Incidence
Survival (%)
0.5/1000
9
In-hospital [60]
0.17 ± 0.09 per bed/year
17
Cardiac arrest is a time-dependent, dynamic process as conceptualized by Weisfeldt and Becker in their 3-phase model of ventricular fibrillation cardiac arrest, which describes a progressive disruption of cardiac electrophysiology, circulation, and metabolism [5]. From cardiac arrest through the first 4 min is considered the electrical phase during which defibrillation is most likely to be successful [3, 6]. This phenomenon is most likely due to a time-dependent decay of a shockable ventricular fibrillation rhythm to a lower amplitude rhythm that is poorly responsive to defibrillation [7, 8]. Chest compressions gain importance after 4 min, which is likely due to the ischemic heart being less responsive to defibrillation [9]. Lastly, significant metabolic dysfunction (the metabolic phase) occurs at approximately 10 min as a result of global ischemia. Lack of return of spontaneous circulation by this time is associated with significantly worse survival.
Of course, there is considerable heterogeneity within the cardiac arrest population. The Weisfeldt-Becker model described above refers to ventricular fibrillation cardiac arrest, which is but one type of cardiac arrest. This heterogeneity extends beyond the type of cardiac arrest to such factors as age, gender, duration of arrest, and comorbidities among other factors, all of which make cardiac arrest treatment and cardiac arrest research extremely challenging.
Challenges aside, it is clear that aggregate outcomes can be improved by timely provision of specific interventions from lay people to paramedics, emergency department staff, and intensive care unit staff. Early identification and activation of the emergency response system, high-quality basic life support including good chest compressions, early defibrillation, expert application of advanced cardiac life support principles, and advanced post-resuscitation care can all improve patient outcome. Advances in care of the cardiac arrest patient have been seen with the advent of chest compressions, defibrillation, skilled airway management, therapeutic hypothermia, early percutaneous coronary intervention, and seizure control. Further incremental advances will be seen through careful resuscitation research, application of promising research findings, and meticulous attention to detail in the provision of postarrest care.
53.2 Overview: Current Advanced Cardiac Life Support (ACLS)
Management of cardiac arrest and the current approach to ACLS can be thought of in terms of the arrest phase and the postarrest phase. While there are interventions that may be applicable in either phase such as airway management, current research on management of the cardiac arrest patient tends to focus on early intervention from lay persons and EMS in the arrest phase and advanced care in centers of excellence in the postarrest phase. Figure 53.1 outlines the current ACLS algorithm for adult cardiac arrest.
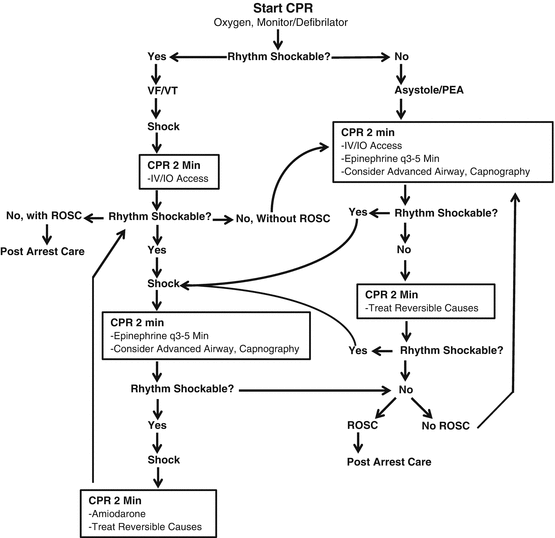
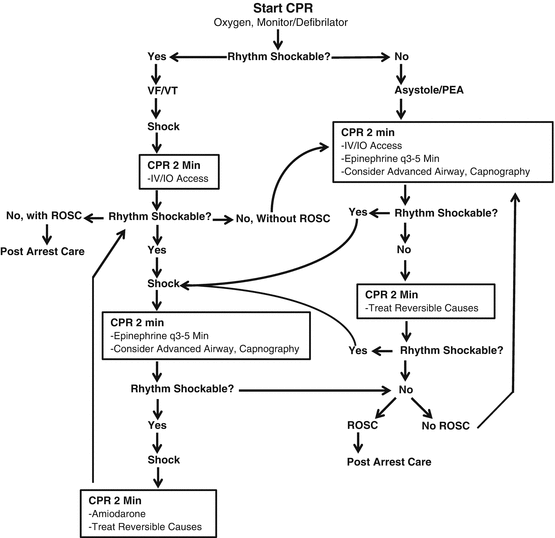
Fig. 53.1
ACLS algorithm for adult cardiac arrest
During the arrest phase, the focus must be on early defibrillation and high-quality chest compressions. Early defibrillation is best accomplished via the use of automated external defibrillator (AED) and activation of the emergency medical system. With regard to CPR, compression-only CPR is favored as it is thought to be as good or better than conventional CPR with ventilations [10–15]. However, there is evidence that conventional CPR may be superior in young patients, those with cardiac arrests of noncardiac origin, those with delay to CPR initiation, and those with prolonged arrests of cardiac etiology [10, 16].
Improving outcomes in the postarrest phase is dependent on multiple factors and is best managed in a hospital setting that enables multidisciplinary teams to work collaboratively, optimizing all aspects of the patient’s care. Such teams should be skilled in managing the brain injury, myocardial dysfunction, systemic ischemia/reperfusion injury, and persistent effects from the precipitating pathology during the immediate presentation. Further, these multidisciplinary teams should be experts in managing the physical and psychosocial aspects of medium- and long-term recovery to optimize outcome.
This chapter will focus on key aspects of prehospital and early hospital care in the cardiac arrest patient, with focused sections on each critical aspect of care.
53.2.1 Prehospital Care
Resuscitation of patients with out-of-hospital cardiac arrest (OOHCA) is based on the “chain of survival” concept, with links of early activation of EMS, early CPR, early defibrillation, advanced cardiac life support, and post-resuscitation care [17]. Optimal treatment of OOHCA in the prehospital setting is complex and requires time-sensitive, coordinated actions involving multiple groups, such as lay persons who can perform bystander CPR and use community-based AEDs and EMS providers to ensure high-quality CPR, early defibrillation, and initiation of ACLS, and transport to hospitals which can provide comprehensive post-resuscitation care including acute coronary interventions, therapeutic hypothermia, neurological care, and goal-directed critical care.
The importance of early CPR on survival in OOHCA has been well established, and early CPR is considered to double or even triple the survival rate [18–20]. In addition, a growing body of data points to the importance of high-quality CPR in achieving return of spontaneous circulation (ROSC) and improving outcomes [20, 21]. As such, public health initiatives have focused on educating the lay public about the importance and mechanics of CPR. As discussed above, compression-only CPR is now recommended for bystanders, with the intent of simplifying and removing barriers to its initiation. International guidelines endorse CPR with minimized interruptions, compressions of adequate depth (>5 cm) and rate (>100/min), and full release of pressure between compressions, as well as avoidance of excessive ventilation [22].
Defibrillation for ventricular tachycardia/ventricular fibrillation is another critical prehospital intervention for OOHCA. Despite an increased presence of AEDs in public places like airports and shopping malls, a minority of patients with OOHCA receive AED defibrillation prior to EMS arrival, suggesting that further expansion of public access defibrillation programs would be beneficial [23, 24].
The final component of the prehospital management of OOCHA is transfer to an appropriate facility. With increased recognition that aggressive, comprehensive post-cardiac arrest care improves outcomes, guidelines recommend that field providers should bypass local hospitals and go directly to a hospital capable of providing angiography within 90 min and helicopter transport for patients when anticipated time to angiography exceeds 90 min [25].
53.2.2 Early Hospital Care
While prehospital interventions like defibrillation and high-quality compressions are critical in achieving ROSC, increased attention has been focused on the role of in-hospital post-resuscitation care to help improve survival rates following cardiac arrest [12]. Epidemiological data on patients resuscitated from OOHCA suggest there is a wide variation in regional and institutional treatments as well as survival rates [26]. Accordingly, there have been increased efforts to define and characterize how elements of post-resuscitation care contribute to increased survival. Data suggests that hospitals that provide an integrated team approach to post-resuscitation care report improved survival rates [27].
The AHA’s 2010 guidelines for postarrest care list the following priorities [12]:
1.
Therapeutic hypothermia to optimize survival.
2.
Identify and treat acute coronary syndrome.
3.
Optimize mechanical ventilation to minimize lung injury.
4.
Support organ function to reduce risk of multiorgan failure.
5.
Assess prognosis for recovery.
6.
Assist survivors with rehabilitation services when needed.
53.3 Post-cardiac Arrest Syndrome
Post-cardiac arrest syndrome describes the global ischemic injury and reperfusion injury characterizing patients resuscitated from cardiac arrest. This complex pathophysiological state includes brain injury, myocardial dysfunction, and systemic ischemia/reperfusion response induced by the arrest event as well as underlying pathology which led to the arrest [26].
The brain is particularly vulnerable to injury in cardiac arrest due to its limited tolerance for ischemia as well as its unique response to reperfusion, which includes excitotoxicity, impaired calcium homeostasis, and activation of protease cascades and cell death signaling pathways [28, 29]. Furthermore, postarrest hypoxemia, hypotension, and impaired cerebral autoregulation can lead to secondary neurologic injury. Clinical manifestations of postarrest brain injury include coma, seizures, cognitive dysfunction, and death [30, 31]. Thus, strategies to reduce brain injury, such as therapeutic cooling and hemodynamic optimization, are core treatment elements following cardiac arrest.
Post-cardiac arrest myocardial dysfunction also contributes to the high mortality rate following cardiac arrest [26]. Importantly, data suggests that myocardial dysfunction, also known as “myocardial stunning,” is both responsive to therapy and reversible, again underscoring the need for aggressive postarrest care [32, 33].
53.4 Therapeutic Hypothermia
Therapeutic hypothermia, also known as targeted temperature management, is a mainstay of treatment to minimize postischemic brain injury in the patient who remains comatose after cardiac arrest. In 2002, two landmark randomized controlled trials (RCTs) demonstrated improved survival in adults with OOHCA from ventricular fibrillation (VF) treated with therapeutic hypothermia. The first study, a large European multicenter trial, reported a 16 % absolute improvement in outcomes in patients with VT/VF arrest cooled to a range of 32–34 °C, and the second study, performed in Australia, reported a 23 % improvement in patients randomized to cooling to 33 °C [34, 35]. In addition, more than 40 nonrandomized trials have reported improved outcomes with therapeutic hypothermia [36]. On the basis of this evidence, professional societies have been advocating therapeutic hypothermia in patients resuscitated after VT/VF cardiac arrest.
A recent international study comparing cooling temperatures has challenged the long-held dogma of therapeutic hypothermia’s benefits. Nielsen et al. randomized 950 patients with OOHCA of presumed cardiac cause to targeted temperature management of 33 °C versus 36 °C and found no difference in outcomes among the two groups [37]. This study has generated intense debate and raised numerous questions about therapeutic hypothermia, including its potential benefit, what the optimal temperature and timing of cooling is, whether avoidance of fever is the beneficial mechanism, or whether the rapid rewarming in the study negated any benefits of therapeutic hypothermia [38, 39]. Further research is needed to clarify optimal parameters for therapeutic hypothermia, which as yet remain undefined.
While no randomized controlled trials have compared hypothermia versus normothermia in patients with non-VT/VF cardiac arrest, observational studies suggest a possible benefit, and therapeutic hypothermia is often used as a treatment for cardiac arrest from non-shockable rhythms as well [40, 41]. Current guidelines recommend cooling comatose patients after VF cardiac arrest to 32–34 °C for 12–24 h (Class I, level of evidence B), with consideration for patients with cardiac arrest from non-shockable rhythms (Class IIb, level of evidence B) [12].
Therapeutic hypothermia can be divided into three phases: induction, maintenance, and rewarming. Induction can be initiated with intravenous ice-cold fluids (0.9 % saline or Ringer’s lactate 30 mL/kg), or ice packs placed on the groin, armpits, and around the head and neck [42–44]. This can be easily started in the prehospital setting. Once the patient arrives in the hospital, internal cooling devices, such as intravascular cooling catheters, are inserted into the femoral or subclavian veins and provide controlled temperature maintenance.
The goal of the maintenance phase is to maintain temperature at 32–34 °C and prevent significant temperature fluctuations, which is best achieved with internal cooling devices [26]. Neuromuscular blockade with sedation may be required to prevent shivering and its associated heat generation. While the optimal rewarming rate has not been defined, the current consensus is to warm at 0.25–0.5 °C per hour [41].
Complications of hypothermia include shivering, increased systemic vascular resistance which can reduce cardiac output, and arrhythmias, particularly bradycardia [40]. In addition, hypothermia triggers a cold diuresis, which can lead to hypovolemia and electrolyte disturbances, such as hypophosphatemia, hypokalemia, hypomagnesemia, and hypocalcemia, all of which can worsen arrhythmias. Electrolytes should be checked frequently and repleted if necessary. Finally, cold-induced impairment of platelets and clotting function can lead to coagulopathy and bleeding problems [26].
53.5 Early Cardiac Catheterization
Coronary artery disease (CAD) is present in the majority of patients with out-of-hospital cardiac arrest, and acute coronary syndrome (ACS) is the leading cause of sudden cardiac death [45]. A recent review showed acute coronary plaque disruptions in 40–86 % of cardiac arrest survivors and in 15–64 % of autopsy studies [46]. This research, along with similar work, suggests that patients resuscitated from VF arrest are likely to have an acute coronary occlusion, and has prompted investigation into early percutaneous coronary intervention (PCI) as a treatment for out-of-hospital arrest (Chap. 7) [25].
The efficacy of early PCI after OOHCA is well established [47, 48]. Studies of post-cardiac arrest patients with ST segment elevation myocardial infarction report angiographic success rates from 78 to 95 % and in-hospital mortality rates of 25–56 %, lower than typical cardiac arrest mortality rates [26]. In addition, studies with broader inclusion criteria, including cardiac arrest patients without ST elevation on EKG, also show promising results and suggest a mortality benefit to early PCI [47]. Many of these studies combine early PCI with therapeutic hypothermia and provide support of the feasibility and benefit of a combined approach [26].
Current guidelines endorse emergent PCI on patients with ROSC after cardiac arrest for patients who have ST segment elevation on EKG (Class I, level of evidence B). However, given the high prevalence of CAD in this population and the unreliability of initial EKG for the diagnosis of ACS, emergent PCI should be considered for patients with a presumed cardiac cause even in the absence of ST elevation on EKG [25]. Therapeutic hypothermia can be safely combined with emergent PCI. If no facility with PCI capability is available, consideration should be given for thrombolytics in patients with ST elevation myocardial infarction, although there is a paucity of data on thrombolytics in the post-cardiac arrest population [25].
53.6 Goal-Directed Therapy and Management of Cardiogenic Shock
Early hemodynamic optimization, or early goal-directed therapy, refers to an algorithmic approach which aims to restore and maintain the balance between systemic oxygen delivery and demands. While early goal-directed approaches have been shown to improve survival in septic shock, there is little data from randomized controlled trials of post-cardiac arrest patients [49]. However, there are numerous physiologic similarities between the postarrest and sepsis states, and current guidelines support a similar resuscitation strategy, consisting of targeting physiologic parameters with a priority on early initiation.
Based on available evidence, resuscitation targets in post-cardiac arrest patients include mean arterial pressure (MAP) of 65–100 mmHg, central venous pressure of 8–12 mmHg, and mixed venous O2 (ScvO2) >70 %, urine output >1 mL/kg/h, and a normal or decreasing serum lactate level [49, 50]. The optimal hemoglobin level has yet to be defined.
53.6.1 Mechanical Ventilation Strategies
A growing body of evidence points to the detrimental effect of hyperoxia in the post-resuscitation period, with a proposed mechanism of increased oxidative stress to vulnerable postischemic neurons [51, 52]. A recent study revealed that ventilation with 100 % oxygen in the first hour post-ROSC contributed to worse outcomes than titration of FiO2 (fraction of inspired oxygen) to maintain oxygen saturation of 94–96 % [53]. Therefore, current guidelines advocate an FiO2 of 100 % during CPR, but rapid titration of oxygen to maintain saturations of 94–96 % once ROSC has been achieved.
Another priority endorsed by the AHA for post-resuscitation care is optimization of ventilation. High tidal volumes can cause barotrauma and volutrauma in patients with acute lung injury [54, 55]. In addition, hyperventilation should be avoided as it can lead to cerebral vasoconstriction with resultant worsening of cerebral ischemia [56]. Likewise, hypoventilation with its associated hypoxia and hypercapnia can increase intracerebral pressure and compound preexisting metabolic acidosis in the postarrest state. Therefore, ventilation should be adjusted to avoid high volumes and to maintain normocarbia, with frequent arterial blood gas measurements to guide optimization of ventilatory parameters.
< div class='tao-gold-member'>
Only gold members can continue reading. Log In or Register a > to continue
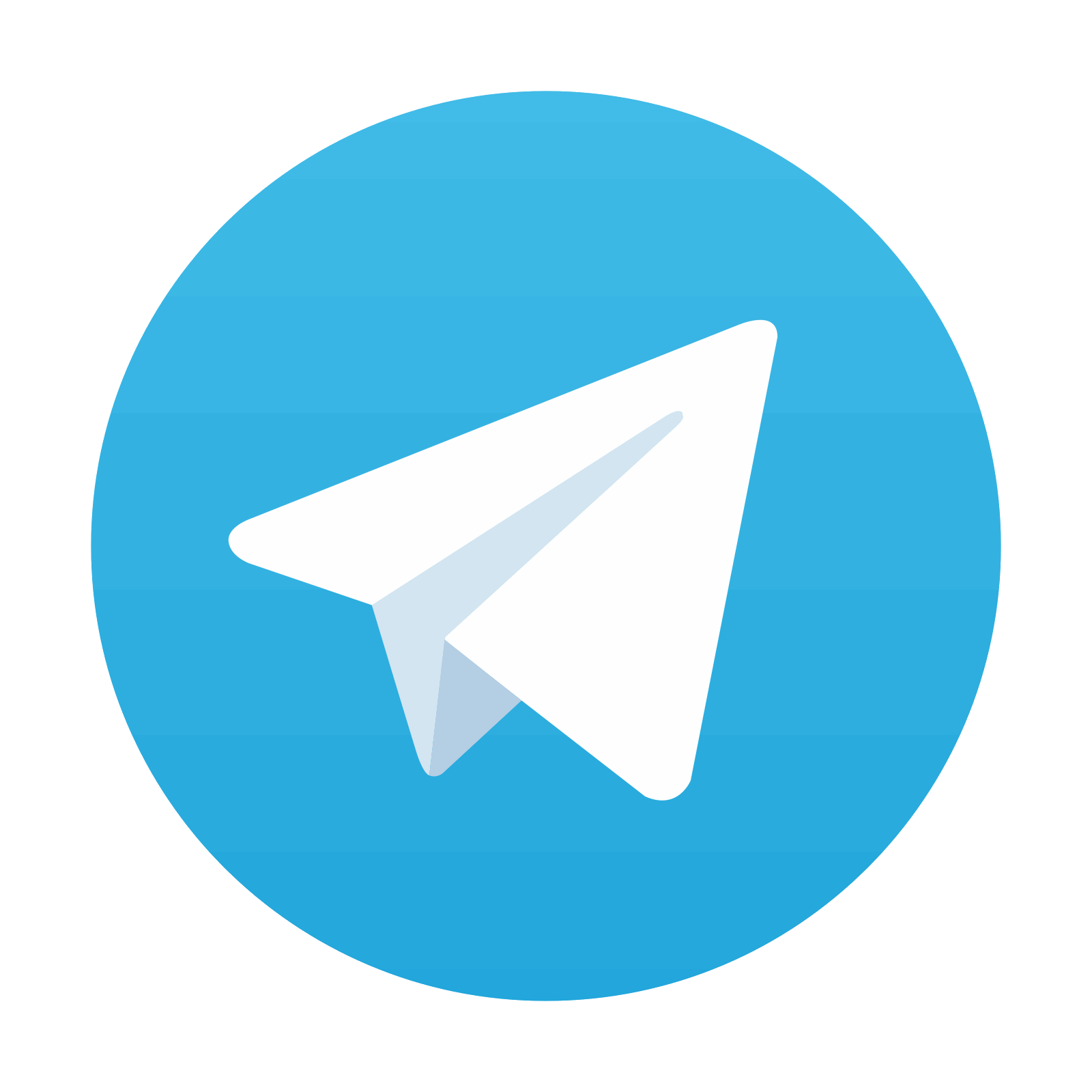
Stay updated, free articles. Join our Telegram channel

Full access? Get Clinical Tree
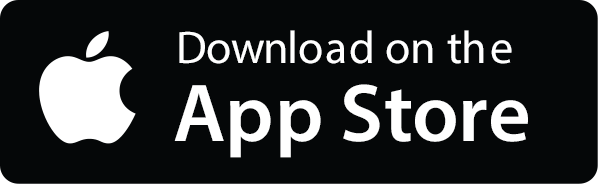
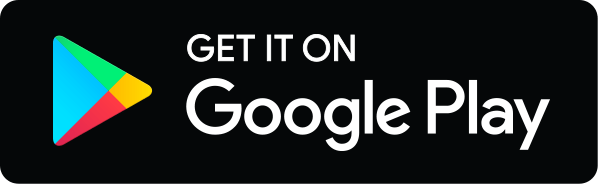