8 Cardiac Computed Tomography and Magnetic Resonance Imaging
Cardiac Computed Tomography
Clinical Indications
Coronary Artery Calcium Score
Coronary artery calcium (CAC) is recognized as a marker of subclinical atherosclerosis. CAC scoring utilizes no contrast and readily detects calcium because of its high x-ray attenuation coefficient (or CT number) measured in Hounsfield units (HU) (Fig. 8-1). The Agatston scoring system assigns a calcium score based on maximal CT number and the area of calcium deposits. Initially promoted as part of a screening paradigm, CAC was originally made available for patient-initiated evaluation of coronary risk on a fee-for-service basis. More recently, analysis of several large clinical datasets has confirmed that the “coronary calcium score” is a predictor of coronary events, independent of traditional risk factors. In at least one study, calcium score was more predictive than C-reactive protein and standard risk factors for predicting CAD events.
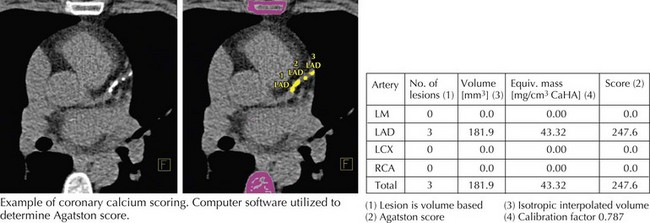
Figure 8-1 Coronary calcium scoring.
LAD, left anterior descending; LCX, left circumflex; LM, left main; RCA, right coronary artery.
CTA utilizes intravenous contrast to differentiate vessel lumen from vessel wall. In 2006, the ACC and many other societies with interests in cardiac imaging put together recommendations of “appropriateness criteria” for utilization of cardiac CTA that include appropriate (Box 8-1) and inappropriate uses of this technology. The most common appropriate utilization is diagnostic study of patients presenting with chest pain who do not have significant ECG changes or elevated cardiac biomarkers but have an intermediate probability of CAD. At experienced centers with careful data acquisition, sensitivities range from 83% to 99% and specificities from 93% to 98% with remarkably high estimated negative predictive value (95%–100%), indicating that CCT may be used to reliably rule out the presence of significant flow-limiting coronary atherosclerotic disease. It should be pointed out that CCT would be inappropriate for patients at high risk for or with other indications of cardiac ischemia such as elevated biomarkers or significant ECG changes. Those patients should be referred immediately for invasive imaging.
Box 8-1 Appropriate Indications for CCT
Structure and Function
CAD, coronary artery disease; CCT, cardiac computed tomography; ECG, electrocardiogram.
Bypass graft imaging is more easily accomplished than coronary artery imaging because of the larger size of bypass grafts (particularly saphenous vein grafts) and less rapid movement of bypass grafts as compared with native coronary arteries. The patency or occlusion of grafts can be determined by the presence or absence of distal target vessel contrast enhancement (Fig. 8-2
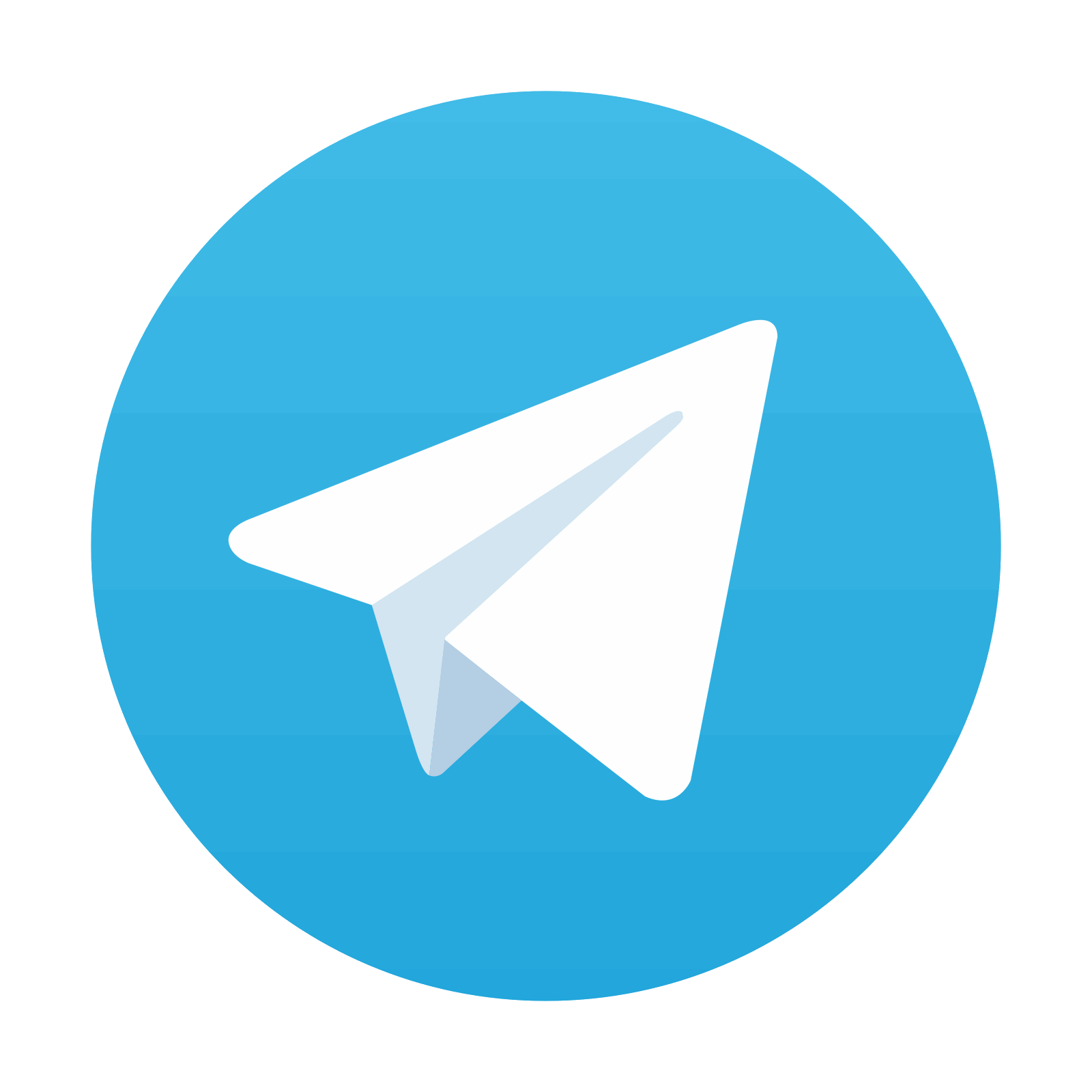
Stay updated, free articles. Join our Telegram channel

Full access? Get Clinical Tree
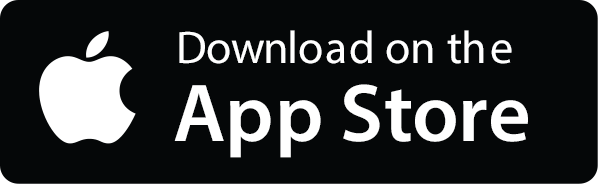
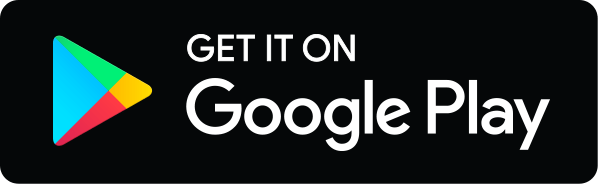