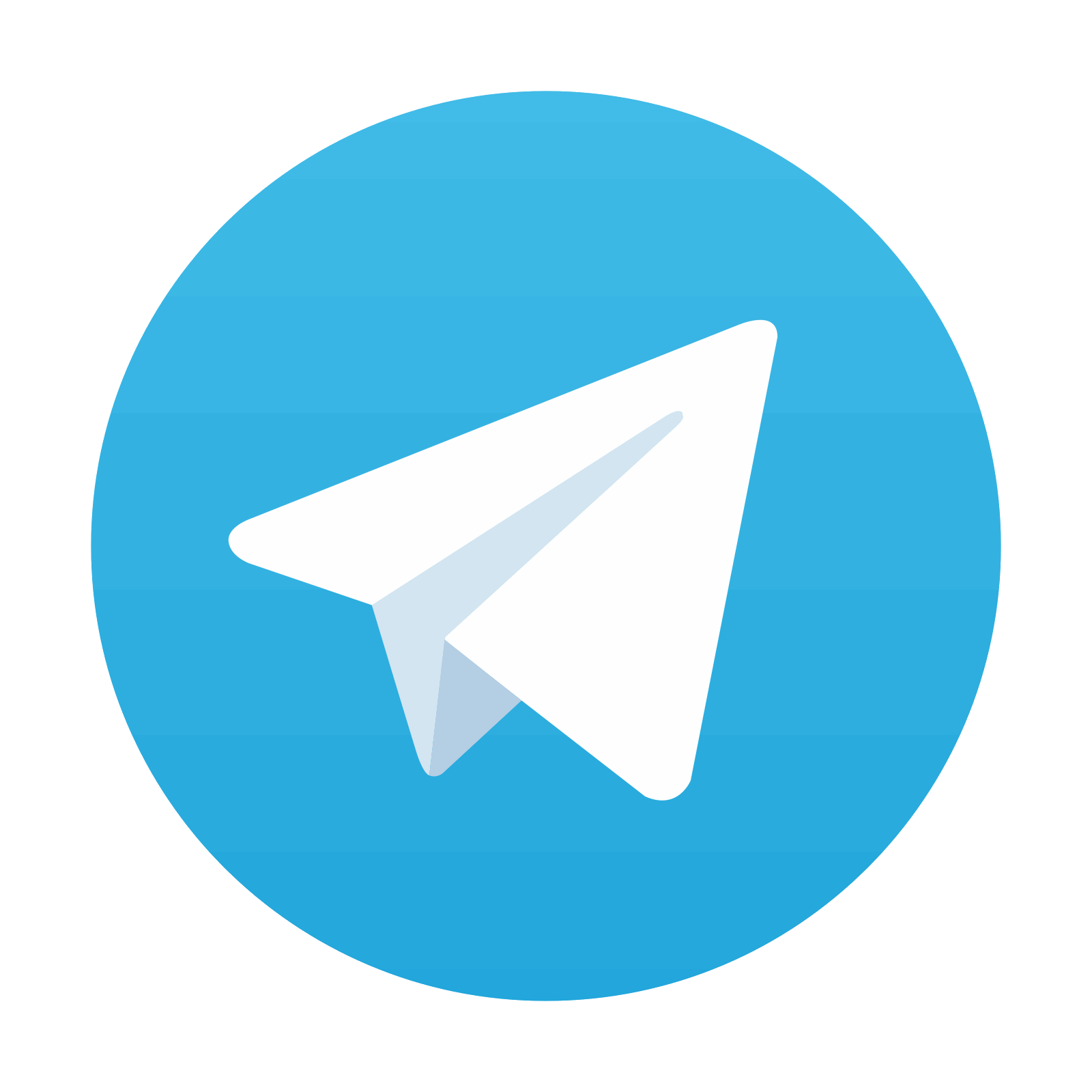
Stay updated, free articles. Join our Telegram channel

Full access? Get Clinical Tree
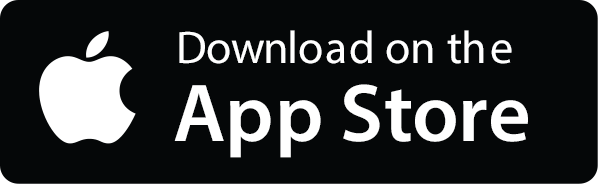
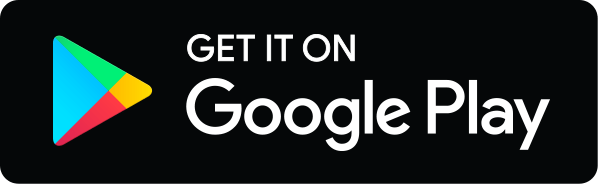