Acknowledgment
Dr. Horlick is supported by the Peter Munk Chair in Structural Heart Disease.
Cardiac catheterization remains a fundamental modality for the diagnosis and interventional treatment of adult congenital heart disease (ACHD); however, there has been a noticeable change in case mix and clinical demands for the ACHD interventionalist over the past several decades. Advances in imaging such as three-dimensional (3D)-echocardiography and the exquisite resolution of modern cardiac magnetic resonance imaging (MRI) provide anatomic and physiologic diagnosis for many ACHD patients. To the noninterventionalist, there is perhaps less appreciation for diagnostic catheterization’s place in patient care.
From a therapeutic perspective, the new millennium has brought a revolution in the structural interventional arena with the advent of transcatheter valve therapies, improved endovascular solutions for large vessel access and stenting, and real-time adjunctive cardiac imaging. In this chapter, we will discuss the following points:
- 1.
Modern-day catheterization and procedure preparation
- 2.
The ACHD catheterization laboratory
- 3.
Hemodynamics and angiography
- 4.
Select congenital interventions
- 5.
Future directions of ACHD catheterization
What Is the Role of Catheterization?
What is the role of catheterization in the modern day? What is its role in the presence of advanced imaging modalities?
Catheterization remains the gold standard of pressure measurement in a vessel or chamber. In contrast to the complexities of the newest imaging technology, the measurement of intracardiac pressures is simple, reliable, and reproducible. Prior to surgery or intervention when there are inconsistent noninvasive results, the hemodynamic significance of a lesion should be directly verified by catheterization. This minor procedure may provide critical adjunctive data that can alter management and enhance safety. Although the routine crossing of a stenotic aortic valve for diagnostic purposes is controversial, valvular hemodynamics remain an important part of the decision making in complex polyvalvular disease, for which noninvasive imaging is inadequate and when the magnitude of a proposed operation is in question. Anecdotal stories from every invasive cardiologist suggest uniformly that many patients have appropriately avoided surgery despite “certainty” of significant aortic valve disease on noninvasive imaging. Similar case examples in assessing severity of conduit stenosis, arterial obstruction, or pulmonary pressures accentuate the role of catheter confirmation.
The ACHD population is often not “routine.” Operative repair in patients with ACHD is complex in itself, meriting due diligence to avoid unexpected operative issues. An anomalous coronary or dual left anterior descending (LAD) supply in a tetralogy of Fallot patient may be undetected and accidentally transected, or an opportunity to address an additional unrecognized defect might be missed. Echocardiographic gradients are often misleading in conduits where alignment with the flow is suboptimal despite best efforts, the continuity equation valve area calculations erroneous, and estimated shunt flows inaccurate even in the hands of expert echocardiographers. Unfortunately the same perils hold true for cross-sectional imaging—an iatrogenic fistula, ventricular aneurysm, peripheral pulmonary stenosis, aortopulmonary collateral may not be fully appreciated despite multiple imaging studies.
There is no doubt that noninvasive imaging has advanced substantially. This enhancement, coupled with generational advances in perioperative and operative management and intervention, has allowed us to push the horizon of what is possible. However, the pursuit of a consistent and confirmed set of data on which to base life-saving interventions that are potentially of great risk remains of prime significance. Especially when inconsistencies in data exist, catheterization is essential in decision making for surgery, intervention, or even the assessment of risk.
Catheterization remains the most accurate method to determine the pulmonary artery (PA) pressure and pulmonary vascular resistance. The time-honored practice of oximetry and shunt determination is a confirmatory piece of information, and the weight placed on it is reflected in our present guidelines for intervention in ACHD. There are a number of situations in which noninvasive imaging cannot provide the anatomic detail required for decision making. The recognition that imaging may not reliably assess the lumen of a PA or collateral vessel after stenting may lead to a further intervention that could improve a patient’s quality of life. Coronary angiography still provides the gold standard to assess coronary lesions and their suitability for revascularization. The addition of invasive physiology (fraction flow reserve [FFR] and invasive cardiopulmonary exercise testing [iCPET]) and additional imaging modalities (intravascular ultrasonography [IVUS] and intracardiac echocardiography [ICE]) can make the resolution of a clinical question regarding lesion severity a straightforward issue ( Fig. 10.1 ). Diagnostic catheterization, even in the present era, remains a critically important part of the diagnostic toolkit in ACHD.

Procedural Preparation
The quality and utility of the diagnostic information provided by an ACHD catheterization procedure are directly related to preprocedure preparation and the knowledge base of the operator. Before embarking on any complex case, it is crucial to have an intimate knowledge of the patient’s native and surgical anatomy. Clarification of the goals of the procedure with the referring ACHD specialists is often important to understand crucial issues that need to be resolved at the time of the procedure.
History and Imaging
A review of the patient’s clinic chart with specific attention not only to the native anatomy but also to the details of previous surgical and interventional repairs is paramount. A tattered 25-year-old surgical report may be a holy grail of information. A seasoned surgeon’s operative report may describe the native and surgical anatomy in great detail and provide insight into what was repaired, how, and why. The surgical report may be the only reliable source as to the size and type of implanted surgical valve or conduit. As any experienced ACHD physician will note, we play broken telephone too often when following patients over decades; all it takes is an errant word or typographic error to alter the substance of the anatomic problem. A close second in the hierarchy is a good-quality computed tomography (CT) scan or MRI by an experienced imager. Finally, review of previous hemodynamic and angiocardiographic evaluations helps to consolidate an understanding of potential procedural issues that may not otherwise be apparent. As an example, knowledge that an unusual catheter shape or technique was helpful in entering an anomalous vessel or chamber at a previous catheterization may facilitate the subsequent procedure significantly, limiting contrast and fluoroscopy. Vascular access is another example: If a patient is known to have had an occluded right iliac venous system as a child, there is not much hope that it has spontaneously recanalized as an adult, and thus alternative plans should be made.
More crucial than the newest technology and expensive equipment is the availability of imaging experts to conduct the study, interpret it, and caution us of any limitations. Collaboration among the imager and clinical, surgical, and interventional physicians allows the integration of knowledge and facilitates the delivery of excellent patient care. Little is gained from the ability to produce beautiful images that are interpreted in a way that is not meaningful to the clinician. It is as important for the radiologist to know the concerns of the surgeon and interventionalist, as is the reverse. Choosing the right modality to answer a particular set of questions is key, as is providing the imager with a clear articulation of the diagnostic question so that correct protocols are used to obtain the information required.
What Information is Essential?
The operator must have a thorough understanding of the anatomy and physiology of all congenital cardiac defects, associated abnormalities, therapeutic options for the defect under investigation, and information the surgeon/interventionalist will require if the patient is referred for treatment. Before starting it is critical for the ACHD interventionalist to know the following:
- •
What information is essential to establish the diagnosis or plan the treatment
- •
What information would be useful to obtain but is not critical
- •
What information is redundant and already available from other imaging studies
When thought and preparation have been given priority before the procedure, the catheterization can be more efficient in achieving the stated goals while minimizing radiation exposure, volume of contrast media, and procedural risk. The following are specific questions in procedural planning:
- 1.
Am I aware of the information that is crucial to complete the procedure? Example: Is a descending aortogram required to map an anomalous vessel?
- 2.
How will I gather the information required to establish the diagnosis; to define the anatomy, physiology, and presence of associated anomalies; and provide the surgeon and ACHD clinician with the information necessary?
- 3.
Will I need additional noninvasive testing either before or after the catheterization to better answer the diagnostic question?
- 4.
Is moderate sedation administered by the interventional cardiologist adequate or will deep sedation or general anesthesia be required, such as in adult patients with developmental delay?
- 5.
Are there comorbidities that might add to the risk of a complication, and, if so, what are the potential preventive measures? Examples: Contrast-induced nephropathy (CIN) risk from baseline renal dysfunction, latex allergy, or history of heparin-induced thrombocytopenia that will require removing heparin from flush solutions.
Not uncommonly, complex cases can become unintentionally long or, worse, be completed without obtaining a key piece of information. The most common essential information required for management decisions concerns the pulmonary vasculature: the PA pressure and resistance, the reactivity of the pulmonary vasculature, and shunt calculations. If access to the PA is difficult, this may prolong the procedure, but time invested here may be far more valuable than recapturing other data already clear from other diagnostic testing. This is never more relevant than when surgical shunts or aortopulmonary collaterals contribute flow to the pulmonary circulation. Similarly the temptation may occur to defer coronary angiography after a difficult procedure in a young adult at low risk for atherosclerosis, thus missing the opportunity to detect a relevant congenital anomaly of the coronary circulation that may directly impact future decisions. As our adult patients age, they may also develop acquired circulatory conditions (eg, coronary artery disease) that may complicate their course. Detection of atherosclerotic coronary disease, coronary compression, elongation, or torsion in patients with symptoms that may be multifactorial is always of importance.
In cases in which a therapeutic intervention is preplanned and focused, such as a coarctation stent, the diagnostic component and intervention may be performed in the same setting. In complex ACHD patients, one must weigh the risks and benefits of ad hoc interventions. Immediate interventions after diagnostic catheterization improve efficiency, minimize the risk of repeat access, and reduce the number of total procedures; however, they are at the cost of increased contrast, fluoroscopy exposure, and on occasion inadequate discourse with the patient. In ACHD patients, optimal treatment decisions should involve multidisciplinary discussions to help to dictate care.
Which Catheters to Use and The Sequence of Events?
The prepared operator will go into the cardiac catheterization laboratory with a clear idea of which catheters are likely to be most helpful and the sequence to obtain the required information. For example, it may be useful to begin the right-sided heart catheterization with a steerable catheter, such as a Goodale-Lubin catheter (Medtronic, Minneapolis, Minnesota), to sample oxygen saturation, probe for atrial septal defects (ASDs) and anomalous venous drainage, and then change to a balloon-tipped catheter to cannulate the PA through a difficult right ventricular outflow tract (RVOT). A modified Judkins right coronary artery catheter with side holes near the tip is also of great value, easing pressure measurements and oximetric sampling in tight spaces. Preplanning of when a catheter with radiopaque markers is needed for measurements or when a multitrack catheter will allow for hemodynamics and high-pressure injections without losing wire access can save considerable time.
When possible, all hemodynamic measurements and oximetry samples should be performed close together in a steady state and on room air. Venous pressures and saturations should be obtained in a structured predictable order so that all the right- and left-sided hemodynamic information is obtained before administering contrast. Having a routine approach allows the nursing staff to anticipate, prepare, record, and chart the procedure accurately.
One should make a checklist at the beginning of the procedure, outlining the hemodynamic information to be obtained, expected chamber/vascular angiography, catheters that will be necessary, and the procedural sequence to be followed. A team huddle prior to the procedure is invaluable to explain the patient’s reason for catheterization, clinical concerns, and going through the expected sequence of events. “Time outs” and checklists, shown by the World Health Organization to reduce operative error, and adapted from the operating room, are now a standard part of the catheterization laboratory ( Fig. 10.2 ). These important protocols have been shown to improve safety and efficiency and must be adhered to.

What Can Go Wrong?
Common problems with cardiac catheterization studies in adult patients with congenital cardiac disease relate to the following:
- •
Patient pain and anxiety and, as a result, oversedation
- •
Prolonged catheterization time and contrast administration
- •
Inadequate, missing, or nondiagnostic information
- •
Catheter complications
Every case starts with the patient’s comfort and best interest as paramount. ACHD patients cover the age spectrum, and, although they may be well versed with medical procedures, procedural anxiety and pain from access must always be adequately addressed. Caring for a patient who has had multiple procedures requires not only the management of the present procedure but also the sequelae and inadequacies of prior procedures. As per the standard of care in children, their prior catheterization laboratory experience may have included general anesthesia as opposed to conscious sedation used in most adult cases. Considerations for adequate sedation, intravenous anesthesia, and local anesthesia are important. In addition, a kind and calming distraction in the form of reassurance, and the caring touch or handholding of an unscrubbed team member often provides comfort and assists the process. The physiologic response to pain can alter steady state and dramatically affect the hemodynamic conclusions. A vagal reaction from access or pain from catheter manipulation at the access site can alter the steady state and jeopardize the integrity of the information obtained. This is usually amplified in ACHD patients who have had many procedures with dense scar tissue at the site of the puncture. An appropriate amount of sedation for most adults is mandatory. Caution should be exercised to “start low and go slow.” The oversedated patient may develop airway obstruction, hypercarbia, systemic hypoxemia, and elevated pulmonary pressures.
Most ACHD procedures can be expected to take substantially more time to complete than the usual right-sided and left-sided heart procedures in patients with coronary or valvular heart disease, particularly if an unexpected finding arises during the procedure. One way to ascertain that the procedures are kept short is to ensure that the invasive test is performed after all relevant noninvasive tests to avoid repeated documentation of known facts. In general, longer procedural times are associated with increased contrast and fluoroscopy. With the aging of the ACHD population and the accumulation of comorbidities, it is of utmost importance to minimize the risk of CIN from the administration of large volumes of contrast agent to document anatomy that is already well appreciated through other cross-sectional imaging modalities ( Table 10.1 ). CIN risk is especially poignant in patients with bidirectional cavopulmonary anastomosis or Fontan surgery. Prehydration, use of low osmolar contrast agents, and cessation of nephrotoxic medications are all strategies that can help prevent CIN development. There is also a growing recognition to the lifetime stochastic risks of radiation exposure, especially to an ACHD population that may require multiple radiologic tests throughout their lifespan. Decreasing fluoroscopic exposure during the case using best practice radiographic techniques is important; the radiation dose should be as low as reasonably achievable to achieve diagnostic images.
Intrinsic patient characteristics | Chronic kidney disease or prior renal dysfunction |
Congestive heart failure or LVEF <35% | |
Diabetes | |
Age >75 years | |
Transplanted kidney | |
Potentially modifiable patient risk factors | Volume status or anemia |
Concomitant medications with potential nephrotoxicity | |
Hypotension or shock | |
Procedural/imaging characteristics | Total volume of contrast |
Multiple contrast injections within short period of time | |
High-osmolar contrast formulations |
Adult Congenital Heart Disease Catheterization Laboratory
Catheterization Laboratory Infrastructure
ACHD and structural procedures have different requirements than adult coronary or peripheral vascular interventions. Modern designs of congenital and structural catheterization laboratories are larger in size to accommodate additional personnel and imaging modalities. Anesthesiologists and echocardiographers are frequently present in complex interventions, especially for intraprocedural transesophageal echocardiography (TEE) guidance. The structural catheterization laboratory must include (1) fluoroscopic digital flat panel monitors to allow for x-ray visualization and hemodynamics from both sides of the table, (2) adequate radiation protection for staff, (3) monitor integration for real-time echocardiography during the case, and (4) biplane fluoroscopic heads with larger size than coronary imaging to allow for adequate coverage for systemic angiography (eg, pulmonary angiography). (5) The ideal ACHD catheterization laboratory should also be a hybrid suite with operating room–style ventilation and technical standards suitable for cardiopulmonary bypass, mechanical ventilation, and cardiovascular surgery.
ACHD interventions often rely on real-time TEE or ICE and the interpretation of CT/MRI cardiovascular imaging to guide treatment strategy. Catheterization laboratory systems should be able to display prior imaging studies tableside simultaneously with live fluoroscopy and ultrasound imaging. Technology harmonizing TEE ultrasound with fluoroscopy allows localization of precise structures in real time on fluoroscopy. Modern catheterization laboratory systems can also use superimposed 3D CT- or MRI-guided roadmaps to direct complex interventions. The ability to perform real-time 3D rotational angiography is also of increasing importance.
Basic catheterization laboratory Equipment
A vast array of wires, catheters, stents, embolization devices, stents, valves, and retrieval devices are needed to address congenital heart lesions in a variety of sizes and configurations. There are few things as disappointing to the operator or patient as arriving at a particular point during a procedure and a particular piece of equipment required to complete the procedure is unavailable. A well-planned procedure will include consideration of the inventory required. However, an abundance of equipment will necessitate that some equipment that will need to be discarded because of date of expiration; this should be looked on as a necessary evil. Careful inventory planning and management is absolutely essential to reduce waste.
Sheaths
A selection of short and long sheaths from 4 to 25 French (Fr) is required. Large-caliber stents needed for coarctation or pulmonary outflow tract treatment can necessitate sheath size up to 16 Fr (eg, for covered stent implantation using a balloon delivery system). Most available transcatheter valves come with their own proprietary sheaths ranging between 14 and 24 Fr. Similarly, companies selling occlusion devices or plugs often sell corresponding delivery sheaths (eg, TorqVue delivery systems for Amplatzer devices [St. Jude Medical, Saint Paul, Minnesota]). Although the size match with these proprietary sheaths is guaranteed, alternate sheaths with adequate inner lumen accommodation can also be used.
There are various Mullins-type sheaths that should be purchased with radiopaque tip markers ( Fig. 10.3 ). In addition, some operators use kink-resistant long sheaths. Such sheaths are advantageous when there is peripheral tortuosity, when large loops in the right atrium are required or the RVOT has an acute angulation, or when delivering devices to the pulmonary arteries. Finally, there is a utility for steerable sheaths, such as Agilis NxT Steerable Introducer (St. Jude Medical), for improved stability and better catheter access and support, such as in interventions involving the pulmonary veins or mitral valve ( Fig. 10.4 ).


Guide Wires
Guide wire sizes range from 0.014 to 0.038 inches in diameter and from 50 to 260 cm in length. Their design includes wire cores with varying degrees of tensile strength and outer coatings that can differ in hydrophilic and lubricious characteristics. The distal 1 to 5 cm end of the wire is often distinct in design and maneuverability from its remaining length; this end often determines a wire’s utility ( Fig. 10.5 ). For example, wires can be labeled as super floppy, ordinary, super stiff, hydrophilic, and glide (eg, Terumo, Sommerset, New Jersey). Spring coil design wires with hydrophilic coating on the distal end are invaluable to engage tortuous vessels while allowing adequate support for catheter exchange (eg, Wholey, Coviden, Plymouth, Minnesota, and Magic Torque, Boston Scientific, Natick, Massachusetts).

The Amplatz super-stiff and ultra-stiff guide wires (Cook Medical, Bloomington, Indiana) (0.025 to 0.038 inch) are the mainstay for almost every case in stabilizing balloons across high-flow lesions and during stent implantation or valvuloplasty. The Meier Backup wire (Boston Scientific) and Lunderquist extra stiff wire (Cook Medical) have been invaluable for transcatheter pulmonary and aortic valve implantation when tortuosity and calcification is a problem. In addition, different 0.014 coronary wires are important to have on hand to engage coronary fistulas and small tortuous arterovenous malformations.
Catheters
A variety of catheters are required; basic configurations such as Amplatz, multipurpose, Goodale-Lubin, Gensini, pigtail, Cobra, Vertebral, and Judkins coronary catheters are essential ( Fig. 10.6 ). These catheters will need to be stocked in a variety of sizes and configurations. The presence of radio-opaque markers on available catheters can be important for calibration and confirmation of any angiographic measurements. It is helpful to stock a series of 4- to 5-Fr hydrophilic catheters in lengths of 100 and 120 cm. These catheters will track through almost any tortuous bend to a destination often unreachable by standard catheters. They permit pressure monitoring from these locations, as well as exchange for stiffer wires to deliver sheaths required for therapy. The Multi-Track angiographic catheter (Braun, Bethlehem, Pennsylvania) allows pressure measurements and high-pressure injections while still maintaining distal wire access ( Fig. 10.7 ). These catheters are invaluable, particularly for RVOT/main PA injections in the setting of pulmonary insufficiency. Use of a pigtail or other side-hole catheter in this location often results in recoil and loss of position with high-pressure injections. They are available with a set of distal marker bands for size calibration.


Swan Ganz and PA catheters are a mainstay of all right heart catheterization—but especially important to use when performing interventions in the RVOT or PAs to avoid damaging the tricuspid valve chordae when later exchanging to large-bore sheaths. Wedge catheters can allow for selective PA/wedge angiography to visualize levophase pulmonary venous return and to confirm the wedge position for pressure measurement. Long microcatheters with lumens that accept 0.018-inch wires should be available for coil delivery. Tapered and nontapered catheters, guiding catheters, and balloon wedge (end-hole) and angiographic (side-hole) catheters, such as the Berman catheters (Arrow Inc, Reading, Pennsylvnia), are the foundation of any interventional laboratory (see Fig. 10.7 ). A balloon-tipped Berman catheter can be useful to float into distal PAs while its side holes allow for high-pressure angiography without exchange; the limitation of these catheters is the inability to measure the pulmonary capillary wedge pressure.
A comprehensive stock of catheters is an asset. Each operator will choose an appropriate selection and become familiar with their use. The more complex the case mix, the greater the variety of catheters that will be needed in the inventory.
Balloons
ACHD interventions require a large variety of balloon sizes and types that run the gamut from designs for coronary interventions, peripheral arterial procedures, and valvuloplasty. Given the variability of procedures and patient population, equipment will range in size, length, design, material, and limitations. Many balloons adapted for ACHD interventions may not have been initially produced for intracardiac or pulmonary applications but rather for peripheral angioplasty ( Fig. 10.8 ). Low-pressure balloons (eg, Tyshak I & II and Z-Med I & II from NuMed Inc, Cornwall, Ontario, Canada) are available in a range of sizes (4 to 30 mm in diameter with 4- to 13-Fr shafts). They are especially advantageous because of their rapid deflation rates, which limit the time an outflow tract is occluded during an inflation cycle. High-pressure balloons also come in a range of sizes and lengths from a number of manufacturers (eg, Mullins-X from NuMed or Atlas from Bard, Murray Hill, New Jersey). Other noncompliant balloons (Atlas Gold and Vida balloons from Bard) have advantages of shorter shoulders on inflation and minimize vessel straightening. The Conquest balloon by Bard is an ultra-noncompliant balloon that prevents any balloon overexpansion from predicted diameters even at very high pressures.

Most balloons can be used as platforms for stent delivery. The BIB (balloon in balloon) from NuMed is very popular for controlled expansion and is especially useful for stent delivery (sizes 8 to 24 mm in diameter). A large range of balloon sizes, as well as an adequate selection of high-pressure balloons, is essential ( Fig. 10.9 ).

Transseptal Equipment
Transseptal needles, using the Mullins transseptal technique, will occasionally be required to enter the left side of the heart, cross through a lateral tunnel Fontan, enter the pulmonary venous baffle in a Mustard/Senning patient, or perforate an atretic vascular structure. For the adult, generally two lengths of transseptal needle can be stocked ( Fig. 10.10 ), a standard (71 cm) and a long (89 cm) length needle if an Agilis sheath is required to reach the septum in massive right atrial dilation. It is usually wise to begin with the standard small transseptal curve and trade upward in case of failure. In addition, the hub of the dilator should be such that when the needle and hub are engaged, only 2 or 3 mm of the needle is exposed from the tip. A useful trick for difficult transseptal punctures is to reintroduce the obturator shipped with these devices (usually removed and discarded when the needle is flushed prior to introduction). There are different curves to modern day transseptal sheaths, accounting for where on atrial septum it would be most advantageous to cross (eg, more inferior for mitral balloon valvuloplasty and more superior for MitraClip interventions). Swartz SL Series of 8- and 8.5-Fr sheaths (St. Jude Medical) come in a 63- or 81-cm length with a primary curve of 50 degrees and variable secondary curves (SL0: 0 degrees; SL1: 45 degrees; SL2: 90 degrees; SL3: 135 degrees; SL4 180 degrees). When engaging the pulmonary veins, SL0 or SL1 may be adequate, whereas for mitral valve interventions, SL2 or SL3 may provide a more posterior orientation. For medial-oriented mitral perivalvular leaks, SL4 curve may be optimal.

Surgical material, such as that of extracardiac Fontan tunnels, can occasionally be challenging to cross with transseptal needles; in these cases, radiofrequency ablation (RFA) needles have proven useful (see Fig. 10.10 ). As long as the anatomy is well understood and there is minimal calcification, RFA can offer variable penetration across most fabrics (eg, Dacron, polytetrafluoroethylene [PTFE], or Gore-Tex), although there are limitations to this technique.
Embolization Equipment
In patients with congenital heart disorders, vascular embolization is achieved by either coils or adaptation of septal and vascular occluder devices, such as ductal, atrial, and patent foramen ovale (PFO) defect occluders.
Coils
Historically, the Gianturco free release coil (Cook Medical) has been the primary device for peripheral embolization; however, controlled release coils (in which coil release is dependent on an active maneuver from the operator) offer a safer implant, especially in higher-flow lesions or areas where precise coil implantation is critical. A large variety of coil sizes, lengths, and shapes are available from various suppliers. A selection of guide catheters and microcatheters should be available for coil delivery ( Fig. 10.11 ). Controlled release coils can be retrieved and repositioned before release. Of note, controlled release coils that use electrolytic detachment should be avoided in the coronary arteries because they can result in chest pain with ECG changes. Coils that use a mechanical release mechanism are generally safe in all situations. Such coils are atraumatic to the vasculature, with low radial friction in the delivery catheter lumen allowing for ease in delivery even in tortuous segments. For an effective occlusion, a dense mass of thick, long coils is optimal. Modern-day coils, such as MReye Embolization Coil (Cook Medical), are safe for future MRI scanning, which is important in our ACHD population.

We prefer platinum coils that allow for future MRIs without the artifact of stainless steel, which is especially important in young patients. We primarily use controlled release coils, given their advantages of repositioning. In situations of high flow in which a large number of coils would be required to achieve embolization, a plug may be the preferred strategy.
Septal Defect Occluder Devices
There are several devices clinically available for closure of both secundum ASDs and muscular ventricular septal defects (VSDs). In North America, there are essentially two design platforms of ASD occluders, including self-centering plug, double-disc design made of a nitinol wire mesh (Amplatzer Septal Occluder [ASO] and Amplatzer Multifenestrated Septal Occluder, St. Jude Medical, or the Figulla Flex II Occlutech, Helsingborg, Sweden; Figulla is not US Food and Drug Administration [FDA] approved), and a five-wire supported frame covered with a thin PTFE patch-like material (Gore Septal Occluder [GSO], Gore and Associates. Flagstaff, Arizona). The ASO is available with waist diameters of 4 to 40 mm with right atria (RA) disc diameter 8 to 10 mm and left atrium (LA) disc diameter 12 to 16 mm larger than the waist. The Figulla Flex II (not available in the United States) ranges in waist size between 4 and 40 mm for defects ranging up to 40 mm in size. The GSO comes in four sizes (15 mm, 20 mm, 25 mm, and 30 mm). The GSO is limited to defects less than 18 mm, whereas the ASO and Figulla Flex II can close defects up to a maximum of 38 and 40 mm, respectively ( Fig. 10.12 ). The issue of device erosion is unique to the nitinol wire mesh devices (although no reports of erosion with the Figulla device), and this has prompted some operators to use non–self-centering devices. Gore has a new device in development with a similar design and materials the same as the GSO that will allow closure of defects up to 33 mm.

The most commonly used implant approved for muscular VSDs is the Amplatzer Muscular VSD Occluder (St. Jude Medical). It is also a nitinol-based, self-expandable, double-disc implant with a 7-mm-long plug with diameters from 4 to 18 mm (waist diameter). The retaining disks are symmetric with an 8-mm larger diameter than the device waist. The technique for VSD closure is fairly standard with the creation of a venoarterial rail to guide the delivery sheath across the defect with device insertion generally from the venous approach. Apical defects may be better approached from the neck, whereas more basal defects from the femoral vein. These devices have been frequently used off-label for other indications, including closure of ASDs, perimembranous VSDs, patent ductus arteriosus (PDA), or fistulas. There is also a postmyocardial infarction Amplatzer VSD Occluder (St. Jude Medical) that has a waist measuring 10 mm (as opposed to the 7-mm waist on the muscular VSD occluder device) to better conform to the adult septum. It comes in sizes 16 through 24 mm ( Fig. 10.13 ). Although the initial iteration of a perimembranous VSD-specific device from St. Jude Medical was taken off the market secondary to high incidence of complete heart block, the first worldwide experience with the redesigned Amplatzer Membranous VSD Occluder 2 (St. Jude Medical) may show promise but requires further disciplined and systematic investigation.

Duct Occluders and Vascular Plugs
Other than the occasional use of coils, the majority of the North American market for PDA closure is dominated by the Amplatzer Duct Occluder (ADO) and Duct Occluder II (St. Jude Medical) ( Fig. 10.14 ). These nitinol-based devices can be easily removed or readjusted prior to detachment from delivery cable, which is an appealing feature. The ADO device is asymmetric with a larger diameter at the aortic side (sizes 5 to 16 mm) than pulmonary end (sizes 4 to 14 mm) with a larger retention skirt that necessitates deliver from a PA approach. Of note, the larger 14- to 12-mm and 16- to 14-mm ADO devices are not available in the United States. The ADO II device has two symmetrical retention disks, allowing for delivery from either arterial or venous approach. It is smaller in caliber, allowing for generally smaller sheath/catheter size for an equivalently sized device (waist diameters range from 3 to 6 mm, with two lengths at each diameter of 4.25 or 6.25 mm). ADO II is more commonly used in the neonatal and pediatric population.

There are a variety of differently shaped vascular plugs that can be very useful in closing venovenous or aortopulmonary collaterals and pulmonary AV malformations. Amplatzer vascular plugs 1 through 4 (St. Jude Medical) represent four different space configurations with a variety of different sizes and lengths ( Fig. 10.15 ). These devices also have an important role in percutaneous treatment of perivalvular leaks.

Endovascular Stents
Operators should be familiar with different types of endovascular stents, noting their individual advantages and limitations. In the adult setting, stocking a range of sizes and lengths can be rationalized, particularly for use in aortic coarctation, baffle stenosis, and in the pulmonary vasculature. Stents are often categorized into four sizes based on maximal diameter: small (2 to 5 mm), medium (5 to 10 mm), large (10 to 18 mm), and extra large (up to 25 mm or larger).
For coarctation and PA stenting, there are a number of balloon-expandable stents, including but not limited to the cobalt chromium–based Andrastent (Andramed, Reutlingen, Germany), platinum iridium–based Cheatham-Platinum (CP) stent (NuMed), and tantalum-based Strecker (Boston Scientific, Natick, Massachusetts). Stainless steel has been the traditional material used in stents with a great track record of radial strength; closed-cell designs include Palmaz XL series and Genesis stents (Cordis, Hialeah, Florida). Open-cell iterations allow for better access to jailed side branches, including Mega and Maxi LD series (Coviden, Plymouth, Minnesota) ( Table 10.2 ).
Stent Class | Stent Type | Manufacturer | Material | Cell-Type | Available Diameters | Additional Characteristics |
---|---|---|---|---|---|---|
Bare metal ( self-expanding ) | Wallstent | Boston Scientific (Natick) | Elgiloy | Closed | Medium-to-large 5-24 mm (6-10 Fr) | All these self-expanding stents have FDA indications for vascular disease. |
ProtégéGPS | Coviden (Plymouth) | Nitinol | Closed | Medium 6-14 mm (6 Fr) | ||
SMART | Cordis (Hialeah) | Nitinol | Closed | Medium 6-14 mm (6-7 Fr) | ||
Zilver vascular stent | Cook (Indiana) | Nitinol | Closed | Medium 6-10 mm (5-7 Fr) | ||
Bare metal ( balloon-expandable ) | Andrastent L, XL, and XXL | Andramed, (Reutlingen, Germany) | Cobalt chromium | Open & Closed | Medium-to-Large 6-32 mm (7-9 Fr) | Unmounted |
Cheatham-Platinum (CP) | NuMed (Hopkinton) | Platinum-iridium | Closed | Large 12-24 mm (10-12 Fr) | Welded-tube design. Available Premounted and unmounted. | |
IntraStent Intrastent Mega | eV3 Inc., (Plymouth) | Stainless Steel | Open | Medium 9-12 mm (9-11 Fr) | High radial strength—used for stenting RVOT and coarctation | |
Formula 418 | Cook Europe (Bjaeverskov, Denmark) | Stainless Steel | Closed | Small-to-medium 3-8 mm | Premounted—minimal shortening with balloon expansion. | |
Palmaz Blue | Cordis(Hialeah) | Cobalt chromium | Closed | Small-to-medium 4-7 mm (6-7 Fr) | Premounted; Low profile and flexible. | |
Palmaz Large & XL series | Cordis(Hialeah) | Stainless steel | Closed | Large 8-25 mm (10 Fr) | Unmounted | |
Palmaz Genesis | Cordis(Hialeah) | Stainless steel | Closed | Medium 4-10 mm (8-9 Fr) | Low profile; Premounted | |
Valeo | Bard(New Providence) | Stainless steal | Open | Medium 6-10 mm (6-7 Fr) | Premounted | |
Covered ( balloon-expandable ) | Advanta V12- or iCAST | Atrium (Hudson) | stainless steel/PTFE | Covered | Large 16-22 mm (9-11 Fr) | Lower profile but less radial strength than Covered CP. Unmounted |
Covered-CP | NuMed (Hopkinton) | Platinum-iridiumPTFE | Covered | Large 12-24 mm (12-14 Fr) | Unmounted | |
Covered ( self-expanding ) | Viabahn endoprosthesis | Gore (Flagstaff) | Nitinol/PTFE | Covered | Medium 5-13 mm (6-12 Fr) | Flexible—good in tortuous vessels |
Valiant Captiva Endograft | Medtronic (Santa Rosa) | Nitinol/Polyester | Covered | Large 22-46 mm (22-25 Fr) | Approved for thoracic Aneurysm | |
TAG Endograft | Gore (Flagstaff) | Nitinol/PTFE | Covered | Large 26-45 mm (20-22 Fr) | Approved for thoracic Aneurysm | |
Zenith TX2 Endograft | Cook (Bloomington) | Nitinol/Dacron | Covered | Large 28-42 mm (20-22 Fr) | Approved for thoracic Aneurysm |
The CP stent is uniquely manufactured from platinum and iridium–based wire that is bent and gold-welded to a cylindrical meshwork called a welded tube design. This platform leads to increased flexibility and delivery at the cost of radial strength. Unlike other implants, this stent has rounded leading and trailing edges that reduce the risk of balloon rupture during inflation and vessel trauma. Importantly, the implant is compatible with MRI. Thus the CP stent is one of the more commonly used stents in congenital heart disease.
There is a role for self-expanding stents such as the stainless steel Wallstent (Boston Scientific, Natick, Massachusetts), for example in venous stenosis (inferior vena cava [IVC]/superior vena cava [SVC]) where balloon expansion may be too aggressive for thinner walled veins. Covered stents (both balloon expandable and self-expanding) have a role in the primary treatment of coarctation of the aorta or in baffle stenosis with concomitant leak. They have an important role as standby or bailout in situations of vascular bleeding/perforation. The covered CP stent (NuMed) is used worldwide for primary treatment in pulmonary outflow tract or coarctation; it remains investigational in the United States, awaiting publication of Coarctation of Aorta Stent Trial-II (COAST II) registry data ( Fig. 10.16 ).

Transcatheter Stent Valves
Transcatheter valves have revolutionized the field of congenital and structural cardiac interventions. Since Dr. Philipp Bonhoeffer performed the first-in-man percutaneous pulmonary valve implantation (PPVI) in 2000, PPVI has become an alternative to surgical pulmonary valve replacement (PVR) in patients with dysfunctional bioprosthetic pulmonary valves, homografts, or conduits with intermediate to long-term follow-up. The two valvular systems with greatest worldwide experience for PPVI include the Melody valve and Ensemble delivery system (Medtronic) and the Edwards Sapien Pulmonic Transcatheter valve (Edwards Life Sciences, Irvine, California) and the Edwards RetroFlex III transfemoral delivery system. Newer-generation valves from Edwards (Sapien XT and Sapien 3) and newer delivery systems (Novoflex and Commander) have also been applied in pulmonic position ( Fig. 10.17 ).

The Melody valve is harvested from a bovine jugular vein that is sutured into a 28-mm-long platinum iridium stent frame. The valve is preserved in a proprietary mixture of glutaraldehyde and alcohol and must be manually crimped onto a 22-Fr BIB (NuMed) balloon with expandable diameters of 18 to 24 mm. The Ensemble delivery system allows the valve to remain sheathed until the correct position at the pulmonary annulus is achieved.
The original Sapien valve consisted of bovine pericardial leaflets with a proprietary Thermafix treatment to prevent calcification sewn into a balloon-expandable stainless steel platform. The Sapien XT and Sapien 3 use a cobalt chromium alloy in place of steel, and the Sapien 3 adds a polyethylene terephthalate outer skirt to minimize perivalvular leak. The Sapien 3 is available in 23, 26, and 29 mm sizes and, given its lower profile with improved stent design, is expected to supplant prior valve generations. The Commander delivery system requires an Edwards Esheath that is 14 Fr for smaller valve sizes and 16 Fr for 29-mm valve (that expand to 18- and 20-Fr outer diameter, allowing delivery in vasculature ≥5.5 mm and ≥6 mm, respectively).
In the pulmonary (or tricuspid valve-in-valve) position, access is usually performed through the femoral or internal jugular vein. In the aortic position with Sapien, access options include transfemoral or occasionally transapical, or transaortic. In those with inadequately sized iliac arteries or for mitral valve-in-valve deployment, the transapical route involves a small left thoracotomy and valve delivery through the left ventricle (LV) apex while the heart continues to beat. A subclavian approach, usually using a short graft, involves a small, high left thoracotomy exposing the subclavian artery to allow transcatheter aortic valve replacement (TAVR) delivery. Direct aortic or transaortic access involves a ministernotomy and access from the upper ascending aorta while maintaining a beating heart. There are also reports of successfully using transcaval access, creating a temporary AV-fistula/connection to the abdominal aorta for TAVR.
Although it is not deployed in a pulmonary position, Medtronic CoreValve Evolut R and EnVeo Delivery system uses a self-expanding technology for delivery, as opposed to balloon expansion (see Fig. 10.17 ). The valve (available in 23-, 26-, and 29-mm diameter) consists of a goblet-shaped, laser-cut nitinol tube 50 mm in length, to which a porcine pericardial valve has been sewn. The lower portion of the stent has high radial strength to exert force on the native annulus. The stent is convexo-concave to avoid covering the coronary arteries. The device has gone through several iterations and presently requires a relatively small, 14-Fr InLine sheath. The implant procedure is slow and deliberate and does not require rapid pacing. Unique to the Evolut R generation is that the valve is fully recapturable and repositionable, which is a significant technical advantage. For the very large aortic annulus, the CoreValve device is available with a 31-mm valve.
Beyond considerations of annulus size, there are several differences between these valve platforms that should be considered. It is optimal for an ACHD catheterization laboratory to have access to several valve choices if performing PPVI. In addition to the pulmonary valve, there are other congenital nonpulmonic valve abnormalities in which transcatheter techniques have been used offlabel. Undoubtedly, the interventional armamentarium to treat congenital valve disease in adults is expected to grow.
Hemodynamic Assessment
Pressure Measurements
Pressure measurement in all the cardiac chambers and vascular beds is the most fundamental constant of any hemodynamic evaluation. Accurate pressure measurement requires an end-hole, large-lumen catheter and tubing with a continuous column of saline connected to a high-fidelity pressure transducer. Side-hole catheters can work in large chambers (eg, left ventricle) but can be problematic in smaller vascular spaces (eg, distal PA). To ensure accurate measurements, there are a few basic rules that must be adhered to: (1) catheter tip should be free from vessel wall and catheter should be kept straight without kinks; (2) fluid column in catheter and tubing should be continuous without microbubbles, air, contrast, or blood; and (3) transducer must be adequately flushed, zeroed, and standardized at right atrial level on regular basis to account for transducer drift.
When measuring pressures, catheter whip and extreme respirophasic variation can make interpretation challenging. Every attempt should be made to achieve a stable catheter position or dampen this artifact by minimizing catheter movement or occasionally accepting more viscous blood into the catheter column. By convention, pressures are measured at end-expiration. Normal intracardiac and pulmonary pressures, waveform pictorals, and waveform components are displayed in Table 10.3 .
Specific points should be considered when measuring hemodynamics in ACHD patients. Small gradients in venous pressures (approximately 1 mm Hg) can be hemodynamically significant (eg, in Fontan physiology). There are also inherent limitations in detecting 1 mm Hg differences in patients who are alive and breathing spontaneously. Similarly, careful and precise pressure measurements should be performed across venous baffles, PA stenosis, and each aortic segment when assessing for coarctation. Pressure gradients across aortic coarctation (or any region of interest) are best obtained with two separate catheters (or sheath and smaller caliber catheter) positioned proximal and distal to a stenosis to avoid issues of pressure recovery, pressure amplification, and collateral blood flow. In general, dual-lumen catheters (eg, Langston catheter) or two separate catheters are preferable to either pullback measurements (or in case of aortic valve using femoral artery as aortic proxy) for optimal accuracy.
Saturations
In the decision-making process for a patient with a congenital heart lesion, oxygen saturation data are of great import. Arterial desaturation may reflect a right-to-left shunt, abnormal diffusion barrier across alveolar-arterial interface, a ventilation-perfusion mismatch, or hypoventilation. It is important to recognize hypoxemia and hypoventilation secondary to oversedation; a climbing CO 2 in a sedated patient may have a profound impact on pulmonary pressures, as well as the oximetric evaluation. Caution is suggested in the sedation of the anxious, low body weight, and in the failing single ventricle system—the mantra “start low and go slow” should be adhered to. In general, systemic venous saturation of less than 50% indicates low cardiac output, whereas high systemic venous saturation (eg, >85%) indicates either a left-to-right shunt or a high-output state.
Saturations should be obtained from each cardiac chamber and vascular space, typically simultaneous with pressure measurements. Depending on particular anatomy, we will obtain saturations at high and low SVC, IVC, RA, right ventricle (RV), main PA, distal right and left PA, pulmonary veins from right and left lung, LV, and aorta. Occasionally, additional saturations will be needed to clarify anatomic connections and physiologic significance (ie, Fontan tunnel, coronary sinus, anomalous venous connections, peripheral atriovenous malformations [AVMs]). Proper technique is crucial to waste stagnant blood in the catheter and avoid entrapment of air into the samples. Of note, in patients with chronic hypoxemia and Hb levels of greater than 22 g/dL, older rapid co-oximetry monitors may give inaccurate results. Modern instruments are generally reliable until Hb of greater than 25 mg/dL, although it is worthwhile to confirm these numbers with the central laboratory at each facility. In these patients, formal blood gases will be necessary to obtain saturation data. Blood gas machines typically measure PO 2 and calculate saturation that is less desirable.
All flow-based calculations in the laboratory, including Fick cardiac output, shunt quantification, and resistance, depend on the accurate determination of oxygen saturation. There are certain assumptions and potential errors when obtaining oxygen saturation data that must be considered, including the following:
- 1.
All measurements are made during a steady state. In other words, there are no changes in blood flow, respiratory rate, heart rate, or level of consciousness. The room should be quiet without distractions, and work should be performed with measured haste.
- 2.
Two or more samples are obtained from at least three sites in rapid succession, which, in the patient with complex congenital heart lesions, can be difficult to achieve.
Determination of what to use for “mixed venous saturation” can be important in making appropriate calculations of Fick cardiac output or shunt quantification. There is not a single, uniform source for mixed venous blood because the mixed venous blood sample has three variable sources (ie, the IVC, coronary sinus, and SVC), with each caval vein having multiple sources of blood with different saturations.
The SVC oxygen saturation may vary by 10% because it receives blood from the jugular, subclavian, and azygous systems, each with very different saturations and flows (the subclavian and azygous veins have higher saturations than the jugular vein). The IVC also has variable oxygen saturations because the components that make up its flow may vary by 10% to 20%. For example, more saturated blood originates from the renal veins, whereas less saturated blood comes from gastrocolic and hepatic sources. The mixed sample from the IVC is generally 5% to 10% greater than from the SVC. Coronary sinus blood also contributes to the total pool of mixed systemic return. Despite making up only 5% to 7% of total venous return, the very low saturation in this sample (25% to 45%) rarely impacts the total mixed saturation.
Because there are multiple contributions to the so-called mixed venous sample, there is no practical way to account for the variations in flow. Not even a sample from the right atrium can completely adjust for streaming. In the absence of a shunt lesion, a sample downstream from the right atrium, such as from the main PA, can provide a thoroughly mixed sample. In addition, it has been noted that the SVC blood saturation is very close to that in the main PA and can be representative of the mixed venous sample unless the patient has a low cardiac output state. Some investigators use a weighted average of SVC and IVC blood as a calculated mixed venous sample. In the presence of a downstream shunt lesion, several samples, obtained in rapid sequence and found to be near or equal in value, should be used. Although some use the formula Mixed venous saturation = (3 × SVC + IVC) / 4 , this is predominantly derived from pediatrics. We commonly use the equation (SVC + IVC) / 2 in adults.
Similarly, the mixed pulmonary venous saturation is a combination of all the pulmonary veins, each reflecting different ventilation to perfusion ratios. As such, a pulmonary vein sample may be 50% to 100% disparate from the true mixed pulmonary venous sample. In the absence of a right-to-left shunt, a downstream sample is preferable (left ventricle or aorta), rather than using a single pulmonary vein saturation. A pulmonary venous sample can help determine the cause of arterial desaturation, distinguishing intrinsic pulmonary disease fro intracardiac shunt.
Hemodynamic Calculations: Cardiac Output and Vascular Resistance
Two standard methods for calculating cardiac output in the catheterization laboratory are the Fick and thermodilution (TD) methods ( Box 10.1 ). The Fick method measures cardiac output by using the difference in O 2 saturation across a vascular bed, using arterial saturation and the chosen mixed venous saturation. The Fick method is especially accurate in low-flow states with high AV-saturation difference or in atrial fibrillation and is independent of tricuspid regurgitation. Disadvantages with Fick calculations include: (1) using estimated oxygen consumption may result in up to 30% error, depending on individual patient factors; (2) co-oximetry saturations are affected by air bubbles, contrast, methemoglobinemia, or carboxyglobinemia; (3) assumption of steady state during measurements; and (d) calculations will be erroneous in the presence of shunts or incorrect sampling.
<SPAN role=presentation tabIndex=0 id=MathJax-Element-1-Frame class=MathJax style="POSITION: relative" data-mathml='CO=VO2(SAO2−SVO2)∗O2C’>CO=VO2(SAO2−SVO2)∗O2CCO=VO2(SAO2−SVO2)∗O2C CO = VO 2 ( S A O 2 − S V O 2 ) ∗ O 2 C |
|
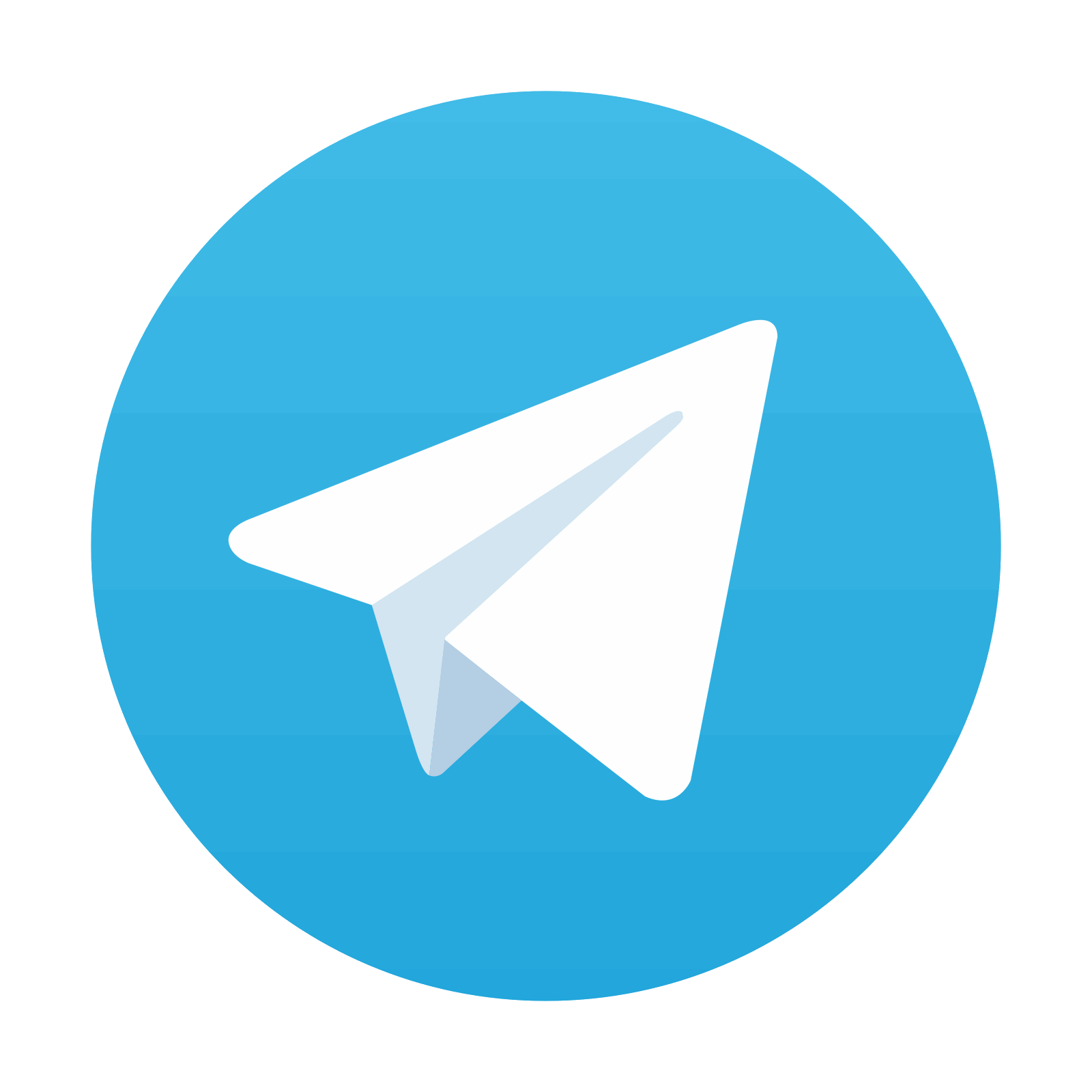
Stay updated, free articles. Join our Telegram channel

Full access? Get Clinical Tree
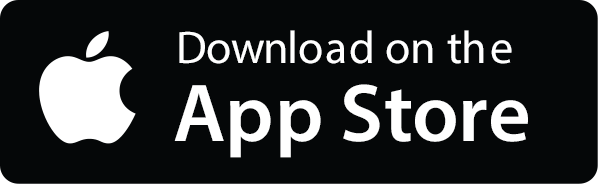
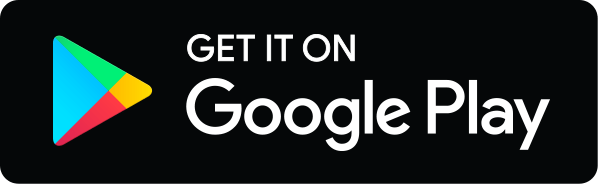
