Heart disease is the leading cause of death in the United States, accounting for 600,000 fatalities annually.1 Coronary artery disease (CAD) is a complex and multifactorial process, primarily characterized by the progressive and silent accumulation of atherosclerotic plaques, fibrous materials, and inflammatory mediators within the coronary arteries, potentially leading to obstruction of flow and resultant myocardial hypoperfusion.2 Manifestations of this disease tend to present in males 40 years and older and in females 50 years and older. Risk factors include a strong family history of the disease, hypercholesterolemia, hypertension, diabetes, obesity, and smoking. If left untreated, CAD can lead to angina, heart failure, arrhythmias, and sudden death. Although medical therapy and percutaneous coronary interventions are often mainstay treatments for milder forms of the disease and most single- and double-vessel lesions, the gold standard for most multi-vessel lesions remains surgical revascularization.3,4
Anesthesiologists should be acutely aware of the heightened risks of myocardial ischemia for patients during revascularization surgery due to their obstructed coronary vessels and the critical dependence on oxidative phosphorylation for cardiac energy production. Myocardial oxygen supply is the product of arterial oxygen content and coronary blood flow.
Maximizing oxygen release at the tissue level involves maintaining high hemoglobin concentration, high oxygen saturation, warm temperature, and increased 2,3-diphosphoglycerate. The myocardium tends to maximally extract oxygen from its blood supply while at rest, and therefore, can only increase myocardial oxygen delivery during stress by increasing coronary blood flow. If this flow is obstructed by CAD, then the risk of myocardial ischemia is greatly increased. Coronary artery blood flow is proportional to coronary perfusion pressure (CPP), where
and is inversely proportional to coronary vascular resistance. Normal coronary arteries have autoregulated flow at CPPs between 50 and 150 mm Hg. Coronary vascular resistance is determined by hormonal and metabolic factors, the autonomic nervous system, endothelial modulation, and in diseased vessels, by atherosclerotic plaques. Left ventricular (LV) endocardial blood flow occurs entirely during diastole, when the vast majority of the remaining myocardium is oxygenated (Fig. 11-1). Therefore, the anesthesiologist attempting to maximize CPP and oxygen supply should target normal to increased diastolic blood pressure, low left ventricular end-diastolic pressure (LVEDP), and a slow heart rate to maximize time during diastole. Additionally, myocardial oxygen demand can be minimized by avoiding tachycardia, minimizing LVEDP, reducing chamber size, and reducing contractility. This general principle of optimizing myocardial oxygen supply while minimizing demand should be an over-reaching goal for the anesthesiologist caring for a patient undergoing coronary revascularization surgery.5,6
FIGURE 11-1
Wiggers diagram depicting relationship of left coronary artery pressure and flow with the phase of the cardiac cycle. Note that left coronary flow occurs primarily during ventricular diastole, even though the head pressure of the vessel is higher during systole. This is due to the high transmural ventricular pressure during systole, and the relatively low pressure in diastole as the myocardium relaxes, as governed by the coronary perfusion equation: CPP=DBP-LVEDP, where CPP=coronary pressure, DBP=diastolic blood pressure, and LVEDP=left ventricular end diastolic pressure. LCA=left coronary artery, LV=left ventricle. (Reproduced with permission from Lee J, Smith NP: The multi-scale modelling of coronary blood flow, Ann Biomed Eng 2012 Nov;40(11):2399-2413.)

When evaluating a patient prior to a coronary revascularization procedure, the anesthesiologist should be mindful of the anesthetic goals common to all surgeries: providing amnesia, analgesia, and a quiet surgical field while ensuring optimal myocardial oxygen supply and demand to minimize ischemic risk. In addition, the anesthesiologist should provide optimal hemodynamic control to optimize cardiac performance, and be prepared to help guide the surgery while rapidly diagnosing cardiac complications, often by using advanced noninvasive and invasive hemodynamic monitors including transesophageal echocardiography (TEE).
Initial preoperative patient assessment should include a comprehensive history and physical examination focused on the cardiac and pulmonary systems, along with a standard airway examination. Though direct laryngoscopy is routinely used to facilitate tracheal intubation, a predicted difficult airway may prompt alternative intubation strategies. A thorough review of the medical record is in order, with careful attention to patient medications, laboratory studies (complete blood count, electrolytes, creatinine, troponin, B-type natriuretic peptide, coagulation panels, blood cross matches), electrocardiogram, carotid ultrasound studies, chest radiograph, cardiac stress tests, cardiac catheterization, and echocardiography reports. The anesthesiologist should be aware of the coronary anatomy and lesions, along with any invasive pressure measurements as described by the catheterization report. Close review of any preoperative echocardiography studies is essential to assess both systolic and diastolic ventricular function and to examine the cardiac valves.
A comprehensive preoperative assessment is important to determine the perioperative risk. The two most widely used risk stratification tools are the Society of Thoracic Surgeons (STS) Risk Calculator and EuroSCORE II, each based on large historical patient databases. These formulae use sophisticated mathematical modeling techniques to allow the user to determine predicted mortality and other complications based on a variety of entered criteria, including age, gender, ejection fraction, urgency of surgery, renal function, and other variables. Risk assessment calculators can be valuable preoperative planning tools for the patient, surgeon, and anesthesiologist, and their use should be considered prior to each case.7,8
While concluding the preoperative visit, the anesthesiologist should ensure informed consent and provide the opportunity for any patient questions to be answered. The anesthesiologist’s visit itself is a powerful means to allay patient fears and helps address patient and family members’ concerns.9 Additionally, the anesthesiologist should consider any premedications to administer, include anxiolytics as necessary. Beta-blocker medications are recommended preoperatively to reduce the risk of postoperative atrial fibrillation, myocardial ischemia, and mortality. Statins should be administered unless contraindicated to reduce the rate of myocardial ischemia, graft atherosclerosis, and mortality.10,11 In general, antihypertensives should be continued on the day of surgery, while use of angiotensin-converting enzyme inhibitors and angiotensin II receptor antagonists is associated with intraoperative hypotension and remains controversial. The use of antiplatelet and anticoagulation agents should be discussed with the surgeon.12
Finally, the anesthesiologist should have an active preoperative meeting with the surgeon and the surgical team, as necessary, in order to facilitate the procedure and minimize complications. Checklist and protocol-driven processes have demonstrated reduced complications and improved mortality.13 Cardiac anesthesiologists with formal fellowship training and skill in TEE are recommended to provide care for high-risk patients.14
A vigilant cardiac anesthesiologist is the best single monitor for patients undergoing revascularization procedures. Standard American Society of Anesthesiology monitors should also be used, including FiO2 monitor, pulse oximeter, end-tidal CO2 monitor, continuous electrocardiogram, noninvasive blood pressure monitor, and a temperature measurement device. In addition to these standard monitors, a urinary catheter often with bladder thermistor, an arterial catheter most commonly placed in the radial artery, and a central venous catheter are all used routinely. Central venous catheter placement using real-time ultrasound guidance is safer than using an anatomic-guided approach, and is the recommended technique when available.15
The risk of intraoperative awareness is increased during cardiac surgery due to the lower use of volatile anesthetics and increased hemodynamic instability in comparison to noncardiac surgery.16 Processed electroencephalograms, such as the bispectral index (BIS) monitor, have been used to detect inadequate patient amnesia with recent meta-analysis data suggesting that their use reduces the risk of intraoperative awareness in high-risk patients with an odds ratio of 0.24.17 Although this risk reduction is not universally found18 and the use of the BIS remains controversial, the National Institute for Health and Clinical Excellence recommends “the use of electroencephalography-based depth of anesthesia monitors…during any type of general anesthesia in patients considered at higher risk.”19
The technique of near-infrared spectroscopy allows for cerebral regional oxygen saturation to be measured using skin sensors placed on the scalp. Low cerebral tissue oxygen values are associated with increased long-term surgical risk and can also detect cerebral hypoperfusion states caused by problems with bypass cannulae, emboli, and obstruction of cerebral vessels. Cerebral oximetry is often used with aortic surgery involving circulatory arrest, and less commonly with isolated coronary artery bypass grafting (CABG) procedures.20 Cerebral oximetry has yet to be clearly demonstrated to be beneficial in high-quality randomized intervention trials, and its use remains controversial.21,22
The pulmonary artery catheter (PAC) is frequently used during revascularization procedures as a means of measuring cardiac output via thermodilution and directly measuring pulmonary artery pressures. Additionally, LVEDP, as a marker of LV preload, can often be inferred from the pulmonary artery occlusion pressure or the pulmonary artery diastolic pressure. The use of a PAC is not without risk however, and it has been associated with rare but often lethal complications including pulmonary artery rupture. PAC use for revascularization surgery became widespread by the 1980s but its current use is declining due to the proliferation of less-invasive cardiac output monitoring devices, TEE, and some data which fails to demonstrate an association with improved outcome.23,24 Even so, PACs remain popular with more than two-thirds of anesthesiologists reporting that they use this catheter more than 75% of the time in cases requiring cardiopulmonary bypass (CPB).25 The perioperative use of a PAC remains recommended in patients with cardiogenic shock (Class I indication), hemodynamic instability (Class IIa), and its use may be reasonable in stable patients undergoing revascularization procedures (Class IIb).26
TEE has become a routine monitor during cardiac surgery, and has been shown to have a significant favorable influence on intraoperative surgical management and outcomes.27 Current practice guidelines recommend that unless contraindicated, TEE be used for all valvular heart procedures and should be considered in CABG surgeries.28 Recent global survey data suggest that TEE is used in 61 to 69% of isolated CABG procedures.29 In addition to serving as the gold standard for valve evaluation, TEE can be used to monitor volume status, ventricular function, and gauge regional-wall motion patterns to detect and assess myocardial ischemia—all useful assessments during revascularization surgery.29
Historically, general anesthesia was commonly induced and maintained with high-dose fentanyl (50 to 100 mcg/kg) and pancuronium (Table 11-1). This high-dose opiate technique purportedly avoided myocardial depression associated with other anesthetic agents while providing stable hemodynamics and effective blunting of surgical stresses.30 In the 1990s, efforts at cost reduction spurred the evolution of “fast-track” cardiac anesthetic techniques using volatile anesthetics and low-dose opiates, with early extubation and reducing ICU stays as primary goals. Fast-track techniques have subsequently been demonstrated to be as safe as historical anesthetics in low- and medium-risk patients.31 Around the same time, the concept of ischemic and pharmacological preconditioning was described, whereby certain maneuvers or pharmacologic agents including volatile anesthetics could minimize the extent of myocardial damage to subsequent myocardial ischemia.32 As a result, contemporary approaches to coronary revascularization surgery commonly involve induction with thiopental, propofol, etomidate, or midazolam, followed by a balanced anesthetic technique employing a volatile anesthetic (ie, isoflurane, desflurane, or sevoflurane) as its primary component. The volatile anesthetic is accompanied by a low-dose semisynthetic opiate (ie, fentanyl, sufentanil, or remifentanil) and a paralyzing agent. There is no significant difference in extubation times between fentanyl, sufentanil, and remifentanil using fast-track protocols.33 The use of a volatile anesthetic, and therefore induction of pharmacologic preconditioning, has now become a more common standard anesthetic approach. Meta-analyses suggest that cardiac surgery mortality is reduced when using a volatile anesthetic as compared to a propofol-based intravenous anesthetic, with an odds ratio of 0.51 in favor of volatile anesthetics.34 Xenon is a promising inhalational anesthetic agent that purports to improve recovery from myocardial stunning, preserve hemodynamic control, and provide neuroprotective qualities. Early trials have been favorable, but its use remains experimental.35
Agent | Dose | Notes | |
---|---|---|---|
High-dose opiate technique | |||
Fentanyl | 50-100 mcg/kg load | Technique now less commonly used due to increased risk of delayed extubation, increased risk of intraoperative awareness, and lack of ischemic preconditioning volatile anesthetic. | |
Pancuronium | 0.1 mg/kg load, then 0.01 mg/kg as needed | Mild tachycardic effect may help offset bradycardia associated with high opiate technique. Must reduce dose in renal impairment. | |
Fast-track balanced anesthetic | Routinely used. | ||
Induction | Propofol | 1-3 mg/kg | |
IV induction agent | Thiopental | 3-5 mg/kg | |
Etomidate | 0.2-0.4 mg/kg | Associated with adrenal suppression. | |
Midazolam | 0.05-0.15 mg/kg | ||
Maintenance | |||
Opiates | Fentanyl | 10-20 mcg/kg | Typically given as initial load during induction, with intermittent boluses as necessary. |
Sufentanil | 0.5-2.0 mcg/kg | Typically given as initial load, with intermittent boluses or infusion as necessary. | |
Remifentanil | 0.05-1.0 mcg/kg/min | Administer long-acting analgesics prior to discontinuing remifentanil. | |
Volatile anesthetic | Isoflurane | MAC 1.13% | Titrate to effect, target 1 MAC for ischemic preconditioning. |
Sevoflurane | MAC 2.05% | Titrate to effect, target 1 MAC for ischemic preconditioning. | |
Desflurane | MAC 7.25 % | Titrate to effect, target 1 MAC for ischemic preconditioning. | |
Paralytic | Rocuronium | 0.6 mg/kg load, then 0.15 mg/kg as needed | |
Vecuronium | 0.1 mg/kg load, then 0.01 mg/kg as needed | ||
cis-Atracurium | 0.15-0.20 mg/kg load, then 0.02 mg/kg as needed | Safe for renal failure patients. | |
Total IV anesthetic | |||
Propofol | 50-150 mcg/kg/min | Combine with opiate and paralytics as above. Lacks benefit of volatile anesthetic-mediated ischemic preconditioning. Likely increased mortality when compared to volatile anesthetic-based technique. |
Neuroaxial techniques are occasionally used during revascularization surgery. However, systemic heparinization increases the concern of epidural hematoma and resultant spinal cord injury thereby limiting their use, although the actual incidence of this complication is very low.36 Preoperative intrathecal opiate injection and continuous thoracic epidural local anesthetics can provide good analgesia and facilitate recovery; however, current data do not demonstrate a reduction in the risk of mortality, myocardial infarction, or neurological complications as compared to general anesthetic alone.37 Awake cardiac surgery is performed in a very small number of centers using thoracic epidural anesthesia and may offer rapid recovery benefits in select patients.38
Patients undergoing coronary revascularization frequently develop hypotension and/or low cardiac output, particularly following CPB. Risk factors for low cardiac output syndrome include renal disease, significant regional-wall motion abnormalities, CABG with mitral valve (MV) surgery, reoperation, moderate-to-severe mitral regurgitation (MR), prolonged aortic cross clamp time, left ventricular ejection fraction (LVEF) < 40%, cardiac index < 2.5 L/min/m2, and LVEDP ≥ 20 mm Hg.39,40 Strict attention to hemodynamic monitors coupled with a command of cardiac physiology and pharmacology will aid the anesthesiologist in maintaining adequate systemic perfusion. Markers of inadequate systemic perfusion include low cardiac index, hypotension, oliguria, metabolic acidosis, increased base deficit, and elevated serum lactate.
The anesthesiologist is tasked with preventing, diagnosing, and treating inadequate systemic perfusion, and implementing an algorithmic approach may help direct therapy (Fig. 11-2). By using a combination of traditional hemodynamic monitors, invasive and noninvasive cardiac output monitoring devices, dynamic indexes, changes in end-tidal carbon dioxide, arterial blood gas analysis, and echocardiography, systemic perfusion can be assessed and treatment adjusted. A number of vasoactive medications are available and their use should be individualized based on each patient’s hemodynamic needs (Table 11-2). A recent addition is levosimendan, a novel inotrope that increases troponin C affinity to calcium without increasing myocardial oxygen consumption or impairing ventricular relaxation, while also exerting pharmacologic preconditioning effects by opening mitochondrial KATP channels. Levosimendan is recommended for treatment of acute heart failure in Europe41 and has shown mortality benefits when compared to dobutamine, but is not yet available in the United States. If after optimizing volume status and maximizing pharmacologic support the patient remains hypoperfused, then mechanical assist devices should be considered.42 The intra-aortic balloon pump (IABP) is well established and technically easiest to place; however its efficacy has been recently questioned.43 In centers trained in their use, ventricular assist devices (VAD) and extra-corporeal membranous oxygenators (ECMO) may confer a survival benefit and should be considered.
a | ß1 | ß2 | V1 | HR | CO | SVR | MAP | RBF | Dosing | Notes | |
---|---|---|---|---|---|---|---|---|---|---|---|
Epinephrine | + | ++ | ++ | 0 | ++ | ++ | +/– | + | – – | 0.01-0.20 mcg/kg/min | May give up to 1 mg intravenously at a time during hemodynamic collapse, repeating as necessary. |
Norepinephrine | +++ | ++ | 0 | 0 | – | – | +++ | +++ | – – – | 0.01-0.20 mcg/kg/min | |
Dopamine | ++ | ++ | + | 0 | + | +++ | + | + | +++ | 2-20 mcg/kg/min | |
Dobutamine | 0 | +++ | + | 0 | + | +++ | 0 | + | ++ | 2-20 mcg/kg/min | Mild vasodilation at low doses, vasoconstriction at higher doses. |
Isoproterenol | 0 | +++ | +++ | 0 | +++ | +++ | – – | +/– | – | 0.01-0.07 mcg/kg/min | Use generally limited to heart transplants. |
Ephedrine | ++ | + | + | 0 | ++ | ++ | + | ++ | – – | 10-25 mg SIVP | Marked tachyphylaxis. Not used as infusion. |
Phenylephrine | +++ | 0 | 0 | 0 | – | – | +++ | +++ | – – – | 50-100 mcg SIVP, 0.3-10 mcg/kg/min | Routinely used to treat mild vasodilation. Causes reflexive bradycardia. |
Vasopressin | 0 | 0 | 0 | +++ | 0 | 0 | +++ | +++ | – | Up to 40 units IV bolus, 0.01-0.1 units/min | |
Milrinone | 0 | 0 | 0 | 0 | + | +++ | – – | – – | – | Bolus up to 50 mcg/kg over 10-30 min, then 0.125-0.75 mcg/kg/min | Phosphodiesterase inhibitor. May be effective for pulmonary hypertension and right ventricular dysfunction can cause systemic hypotension. |
Levosimendan | 0 | 0 | 0 | 0 | + | ++ | – | – | + | Bolus 12-24 mcg/kg over 10 min, 0.05-0.3 mcg/kg/min | Inodilator and calcium sensitizer, not available in the United States. |
The anesthetic care of a patient undergoing CABG is a complex, multi-step process. After the anesthesiologist completes a thorough preoperative evaluation, a large bore peripheral IV is placed followed by an arterial catheter, usually placed in the radial artery. Anxiolytics are routinely administered in the preoperative area. In the operating room, standard ASA monitors are applied, and intravenous induction and tracheal intubation are performed, most commonly with a fast-track approach (Table 11-1), targeting early extubation unless contraindicated. A central venous catheter is then placed if not placed prior to induction, often with PAC. Then, the patient is positioned and draped for surgery while a TEE is performed and results are discussed with the surgeon. Prior to incision, an antifibrinolytic is commonly administered (eg, aminocaproic acid, tranexamic acid) to reduce perioperative bleeding. Antibiotics (typically vancomycin and cefuroxime) are given as infection prophylaxis. Anesthetic depth is adjusted to match changes in surgical stimulation with attention to hemodynamic control and myocardial oxygen supply/demand optimization. Heparin is administered several minutes prior to bypass cannulae placement (usually 300 to 400 units/kg), and its effect confirmed by measuring an activated clotting time (ACT) value of 480 seconds or greater prior to initiating bypass. Inadequate ACT values must prompt action by the anesthesiologist to determine the cause and achieve proper anticoagulation before initiating CPB. The surgeon and perfusionist should be kept notified of the ACT values and any difficulties in anticoagulation. Heparin resistance may require treatment with fresh frozen plasma or antithrombin III concentrate to achieve adequate heparin effect.
After heparinization, the surgeon places an arterial cannula and a venous cannula. Upon initiation of CPB, mechanical ventilation is stopped, the heart is arrested, and revascularization is performed. During this time, volatile anesthetic is typically administered via the bypass circuit by the perfusionist under the anesthesiologist’s and surgeon’s direction. ACT, hematocrit, arterial blood gases, and blood sugar should be checked periodically during CPB, and any aberrations treated. Serum glucose levels > 180 mg/dL should be treated with insulin infusion.
After the revascularization is complete, the patient should be weaned from bypass. The anesthesiologist should anticipate bypass separation and be prepared, following a standardized routine. Separation preparation steps include: ensuring function of intravascular catheters, ensuring adequate depth of anesthesia, assessing for intracardiac air with TEE, ensuring that core temperature ≥ 36°C, performing a lung recruitment maneuver, and resuming normal ventilation. Establishing an adequate cardiac rhythm and rate, generally 80 to 100 beats per minute may require a pacemaker. Normalizing electrolytes, treating anemia, and preparing vasoactive medications are also important considerations. Though most patients will have adequate cardiac function with a ventricular pacemaker lead, some patients dependent on active atrial contraction for ventricular filling (eg, severe diastolic dysfunction) will require atrial pacing. Biventricular pacing may improve cardiac output to a small degree, which may be clinically important in patients with severe cardiac dysfunction. The actual process of separation requires intense focus and frequent communication amongst the surgeon, anesthesiologist, and perfusionist, and therefore should be conducted in a quiet, “sterile cockpit” environment.44
Once the anesthesiologist, surgeon, and perfusionist are prepared to wean from CPB, the anesthesiologist should work to ensure systemic perfusion and cardiac performance by optimizing heart rate and rhythm, infusing volume from the CPB machine to achieve normovolemia, adjusting afterload with vasodilators or vasopressors as necessary, and optimizing contractility with inotropes as needed. This dynamic process occurs while gradually reducing CPB machine flows to minimal support, at which point cardiac function is reassessed, and then pump flow is stopped completely. If cardiac function is not adequate, then steps to correct this are taken prior to separation.
Immediately after separation, the heart is assessed for adequate function, particularly for markers of ischemia (eg, wall motion abnormalities, worsened ventricular function, inadequate graft flow), valvular abnormalities, and for preload, afterload, and contractility. Frequently, adjustments in volume therapy and vasoactive medicines are made, guided primarily by TEE and hemodynamic monitors. After achieving sufficient hemodynamic parameters generally targeting SBP 100 to 120 mm Hg, and a CI ≥ 2.2 L/min/m2, protamine is slowly given prior to the bypass cannulae being removed.
Following separation, the anesthesiologist must remain vigilant and continue to control and optimize patient hemodynamics. Hemostasis should be assessed by examining the surgical field, measuring ACT and heparin concentration, and in certain cases with thromboelastography. Cardiac output should be measured again after chest closure and support adjusted as necessary. Transfer to the ICU should conclude with a detailed, clear handoff to the ICU team in order to minimize communication failures.
Surgical coronary revascularization can also be performed on a beating heart without CPB, and is termed “off-pump” coronary artery bypass (OPCAB). OPCAB typically utilizes a heart stabilization device that isolates a localized region of surgical interest immobile while allowing the rest of the heart to beat. OPCAB became popularized in the late 1990s and early 2000s, eventually becoming the surgical approach for nearly one-third of coronary revascularizations in the United States. OPCAB purported to reduce the surgical inflammatory stress response, mortality, stroke, cognitive dysfunction, and kidney injury. However, recent meta-analyses suggest that there is no significant difference in cognitive dysfunction between OPCAB and CABG,45 and that all-cause mortality in OPCAB (3.7%) was slightly higher than CABG (3.1%).46 Furthermore, the need for subsequent revascularization may be higher in OPCAB.47 As a result, the use of beating heart techniques in the United States is declining.
The OPCAB lends itself to fast-track anesthetic regimens, and in some centers, this is augmented with thoracic epidural analgesia. Heparinization protocols for OPCAB are variable, though many surgeons target ACT values of 300 seconds. Ischemic preconditioning techniques should definitely be considered, given that transient ischemia is likely during the grafting procedure. Surgical exposure may lead to marked alterations in the heart’s position within the chest, potentially dramatically impacting cardiac output. The anesthesiologist should be prepared to monitor systemic perfusion most commonly with TEE and continuous cardiac output monitors, and treat hypoperfusion with volume and inotropes as necessary. Occasionally, despite hemodynamic optimization, adequate perfusion cannot be maintained, necessitating conversion to an on-pump procedure. As a result, a perfusionist and CPB machine should be available during OPCAB.
Minimally invasive approaches to coronary revascularization, either on- or off-pump are becoming more common, and involve either a shorter sternotomy incision or any of various small thoracotomies for access to the coronary arteries. Robotic-assisted techniques allow for remote surgical access via small incisions or ports into the chest cavity. The anesthesiologist must communicate with the surgeon preoperatively to discuss the operative strategy and plan accordingly. Often, lung isolation with a double-lumen endotracheal tube or bronchial blocker facilitates surgical visualization. Anesthesiologists should also be prepared to aggressively avoid hypothermia and ensure that external defibrillator pads are placed due to the limited direct access to the heart.48
The perioperative care of patients with valvular heart disease creates a considerable challenge for anesthesiologists, surgeons, and intensivists. A great deal of challenge stems from the wide diversity of pathophysiologic derangements found in these patients. This diversity can, in part, be appreciated by comparing representative LV pressure-volume loops for the different left-sided valvular lesions (Fig. 11-3). Clearly, there are significant and important distinctions in end-diastolic LV volume, intracavitary pressure, and stroke volume. However, not only do the major valvular lesions differ from one another in terms of adaptive ventricular responses and hemodynamic profiles, but patients with the same valvular lesion may present with entirely different degrees of symptomatology and hemodynamic compromise depending on the clinical situation and time course over which the valve pathology develops. Again, LV pressure-volume loops illustrate this concept. The patient who develops acute, severe aortic regurgitation (AR) due to endocarditis will present with notably different LV filling volumes and pressures when compared to a patient with a similar regurgitant fraction whose AR develops gradually due to progressive aortic root dilatation (Fig. 11-4). Clinical management must be appropriately adapted.
Another complicating factor in the management of patients with valvular heart disease is the frequent occurrence of multiple valvular lesions in the same patient. General hemodynamic goals for patients with left-sided valve pathology can be easily summarized (Table 11-3). However, not uncommonly, two valve lesions coexist in which hemodynamic goals are opposite. For example, selecting an ideal heart rate for the patient with aortic stenosis (AS) becomes more difficult in the setting of concomitant MR. In these situations, the physician either determines which lesion contributes most to the patient’s symptoms, signs, and hemodynamic profile or chooses a management strategy that represents a compromise between competing goals.
Although the spectrum of valvular heart disease encompasses a wide variety of hemodynamic findings and adaptive ventricular responses, the natural history of many valve disorders often follows a similar general course. During the initial development of valvular stenosis or regurgitation, the patient remains asymptomatic. Ventricular remodeling serves to normalize indices such as wall tension or stroke volume. Atrial enlargement may mitigate increases in pulmonary or systemic venous pressure. Eventually, following a variable latent period, adaptive responses fail leading to the elevation of cardiac filling pressures, exhaustion of preload reserve, and declining cardiac output. Signs and symptoms of congestive heart failure (CHF) develop or evidence of ventricular dysfunction manifests during imaging studies prompting surgical intervention. The optimal timing of surgical intervention for valvular heart disease continues to evolve, guided by clinical investigations and expert consensus.49
Aortic stenosis represents the most common valve disorder in the United States.50 Two reasons help explain this observation. First, a congenital bicuspid aortic valve (AV), which has an increased tendency to become stenotic over time, is found in 1 to 2% of the population. Second, risk factors for degenerative calcification of a trileaflet AV such as hyperlipidemia, hypertension, smoking, and advanced age are also common. Obstruction to outflow creates abnormal systolic pressure (P) within the LV cavity, which in turn, increases wall tension (T) according to the law of Laplace:
where R represents the radius of the ventricular cavity and h represents myocardial wall thickness. Increases in wall thickness by the process of concentric myocyte hypertrophy serve to normalize wall tension, although this remodeling invariably leads to diastolic dysfunction and creates the setting for oxygen supply and demand mismatch. Thus both LV outflow restriction and the pathophysiology of concentric hypertrophy guide perioperative management. For example, tachycardia is particularly deleterious. Increased heart rates disproportionately encroach on diastolic filling and coronary perfusion time in a ventricle where myocardial relaxation is delayed. At the same time, tachycardia increases myocardial oxygen consumption. Elevated end-diastolic pressures in an LV with reduced compliance limit coronary perfusion to subendocardial regions of the hypertrophied myocardium. Reduced LV compliance also makes the LV more dependent on atrial contraction for diastolic filling and atrial fibrillation may be poorly tolerated. Synchronized cardioversion should be employed early in the patient with severe AS who develops atrial fibrillation with signs of low cardiac output.
The anesthesiologist must consider the pathophysiology of outflow obstruction as well as consequences of concentric LV hypertrophy when selecting a perioperative management plan. Since patients with severe AS are very sensitive to anesthetic-induced decreases in preload and afterload, an arterial line is often placed prior to induction to allow early recognition of blood pressure changes and response to treatment. The choice of induction agent is probably not as important as adherence to key hemodynamic goals that include the maintenance of preload and afterload along with a heart rate in the low to normal range. Hypotension is treated promptly, usually with an α-adrenergic agonist. Opioids such as fentanyl are often included in a balanced anesthetic induction and maintenance regimen since they tend to lower heart rate and minimally impact myocardial contractility. High-dose opioid anesthetic regimens, more popular in preceding decades, provide hemodynamic stability but may hamper early postoperative extubation. Drugs that increase heart rate, such as ketamine or pancuronium, should be used cautiously or in conjunction with vagotonic agents such as opioids. However, while avoidance of tachycardia represents an important perioperative goal, anesthesiologists must also avoid severe bradycardia.51 In patients with AS, flow across the AV limited and cardiac output declines with heart rates less than 50 beats per minute. When treating bradycardia, drugs such as β-adrenergic agonists or anticholinergics should be titrated carefully to avoid overshoot and the development of tachycardia.51 Atrial or atrio-ventricular pacing represents an attractive option for the cardiac surgical patient since it allows precise titration of heart rate.
In addition to an arterial line, central venous access along with a PAC is generally selected for patient undergoing aortic valve replacement (AVR). Central venous access is desirable for the administration of vasoactive medications although central venous pressure (CVP) does not accurately reflect LV filling pressures in patients with severe AS. Likewise, the pulmonary capillary wedge pressure (PCWP) also does not provide a reliable assessment of LV filling in the setting of AS and reduced ventricular compliance. Measuring end-diastolic LV cavity area with TEE provides a more accurate reflection of preload compared with PAC-derived measurements.51 Nonetheless, despite the limited ability to assess preload and the small risk of arrhythmia during insertion, the PAC offers several advantages including the opportunity to track cardiac output, measure pulmonary artery (PA) pressure, and serve as a conduit for a temporary pacing wire.
Aortic regurgitation (AR) leads to significant volume overloading of the LV and when chronic, the pressure-volume loop shifts farther to the right than for any other valvular lesion (Fig. 11-3). The eccentric LV hypertrophy and chamber dilatation that develop in the setting of chronic AR serve to normalize wall tension and accommodate very large end-diastolic volumes without significant increases in end-diastolic pressure. Patients may remain asymptomatic for years, though eventually compensatory changes are exhausted and in the face of progressively larger ventricular volumes, LV systolic function declines. In cases of acute severe AR, compensatory LV changes are not present. End-diastolic pressure rises quickly and may reach aortic diastolic pressure. In such cases, the combination of a low aortic diastolic pressure and a high LV end-diastolic pressure limit CPP, creating the potential for ischemia.
The hemodynamic goals for patients with severe AR differ from those used to manage AS patients. When the regurgitant fractions exceed 60%, maintenance of cardiac output requires an increase in heart rate.51 A faster heart rate also reduces the diastolic period available for regurgitant flow into the LV. Avoidance of opioid-induced bradycardia along with the use of a muscle relaxant that increases heart rate such as pancuronium may prove useful. A reduction in afterload promotes forward flow. Propofol, which reduces afterload and displays minimal effects on myocardial contractility at usual clinical doses, represents a reasonable option for the induction of anesthesia.52 Additional afterload reduction in the form of vasodilators such as sodium nitroprusside may be selected. Because the LV may be severely dilated and LV systolic may be depressed even in asymptomatic patients,51 inotropic support should be readily available. Agents with β-adrenergic agonist activity, such as dobutamine along with phosphodiesterase inhibitors such as milrinone, increase myocardial contractility while favoring a reduction in afterload.
The hemodynamic monitors chosen for patients with severe AR undergoing AVR are similar to those selected for patients with AS. Intra-arterial pressure monitoring, central venous cannulation, and PAC placement are generally used together with intraoperative TEE. As in the case of AS, the measurement of PCWP may not accurately reflect LV filling, particularly in the case of severe, acute AR where the rapid rise of LV end-diastolic pressure may promote closure of the MV in late diastole. However, many anesthesiologists find the ability to obtain serial measurements of cardiac output to be beneficial. Furthermore, a PAC provides an easy route by which a pacing lead may be placed prior to sternal opening, facilitating the maintenance of an elevated heart rate.
Rheumatic disease accounts for most cases of acquired mitral stenosis (MS) in developed countries. Patients with rheumatic MV disease may remain asymptomatic for many years as the left atrium (LA) progressively dilates. Symptoms develop when severe MS limits LV filling and cardiac output. Alternatively, patients with moderate MS may become symptomatic during periods of increased transvalvular flow such as pregnancy or conditions associated with tachycardia.51 As shown in the simplified Gorlin equation, for a given valve area, when transvalvular flow increases, the pressure gradient also rises.
Rising LA pressure promotes pulmonary venous congestion and leads to feelings of dyspnea. Over time, pulmonary hypertension develops which may, in turn, lead to right ventricular (RV) enlargement, RV systolic dysfunction, and functional tricuspid regurgitation (TR). A major goal in the perioperative care of patients with severe MS is the identification and quantification of RV impairment.
The anesthetic management of patients with severe MS undergoing MV replacement is guided by several principles. First, tachycardia, which shortens diastolic LV filling time and increases LA pressure, should be treated promptly. The judicious use of preoperative sedation may ameliorate anxiety-induced tachycardia although excess sedation promotes hypoventilation with the attendant risk of increased pulmonary vascular resistance. A number of different agents may be chosen to reduce heart rate including calcium channel blockers, β-blockers, or amiodarone. Atrial fibrillation is commonly found in patients in this patient group and is generally tolerated provided the ventricular rate is controlled. Therefore, unlike patients with severe AS who develop atrial fibrillation, the immediate focus in patients with MS should be to maintain a low to normal ventricular rate rather than conversion to sinus rhythm. Second, because LV filling is already impaired by the stenotic MV, additional reductions in preload caused by anesthesia or blood loss should be carefully corrected. Vasodilation resulting from inhaled or intravenous anesthetics may be treated with incremental doses of an α-adrenergic agonist or vasopressin, ideally while monitoring PA pressures. Surgical blood loss should be replaced though overly zealous fluid administration should be avoided to prevent pulmonary vascular congestion and further RV impairment. While the PCWP does not reflect LV end-diastolic pressures in the setting of severe MS, display of PA pressures and serial measurement of cardiac output may be useful in guiding fluid administration. A third principle in the management of these patients involves control of PA pressures and augmentation of the RV systolic function. Because of the aforementioned need to maintain preload, the use of systemic vasodilators, though they may reduce PA pressure, may be poorly tolerated in this group. Instead, a select pulmonary vasodilator such as inhaled prostaglandin or nitric oxide should be considered for those with pulmonary hypertension and RV dysfunction. Additionally, arterial blood gas management is essential. The avoidance of hypoxia together with mild hyperventilation, combine to reduce pulmonary vascular resistance.
Following MVR with normalization of left-sided preload, LV systolic performance is expected to improve. However in a subset of patients, LV systolic performance may be impaired following valve replacement. Possible causes include rheumatic endocarditis or an unfavorable position of the ventricular septum in the setting of pulmonary hypertension and RV enlargement.51 Intraoperative TEE readily detects abnormalities of ventricular septal position and RV dysfunction in addition to providing a qualitative and quantitative assessment of LV systolic performance. Inotropic support may be required.
Mitral regurgitation shares some similarities with AR. Both conditions increase the preload of the LV and over time, eccentric hypertrophy develops. Like those with AR, patients with MR may remain asymptomatic for long periods. Similar to AR patients, those with MR present for surgery with a spectrum of clinical and imaging findings. The patient with MV prolapse who is followed with serial echocardiograms over a decade differs in important ways from the patient with acute, severe MR due to papillary muscle rupture. The former may well remain asymptomatic or minimally symptomatic and be referred for surgery due to abnormalities in LV size or function, while the latter is likely to present in heart failure with florid pulmonary edema and require intra-aortic balloon pump support preoperatively.
In patients with chronic MR, the contractile appearance of the LV in MR patients often improves initially in response to preload augmentation according to the Frank-Starling principle. Furthermore, the ejection of large quantities of blood into the low pressure LA leads to increases in the calculated ejection fraction although forward cardiac output may decline. An LVEF < 60% identifies reduced systolic performance in patients with severe MR and represents an indication for surgery.49,53 Physicians have also recognized that an early decline in LVEF following MV surgery for MR is common. Although sometimes considered an inevitable consequence of MV surgery for MR, significant reductions in LV function postoperatively associated with an ejection fraction < 40% may identify a group at increased risk for premature death.54 Several reasons have been postulated to explain reduced LV systolic performance following MV surgery, including reduced preload and ischemic cross-clamp injury55 as well as unrecognized, preexisting systolic dysfunction.54 Impaired preoperative LV systolic impairment may be underappreciated by echocardiography.56 Several risk factors identify patients with MR who are at increased risk for low ejection fraction following mitral surgery, including preoperative pulmonary hypertension, preoperative atrial fibrillation, and a preoperative LV end-systolic diameter > 36 mm which confers a 6.5-fold increased risk of having a postoperative LVEF < 40%.54,55 By assessing preoperative risks factors, anesthesiologists and surgeons can better prepare for the possible use of pharmacologic and mechanical circulatory support after MV surgery. Inotropic agents such as epinephrine and milrinone should be available. Early consideration should be given to placement of an IABP if hemodynamic compromise is refractory to drug therapy.
Hemodynamic goals for patients with severe MR include a reduction in the regurgitant fraction with augmentation of forward flow. For those patients with longstanding MR and pulmonary hypertension, support of the RV is also important. Providing that LV systolic function is not severely impaired, most patients tolerate an intravenous induction of anesthesia. Agents such as propofol reduce afterload and promote forward flow. Bradycardia is avoided since lower heart rates facilitate greater LV filling and distention. Inhalation agents are good choices for the maintenance of anesthesia as they also provide vasodilatation and may increase heart rate slightly. In patients with chronic MR, the LA is usually dilated. Atrial fibrillation may be present but is generally well tolerated since LV filling is unimpeded.
The popularity of robotic and other minimally invasive approaches to MV surgery continues to grow. Advantages of these approaches include reduced time to extubation, shortened hospital length of stay, lower blood product transfusion rates, and improved postoperative respiratory function.57,58 The use of robotic and minimally invasive techniques necessitates changes in anesthetic management. These changes include the need for one-lung ventilation, the percutaneous placement of cannulae for CPB and retrograde cardioplegia, the expanded use of TEE (Table 11-4), and altered strategies for defibrillation. Smaller incisions and reduced need for opioid medications also create the potential for more rapid extubation and the expanded use of regional anesthesia.
|
Both thoracoscopic and robotic mitral repair utilize a small working incision and additional port access in the right chest. This approach requires deflation of the right lung. Either a double-lumen endotracheal tube or a bronchial blocker may be used to facilitate the operation.59 Oxygen desaturation associated with one-lung ventilation is particularly problematic following CPB and may be treated by the application of continuous positive airway pressure (CPAP) to the nonventilated right lung, the use of positive end-expiratory pressure (PEEP) with the left lung, or intermittent two-lung ventilation.60
Cannulation for CPB for these operations usually consists of femoral arterial and venous access with or without supplementary venous drainage in the form of a vent line in the pulmonary artery or a superior vena cava cannula placed percutaneously from the right internal jugular vein. Surgeon preference and institutional experience guide the cannulation strategy. Intraoperative TEE is used to verify the position of the guidewire and femoral venous cannula. Inadvertent placement of a femoral venous guidewire across a patent foramen ovale is possible and should prompt redirection (Fig. 11-5). Supplementary venous drainage from the main pulmonary artery can be accomplished by means of a specialized endovent, the tip of which should be located in the main PA, near the bifurcation (Fig. 11-6A). Again, intraoperative TEE is used to ensure proper placement. TEE imaging can be difficult in these cases requiring the placement of multiple lines in the right heart (Fig. 11-6B).
FIGURE 11-6
(A) A potential venous cannulation strategy for robotic mitral valve repair using a multi-orificed cannula inserted via the femoral vein and positioned with its tip in the superior vena cava along with supplemental drainage provided by a vent placed percutaneously into the pulmonary artery. (B) Transesophageal echocardiogram demonstrating a venous cannula in the right atrium and superior vena cava (arrows) along with a pulmonary artery vent (arrowheads).

Aortic cross clamping and cardioplegia administration can be accomplished by several different mechanisms. An endo-aortic balloon may be advanced from the femoral artery into the ascending aorta where inflation serves to isolate the aortic root and facilitate antegrade cardioplegia delivery from the end of the delivery catheter. A percutaneous coronary sinus catheter may also be placed via the right internal jugular vein using TEE or fluoroscopic guidance to allow for the administration of retrograde cardioplegia. Lastly, an antegrade cardioplegia and vent line may be placed via a parasternal stab incision, a strategy similar to many open cardiac operations (Fig. 11-7A). In this last scenario, a long transthoracic aortic cross-clamp is used via a small lateral thoracic incision. The position of this cardioplegia line (Fig. 11-7B) and subsequent cardioplegia delivery (Fig. 11-7C) can be monitored with TEE.
FIGURE 11-7
(A) Surgical site photo of a patient undergoing robotic mitral valve repair. A percutaneous antegrade cardioplegia line is seen (open arrow) along with a right internal jugular vein introducer sheath (solid arrow) used facilitate placement of a percutaneous superior vena cava cannula. (B) Transesophageal echocardiogram demonstrating the tip of the percutaneous antegrade cardioplegia line in the proximal ascending aorta (arrow). (C) Antegrade cardioplegia administration via a percutaneously placed line.

Small incisions preclude the use of internal paddles for defibrillation and cardioversion in robotic MV surgery. Instead, external patches are applied in an anterior-posterior orientation on the left chest.61 Should defibrillation prove difficult, consideration should be given to inflating the right lung to improve electrical impedance.59
Minimally invasive mitral surgery facilitates rapid extubation, particularly when regional anesthesia is used.57 Multiple regional anesthetic techniques have been used in these cases including intrathecal opioid administration, intercostal nerve block, and paravertebral nerve block. The use of preoperative paravertebral nerve block together with a limited opioid dosing strategy was shown to facilitate extubation in the operating room following robotic mitral repair in approximately 90% of cases in one study.57
Tricuspid regurgitation (TR) found in patients presenting for cardiac surgery is most often functional in nature and occurs secondary to left-sided valve disease, pulmonary hypertension, or RV dysfunction. As such, the anesthetic management of these patients is usually dictated by the primary condition. However, patients may present with TR due to isolated, organic tricuspid valve (TV) disease. Examples include endocarditis, carcinoid valvulopathy, trauma, or pacemaker-induced valve dysfunction.
General hemodynamic goals for patients with severe TR include support of RV contractility, maintenance of a mildly elevated heart rate, and reduction of RV afterload by reducing pulmonary vascular resistance. Phosphodiesterase inhibitors such as milrinone may augment RV contractility while reducing RV afterload. Dobutamine and isoproterenol represent other possible options. As in cases of pulmonary hypertension or RV systolic dysfunction, careful attention to arterial blood gas values provides an important nonpharmacologic means by which the anesthesiologist can manipulate pulmonary vascular resistance.
In addition to direct arterial pressure monitoring, CVP monitoring is nearly always used for patients undergoing TV surgery even though CVP is a poor indicator of RV filling in the setting of severe TR. Although it may be difficult to advance in the face of severe TR, consideration can be given to PAC placement especially if TV repair or replacement with a tissue prosthesis is contemplated. A PAC facilitates assessment of pulmonary vascular resistance and thermodilutional cardiac output measurement becomes more feasible following TV surgery. The measurement of cardiac output by arterial waveform analysis provides the anesthesiologist with a reasonable alternative to placement of a PAC. These devices can be connected to any invasive arterial monitoring line. Early comparative studies with thermodilutional techniques were disappointing though the updated software in newer models may deliver more robust results.62,63 The use of TEE can be invaluable in assessing not only valvular pathology but RV function as well. An additional important use of TEE in isolated TV surgery is the identification of intracardiac shunts since in the absence of shunting, CPB without a cross clamp becomes feasible.
The spectrum of diseases of the thoracic aorta may be classified according to pathology, etiology, timing, clinical presentation, location, and management options.64-66 Thorough preoperative assessment including a comprehensive history and physical exam, review of preoperative imaging studies, and patient stability all play a role in determining whether the patient can be treated medically with continued surveillance, or surgically with definitive intervention.64-66 When surgery is warranted, the urgency and planned surgical approach to repair significantly influence the anesthetic plan. Factors such as the cannulation strategy for CPB, the need for temporary cessation of blood flow, and anticipated methods for organ protection determine the optimal anesthetic technique and monitoring choices during thoracic aortic surgery. While routine monitoring involved in all cardiac surgery patients applies to aortic surgery as well, the anesthesiologist must further individualize management to the patient’s disease and the surgeon’s operative approach. Hemodynamic goals, especially blood pressure management, can be significantly different from those of the cardiac surgical patient presenting for nonaortic surgery. Maintaining organ perfusion and protecting major organs, specifically those subjected to periods of ischemia, is crucial in allowing for optimal outcomes. Additional monitoring to detect and treat end-organ ischemia is another factor in procedural success. Furthermore, complex thoracic aortic surgery associated with prolonged procedure times and hypothermia frequently results in postoperative coagulopathy that can be effectively managed with anticipation of bleeding and transfusion requirements. In addition, reliance on intraoperative TEE in guiding the operation, managing isolated single-lung ventilation to allow for adequate surgical exposure, and placement of lumbar cerebrospinal (CSF) drainage catheters are also essential aspects of the operation that give the cardiac anesthesiologist a key role in the surgical treatment of thoracic aortic disease. Recent multi-disciplinary guidelines for thoracic aortic diseases include evidence-based recommendations regarding anesthetic techniques and intraoperative monitoring.64-66 This review of anesthetic and monitoring considerations for thoracic aortic surgery will be presented in the following three sections: (1) aortic root/ascending aorta; (2) aortic arch; and (3) descending thoracic aorta.
Surgical intervention for the aortic root and ascending aorta may be isolated to these aortic segments or may continue distally into the arch and beyond. Surgery for ascending aorta pathology above the root may often involve partial reconstruction of the aortic arch as well.67 When isolated to the level of the aortic root, the aortic arch may not be included in the surgical intervention. Typically, surgical indications for ascending aortic intervention include dissection, intramural hematoma, and aneurysm.64-67 Factors such as patient age, connective tissue disorder, family history, and bicuspid aortic valve must all be taken into account when finalizing the surgical plan.64-67
The surgical approach to these cases is via a midline sternotomy, and therefore, patients will be positioned supine with both arms tucked and padded at their sides. Along with a balanced general endotracheal anesthetic, these patients typically have an arterial line and PAC placed for hemodynamic monitoring. The maintenance of normotensive blood pressure is suitable for patients with chronic aortic disease. When presenting for elective surgery, preoperative antihypertensive therapy should be titrated to achieve a blood pressure less than 140/90 mm Hg or less than 130/80 mm Hg in patients with diabetes or chronic renal disease (Class I recommendation; Level B evidence).64,65 Preoperative therapy may even be titrated in known aortic aneurysm patients to achieve the lowest possible blood pressure that is tolerated without adverse effects (Class IIa recommendation; Level B evidence).64,65 In acute pathologies of the ascending aorta such as dissection, more aggressive treatment to a systolic pressure of 100 to 120 mm Hg is recommended since aortic rupture is a major concern in this phase.64,65 This strict control of systolic blood pressure is typically achieved with adequate anesthetic depth, titrated analgesia with opioids such as fentanyl and morphine, and vasodilator therapy with agents such as beta-blockers, nitroprusside, nicardipine, or morphine. Intravenous beta-blockade is typically avoided in the settings of significant aortic regurgitation and/or cardiac tamponade since cardiac output in these presentations remains rate-dependent.64,65,68 Since acute aortic dissection is dynamic and progressive, patients may become acutely hemodynamically unstable, emphasizing the importance for not only being prepared for these scenarios, but also the importance of a prompt anesthetic induction to minimize delays and control patient anxiety and hypertension.
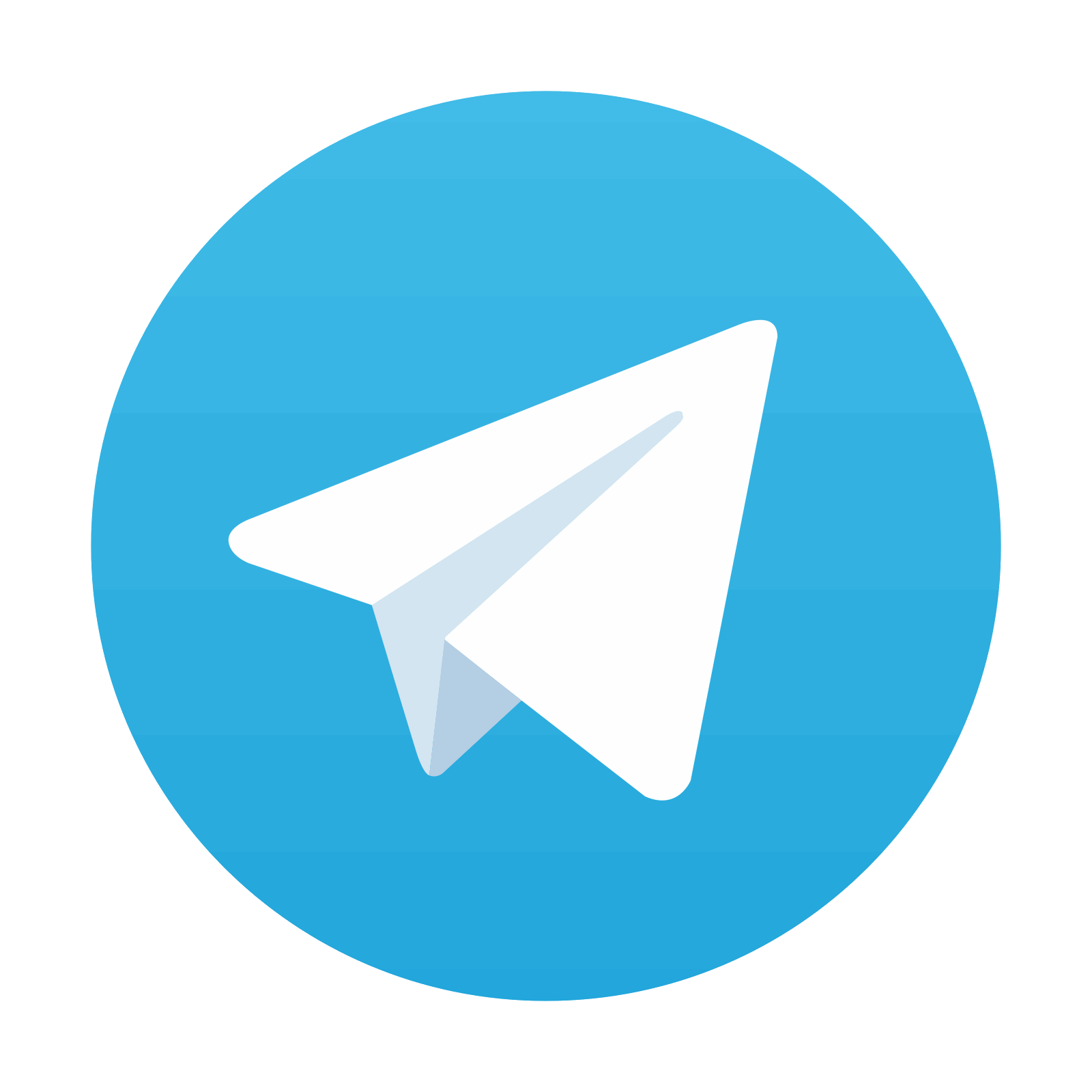
Stay updated, free articles. Join our Telegram channel

Full access? Get Clinical Tree
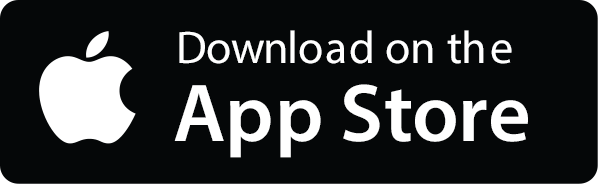
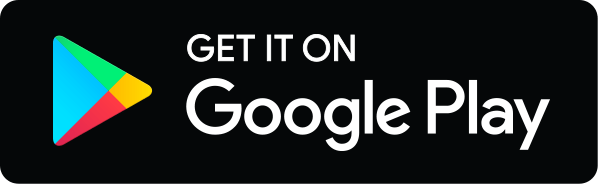