Cardiac anatomy
Introduction
The heart is the major organ of the mediastinum and is contained within the middle mediastinum, enclosed by the pericardium (Figure 4.1). It is a dual muscular pump, forcing blood around the pulmonary and systemic circulations. The heart can be split into a right side and left side that act simultaneously but on separate circulatory systems. The heart can also be divided into four chambers: two on the right and two on the left. These chambers all have a valve at their exit to prevent back flow of blood. Both sides have an atrium, which receives blood from the venous system; and a ventricle, which propels blood around the associated circulatory system. The right side pumps blood to the lungs around the pulmonary circulation, while the left side pumps blood around the rest of the body via the systemic circulatory system. The two systems have different lengths and pressures and the two sides of the heart therefore have slightly different morphologies to accommodate this (Moorjani, Viola & Ohri 2011b, Anderson & Cook 2014, Drake, Vogl & Mitchell 2015).
Although the heart can be described as ‘left and right’ or as’ four chambers’, it is all one organ formed by a continuous network of specialised muscle fibres – the myocardium. Within the myocardium runs the conduction system, and the connective tissue of the cardiac skeleton. The muscle fibres and the path of the cardiac conducting system are oriented so that contraction of the muscle is synchronised to efficiently expel blood from the heart (Drake, Vogl & Mitchell 2015).
Pericardium
The fibrous pericardium (the outermost layer of the pericardium) forms the boundary of the middle mediastinum. The cavity within the fibrous pericardium, which houses the heart, is lined by serous pericardium. The serous pericardium can be further subdivided into the parietal and visceral layers; the parietal layer is attached to the inner surface of the fibrous pericardium and the visceral layer, known as the epicardium, is closely attached to the heart (Sinnatamby 2011).
The parietal and visceral layers are continuous with each other at the sites where blood vessels penetrate the pericardium and along folds of pericardium, between these vessels, posterior to the heart, that tether it in position. These folds create two pockets within the pericardium: the oblique sinus, posterior to the left atrium and bounded by the inferior vena cava, the four pulmonary veins and the folds of pericardium connecting them; and the transverse sinus, a smaller pocket lying posterior to the aorta and pulmonary trunk, bound by the superior vena cava, the two superior pulmonary veins and the fold of pericardium connecting them (Moorjani, Viola & Ohri 2011b). The space between the two layers of serous pericardium, including the two sinuses, is called the pericardial space. This space is usually very small, containing approximately 5–10ml fluid, which is continuously secreted and absorbed by the mesothelial cells that line the serous pericardium (Moorjani, Viola & Ohri 2011b). The fibrous pericardium is inelastic and maintains a fixed volume. This means that, in the event of a build-up of fluid within the pericardium, such as a bleed into the pericardial space or an inflammation causing a pericardial effusion, the heart is compressed and its function restricted (Okum & DeAnda Jr 2014).
Chambers of the heart
The heart is composed of four chambers: two atria (left and right) and two ventricles (left and right). Atria are collecting chambers, receiving blood that is returned to the heart. Ventricles are pumping chambers, with thick muscular walls that contract to eject blood at high pressure. The right-sided chambers collect blood from the systemic circulation and pump it around the pulmonary circulation. The left-sided chambers collect blood from the pulmonary circulation and pump it around the systemic circulation. The different demands of each system, and the different embryological origin and requirements of each chamber, influence their structure (Sinnatamby 2011, Anderson & Cook 2014).
Right atrium
The right atrium is a deformed vertical tube at the right border of the heart. Posteriorly, its inner surface is smooth and continuous from the inferior vena cava (IVC) inferiorly to the superior vena cava (SVC) superiorly. Anteriorly, there is a triangular pouch (the right atrial appendage or auricle) which curves medially to slightly overlie the aortic root. The interior surface of the anterior wall is trabeculated by ridges of pectinate muscle. The margin between these distinct interior surfaces is delineated by a smooth ridge of muscle, running on the lateral wall from the anterior edge of the SVC orifice above to the anterior edge of the IVC orifice below, called the crista terminalis. At the inferior end of the right atrium, just medial to the opening of the IVC, is the opening of the coronary sinus. Where these two orifices come together, each is guarded by a small thin fold of fibrous tissue: the eustachian valve guarding the IVC, and the thebesian valve guarding the coronary sinus. They join at a common fibrous ridge on the posterior atrial wall, the tendon of Todaro.
The medial surface of the right atrium has the right atrioventricular valve, the tricuspid valve, which separates the right atrium from the right ventricle. The septum between the right atrium and the left forms its posterior wall. A small oval-shaped depression, called the fossa ovalis, is visible in the septum. The tissue within this oval makes up the septum primum. The pronounced superior border of this oval, called the limbus, marks the extent of a deep infolding of intra-atrial wall that provides a double layer septum between the right and left atria, the septum secundum.
From outside the heart, these two layers can be separated to allow the surgeon access to the left atrium without entering the right. If the septa primum and secundum are not properly fused, they may overlie each other but permit a pathway between the right and left atrium, a persistent foramen ovale. In this instance, the way the septum secundum overlies the septum primum forms a one-way valve between the atria, with the higher pressure in the left atrium holding it shut. This is therefore usually a benign asymptomatic condition but it can have implications if the haemodynamics change (Moorjani, Viola & Ohri 2011b, Sinnatamby 2011, Anderson & Cook 2014).
Right ventricle
The right ventricle lies to the left and slightly anterior to the right atrium. Its anterior free wall constitutes the major component of the anterior surface of the heart. In cross-section, it is crescent-shaped, as the interventricular septum bulges slightly into the right ventricle. This reflects the lower pressure in the pulmonary circulation, and the reduced radial force that right ventricular walls are required to produce to eject blood.
It is helpful to consider the structure of ventricles in three parts: the inlet, the apical trabecular and the outlet components. Blood travels in through the inlet, and is directed down towards the apex, where it makes a sharp turn and is directed superiorly into the outflow tract. The inlet from the right atrium is guarded by the tricuspid valve. The apical trabecular component is so named because its inner surface is notable for the multiple ridges and bridges of muscle, called trabeculae, that cover it. In the right ventricle, the trabeculae are large and distinct – coarse trabeculae. The pattern of these trabeculae is mostly variable but there is some commonality found between patients. The most consistent trabeculae have distinct names and functions.
Papillary muscles are portions of muscle that protrude from the inner surface of the ventricle, with their origin in the ventricular wall and their insertion into the chordae that suspend the tricuspid. The right ventricle has up to three papillary muscles:
• The anterior papillary muscle, the largest and most consistent papillary muscle, on the anterior wall, supporting the anterior leaflet and commissure between the anterior and posterior leaflets
• The medial papillary muscle, attached to the interventricular septum and inserting into the chordae of the septal leaflet and zone of apposition between the septal and anterior leaflets
• The posterior papillary muscle, which arises from the posterior wall and anchors the chordae of the posterior leaflet and septal-posterior commissure.
Another consistent trabecula is the septomarginal trabecula. This arises from the septum at the base of the medial papillary muscle, courses towards the apex and bridges the ventricle, to attach to the anterior wall at the anterior papillary muscle. The bridging portion of this trabecula is called the ‘moderator band’ and the portion on the septum the ‘septal band’. The parietal band is a ridge of muscle separating the tricuspid valve from the outflow tract. It is continuous with the septomarginal trabecula on the interventricular septum. The outlet of the right ventricle is a smooth-walled muscular sleeve known as the infundibulum (Moorjani, Viola & Ohri 2011b; Sinnatamby 2011; Anderson & Cook 2014; Harington, Mora & Wu 2014; Drake, Vogl & Mitchell 2015).
Left atrium
A simpler structure than the right atrium, the left atrium is a smaller, flatter chamber, corresponding to the posterior surface of the heart. Its posterior wall is smooth and it has two venous orifices on each side where the right and left superior and inferior pulmonary veins drain. To the right, its anterior wall forms the inter-atrial septum, with a corresponding depression at the site of the fossa ovalis. To the left, there is a pocket of trabecular muscle, which wraps around the right ventricular outflow tract: the left atrial appendage. The left atrial appendage can take various shapes. One study categorised these shapes into ‘chicken wing’, ‘cactus’, ‘wind sock’ and ‘cauliflower’. It is often indicated as a site for clot formation in atrial fibrillation – a cause of stroke. The ‘cauliflower’ morphology is thought to be associated with the highest risk; ‘chicken wing’ the least. The appendage can be surgically excluded in an attempt to attenuate stroke risk. On the anterior wall of the left atrium is the opening into the left ventricle, occupied by the left atrioventricular valve, known as the mitral valve (Moorjani, Viola & Ohri 2011b; Sinnatamby 2011; Di Biase et al. 2012; Anderson & Cook 2014; Harington, Mora & Wu 2014).
The mitral valve occupies the inlet of the left ventricle, with the apical trabecular component anchoring it via chordae and two papillary muscles (the anterolateral and the posteromedial papillary muscles), lying in the anatomical positions described by their names. Both muscles have chordae to both mitral valve leaflets but the anterolateral muscle supplies more to the anterior leaflet, and the posteromedial more to the posterior leaflet. The trabeculae are finer in the left ventricle than the right and the chamber is slightly bigger.
The outlet of the left ventricle (the aortic antrum) is smooth walled and runs vertically, posterior to the infundibulum. Medially, the thin-walled membranous septum separates the left ventricle from the right atrium, just below the aortic valve. Posteriorly, between the anterior leaflet of the mitral valve and the aortic valve, is a non-muscular, fibrous area forming the aorto-mitral curtain. In cross-section, through the trabecular zone, the left ventricle is circular, with thick walls and a muscular septum. This allows maximum radial force to expel blood (Moorjani, Viola and Ohri 2011b; Sinnatamby 2011; Anderson & Cook 2014; Harington, Mora and Wu 2014; Drake, Vogl & Mitchell 2015).
Arterial blood supply
The blood supply to the myocardium is derived from the coronary arteries, which are the first branches of the ascending aorta. The word coronary comes from the Latin corona, which means ‘crown’ and describes how the arteries appear to encircle the heart. There are two main arteries, the left coronary artery and the right coronary artery, which arise from the left and right coronary sinuses respectively, just superior to the aortic valve. It is important to be aware that there are variations in the coronary anatomy. The coronary arteries are often visible on the surface of the heart, covered just by epicardium. They may, however, be covered by epicardial fat, or have a deeper intramuscular course (Moorjani, Viola & Ohri 2011b).
Left coronary artery
The left coronary artery begins at the left coronary ostium on the posterior surface of the aorta as the left main stem (see Figure 4.2).
Figure 4.2: The coronary angiogram of the left coronary artery, with its branches
Left anterior descending artery
The LAD courses towards the apex of the heart anteriorly and inferiorly in the anterior interventricular groove, overlying the interventricular septum. It gives rise to diagonal and septal branches. The diagonal branches travel laterally to the left to supply the anterolateral wall of the left ventricle (usually 2–6 diagonal branches) and the septal branches (between 3 and 5) penetrate deep into the muscle to supply the anterior two-thirds of the interventricular septum.
The LAD can be further divided into thirds. The proximal third begins after the left main stem and terminates at the first septal branch. The middle third arises from the first septal to the start of the last diagonal branch; and finally the distal third is found between the last diagonal and where the LAD terminates at the apex. Occasionally the LAD will also give right ventricular branches, which travel medially to supply the anterior surface of the right ventricle (Moorjani, Viola & Ohri 2011b; Sinnatamby 2011).
Circumflex artery
The circumflex artery runs along the atrioventricular groove and passes laterally to the left to the posterior surface of the heart supplying the left atrium and left ventricle. The main branches are the obtuse marginal arteries which travel anteriorly to supply the lateral wall of the left ventricle and the anterolateral papillary muscle of the mitral valve. There are smaller left atrial branches that travel posteriorly to supply the left atrium. In 40% of patients the circumflex artery will also give a branch to supply the sinoatrial node, and in 10 to 15% of patients it gives a branch to supply the atrioventricular node (Moorjani, Viola & Ohri 2011b).
Right coronary artery
The right coronary artery (RCA) begins at the right coronary sinus and courses anteriorly and laterally (see Figure 4.3). In 60% of individuals it gives a branch near its origin which ascends to the sinoatrial node. It descends along the right atrioventricular groove giving off an acute (or right) marginal branch which runs anteriorly along the antero-inferior margin of the right ventricle, supplying the right ventricle and apex. The RCA then courses posteriorly giving off the Atrio-Ventricular Nodal branch in 85–90% of patients at the crux of the heart (junction where septa and walls of the four chambers meet). The RCA continues as the posterior left ventricular artery (supplying the posterior left ventricular wall) (Ellis 2006, Moorjani, Viola & Ohri 2011b; Sinnatamby 2011; Drake, Vogl & Mitchell 2015).
Posterior descending artery
In 85–90% of individuals the posterior descending artery (PDA) arises from the RCA on the inferior surface of the heart as it crosses the crux. In 10–15% of individuals the circumflex system is more developed and the PDA arises at the same point as it is crossed by the circumflex artery. In approximately 5% of cases both the RCA and the circumflex are well developed and both give a supply to the PDA. The PDA runs along the posterior interventricular groove towards the apex of the heart and gives off septal perforators deep into the interventricular septum, which supply its posterior third. It also provides the only blood supply to the posteromedial papillary muscle of the mitral valve (Moorjani, Viola & Ohri 2011b).
Dominance
Dominance of the coronary arteries is determined by which artery gives rise to the posterior descending artery. Around 80–85% of individuals are right dominant, 10–15% left dominant and 5% co-dominant. Left dominance is slightly more frequent in men and is associated with bicuspid aortic valves (Moorjani, Viola & Ohri 2011a, 2011b).
Collateralisation
In some areas, it is possible that small branches from one cardiac artery can anastomose from the branches of another. This allows for collateral flow of blood to supply the dependent myocardium of a vessel if it is blocked proximally. These anastomoses may be congenital or may be formed by angiogenesis in the presence of chronic myocardial ischaemia (Moorjani, Viola & Ohri 2011b; Meier et al. 2013).
The venous drainage of the heart is through cardiac veins that mainly accompany the arteries. These veins drain into the right atrium via the coronary sinus: a large valveless vein that runs in the posterior atrioventricular groove. The great cardiac vein accompanies the LAD, then the circumflex artery and drains end to end into the coronary sinus in the atrioventricular groove. The transition point is marked by the valve of Vieussens. The middle cardiac vein accompanies the PDA and drains into the coronary sinus near its opening into the right atrium. The small cardiac vein runs around the right border of the heart in the right atrioventricular groove with the RCA and acute marginal artery. It drains into the coronary sinus near its opening.
The posterior vein of the left ventricle and oblique vein of the left atrium drain into the coronary sinus towards its transition to the great cardiac vein. The posterior vein of the left ventricle drains the posterior wall of the left ventricle, and the oblique vein of the left atrium descends across the posterior wall of the left atrium. The anterior cardiac veins drain the anterior surface of the right ventricle and drain directly into the right atrium. Thebesian veins (also known as the smallest cardiac veins or venae cordis minimae) drain directly into the chambers of the heart. They are most frequently found in the walls of the right atrium and the right ventricle, then the left atrium and, rarely, the left ventricle (Ellis 2006; Moorjani, Viola & Ohri 2011b; Sinnatamby 2011).
Conducting system of the heart
The cardiac cycle is co-ordinated by the conducting system of the heart. This consists of a group of specialised cardiomyocytes, namely the sinoatrial and atrioventricular nodes, which could generate spontaneous action potentials. These cardiomyocytes and the conducting fibres allow coordinated contraction between the atria and ventricles. All cardiac muscle transmits a current from cell to cell while contracting. To coordinate this contraction, the atria are insulated from the ventricles by the cardiac skeleton; and current is directed around the ventricles, down fast-response conducting fibres so that they contract in a manner that ejects blood from the apex, then out through the outflow tracts.
Sinoatrial node
The sinoatrial (SA) node is the primary pacemaker of the heart. This node is located deep to the epicardium, anterolaterally at the junction of the superior vena cava and the roof of the right atrium, near the superior end of the sulcus terminalis. The SA node generates a signal which spreads around the right atrium, via the anterior, middle and posterior intermodal tracts, and arrives at the atrioventricular node. The cells spontaneously depolarise at approximately 100 beats per minute and the rate is regulated by the autonomic nervous system. The signal also travels from the right to the left atrium, via Bachmann’s bundle, causing both atria to contract almost simultaneously. The conduction pathways within the atria are not made from specialised conduction tissue, like the Purkinje fibres found in the ventricular walls, but from cardiac muscle bundles.
The atrioventricular (AV) node is the secondary pacemaker and discharges electrical impulses (without any stimulation) at a rate of 40–60 beats per minute. It is in the posterior-inferior region of the interatrial septum. The AV node conducts the signal from the SA node to the ventricles via the AV bundle (bundle of His), which acts as a bridge between the atrial and the ventricular myocardium. The AV node is ‘slow response’, meaning that it takes longer to transmit the signal than other cardiac tissue. This delay allows the atria to fully contract and eject blood into the ventricles before the ventricles begin to contract.
The bundle of His conducts the signal through the insulating fibrous skeleton of the heart, along the membranous part of the interventricular septum, dividing into the right and left bundle branches at the junction of the membranous and muscular septum. These branches proceed downwards along the septum, towards the apex, and branch into the walls of the right and left ventricle as Purkinje fibres (sub-endocardial branches).
The right bundle branch deploys many fibres into the sub-endocardial layer of the right ventricle at the base of the medial papillary muscle of the tricuspid valve. They run within the moderator band (septomarginal trabeculation) and direct current to the anterior papillary muscle and the rest of the right ventricular wall via Purkinje fibres. The left bundle branch splits first into the anterior and posterior fascicles before these go on to direct current towards the papillary muscles and walls of the left ventricle via Purkinje fibres.
Location of the atrioventricular node within the Triangle of Koch
The location of the AV node can be triangulated by identifying landmarks within the right atrium. These three landmarks form the edges of an imaginary triangle known as the triangle of Koch, at the apex of which lies the AV node. The three boundaries are formed by:
1. The base of the septal leaflet of the tricuspid valve
2. The coronary sinus
3. The tendon of Todaro – a ridge of tissue formed at the common insertion of the Eustachian and Thebesian valves.
The AV node is found where the tendon of Todaro meets the septal leaflet of the tricuspid valve.
The bundle of His can be located at the membranous septum, just superior to the triangle of Koch, where it penetrates the muscular septum (Moorjani, Viola & Ohri 2011b; Anderson & Cook 2014).
Fibrous skeleton of the heart
The fibrous skeleton of the heart consists of four rings, each encircling a valve. It acts as an electrical insulator to both the atria and ventricles, permitting impulses through specialised conduction tissue only. It also provides attachments for valve leaflets and cusps. The orientation of the valves within the heart is such that, although they are in different chambers, their circumferences almost touch.
Heart valves
At the inlet and outlet of each ventricle there is a valve. The inlet valves lie between the atria and ventricles and are called atrioventricular valves. The outflow valves lie between the ventricles and the great arteries and are called arterial valves. Each valve has its own name, related to its appearance or location, but in the congenitally malformed or corrected heart, these names can be misleading or redundant (Anderson & Cook 2014).
The valves at the inlet must be large enough to transmit blood from the atria quickly, at the relatively low atrial systolic pressure, but robust enough to prevent high pressure blood regurgitating into the atria during ventricular systole. The valves must also be able to open and close quickly and remain competent, despite their annulus distorting as the muscle of the ventricular wall contracts. The valves at the ventricular outflow must allow smooth blood flow and not exert the shearing forces on blood cells seen in turbulent flow. The valves must then close quickly and withstand the pressure produced by elastic recoil of the great vessels.
The differing morphologies of these valves reflect the different demands placed upon them (Moorjani, Viola & Ohri 2011b; Anderson & Cook 2014; Drake, Vogl & Mitchell 2015).
Tricuspid valve
The right atrioventricular valve typically has three leaflets or ‘cusps’. This distinguishes it from the left atrioventricular valve, which has only two cusps. It is therefore given the common name – the tricuspid valve. The three cusps are the anterior, posterior and septal leaflets. The attachment of each leaflet determines the name it is given (with the anterior leaflet attaching to the anterior free wall etc.). Where the leaflets attach to the wall, the tissue is the more fibrous, insulating tissue of the cardiac skeleton. The points at which each leaflet meet on the annulus are called commissures. The free margins of the leaflets are serrated and held curved inward towards the apex of the ventricle by the chordae tendineae and their attachments to the papillary muscles. This curve means that the line of apposition between leaflets is not at the free margin, but on the atrial surface of each leaflet that they coapt. When the papillary muscles contract, the chordae tether the leaflets and prevent them from everting and leaking during ventricular systole.
When approaching surgical repair of the tricuspid valve, it is important to consider the surrounding structures. At the commissure between the anterior and septal leaflet, and halfway around the septal annulus, lies the atrioventricular node and bundle of His. From midway round the septal annulus to midway round the posterior annulus runs the coronary sinus, and from the commissure between the septal and posterior leaflets to midway round the anterior leaflet annulus, the right coronary artery runs in close proximity. The remainder of the anterior leaflet annulus is near the non-coronary cusp of the aortic valve. If the right ventricle dilates, as in right-sided heart failure, the tricuspid annulus can dilate. As the leaflets remain the same size, the coaptation areas move towards the free margin of the leaflet and, if it dilates too far, the valve leaks (Sinnatamby 2011; Anderson & Cook 2014; Harington, Mora & Wu 2014; Drake, Vogl & Mitchell 2015).
Pulmonary valve
The right arterial valve, in the normal arrangement, lies at the origin of the pulmonary trunk (main pulmonary artery). For this reason, it is called the pulmonary valve, and, in congenital malformation such as transposition of the great vessels (where the aorta arises from the right ventricle and the pulmonary trunk from the left), the name ‘pulmonary valve’ is retained by the valve at the origin of the pulmonary trunk.
The valve is a semilunar valve; it is composed of three cusps that each occupy approximately one-third of the cross-sectional area of the pulmonary trunk. The attachment of each cusp to the arterial wall is curved so that each end of the cusp is more distal in the artery and the middle of the valve forms a nadir. This curved attachment of each leaflet makes the valve annulus a crown-like structure, rising up towards the commissures and dipping down towards the basal attachment of each cusp. At the level of the commissures a ridge is visible on the inner surface of the artery; the sinotubular junction. The areas of the artery wall demarcated by the attachment of the leaflets and the sinotubular junction bulge outwards ever so slightly to form three pouches; the pulmonary sinuses.
Viewed anteriorly, there is a left, right and anterior sinus. When the valve is closed there are three zones of apposition with a central point where all three leaflets meet; thus, from above or below, the closed valve looks like a Mercedes Benz sign. The free edges of the cusps are slightly thickened, forming the ‘lenules’, and the central portion where all three cusps meet is thickened further, forming the nodule of the semilunar cusp or ‘nodule of Arantius’.
The zones of apposition, when the valve is closed, fall on the plane of the sinotubular junction so that each cusp, along with its associated sinus, forms a little cup. It is the pressure of the blood filling this cup, rising above that of the ventricle, that forces the valve closed in ventricular diastole. The opening and closing of the semilunar valves is entirely passive (Moorjani, Viola & Ohri 2011b; Sinnatamby 2011; Anderson & Cook 2014; Harington, Mora & Wu 2014; Drake, Vogl & Mitchell 2015).
Mitral valve
The left atrioventricular valve has two leaflets: the anterior (or aortic) leaflet and the posterior (or mural) leaflet. The annulus of the valve is kidney-shaped, with the anterior leaflet occupying the inner curve (approximately one-third of the annular circumference) and the posterior leaflet, the outer curve (approximately two-thirds of the annular circumference). The two leaflets have approximately the same surface area, as the anterior leaflet is longer and crosses about two-thirds of the orifice diameter before meeting the posterior leaflet. This gives the coaptation area a curved appearance from which the ‘mitral valve’ derives its name, as it is said to resemble a bishop’s mitre.
The posterior leaflet, rather than appearing as a single sheet, is usually divided into three ‘scallops’. These scallops are like miniature leaflets within the leaflet and are useful functional segments to consider when approaching surgical repair. From the anterolateral to the posteromedial commissure, they are labelled P1, P2 and P3. The anterior leaflet is a single sheet, but the corresponding coapting regions are named A1, A2 and A3. The annulus is again composed of fibrous insulating tissue but has a different variety of structures to be aware of when surgery is contemplated.
The orientation of the valve is such that the anterior leaflet has its annular base just beneath the left and non-coronary cusps of the aortic valve. From the anterolateral commissure round to the nadir of the posterior annulus runs the circumflex coronary artery, and from the nadir to the posteromedial commissure runs the coronary sinus. At the posteromedial commissure is the right fibrous trigone, where the atrioventricular node and conducting bundles run in close proximity. When the left ventricle becomes dilated (as with myocardial infarction), the papillary muscles pull away from the mitral valve towards the apex. This can prevent the valve from closing, allowing regurgitation of blood into the left atrium during ventricular systole. If the papillary muscles themselves become infarcted, they can rupture, leaving a flail segment prolapsing into the left atrium. A similar, though less dramatic, situation can arise with snapped chordae (Moorjani, Viola & Ohri 2011b; Sinnatamby 2011; Anderson & Cook 2014; Harington, Mora & Wu 2014; Timek and Fann 2014).
Aortic valve
The left arterial valve, in the normal arrangement, lies within the origin of the aorta. It is similar in structure to the pulmonary valve, with a similar mechanism of operation and similar surrounding structures and crown-shaped annulus. As with the pulmonary valve, it is named for the vessel in which it lies – the aorta. The three sinuses of the aortic valve are the left coronary sinus, the right coronary sinus and the non-coronary sinus. They are named for the coronary artery that arises from them (with, typically, no vessel arising from the non-coronary sinus). When viewed from an anterior perspective, the left coronary sinus lies posteriorly, the right coronary sinus lies anteriorly to the right, and the non-coronary sinus lies anteriorly to the left (Moorjani, Viola & Ohri 2011a, 2011b; Anderson & Cook 2014; Harington, Mora & Wu 2014; Drake, Vogl & Mitchell 2015).
The great vessels
The two arteries arising from the ventricles and bounded by arterial valves are called ‘the great arteries’ and the veins that drain into the heart are called ‘the great veins’. They have typical paths and relationships to surrounding structures that are important to know about when operating.
The superior vena cava (SVC) drains into the superior aspect of the right atrium. It is the rightmost of the vascular structures connected superiorly to the heart (leftmost when viewed from the front). Descending vertically into the heart, it is formed by the confluence of the right and left brachiocephalic (or innominate) veins, at the level of the first costal cartilage, and it enters the pericardium just before it connects to the heart. The left innominate vein crosses from the left anterior to the other superior great vessels and their branches. It is at risk of injury when identifying the superior extent of the pericardium to expose the aorta. It receives a tributary in the form of the azygous vein on its right lateral border immediately before entering the pericardium. Within the pericardium, it is covered anteriorly by serous pericardium but posteriorly it is bare and helps form the border of the pericardial sinuses (Ellis 2006; Moorjani, Viola & Ohri 2011b; Drake, Vogl & Mitchell 2015).
Inferior vena cava
The inferior vena cava (IVC) enters the pericardium immediately after penetrating the diaphragm at the level of T8. It has a very short intra-pericardial length, where it is covered anteriorly by serous pericardium, before joining the right atrium (Drake, Vogl & Mitchell 2015).
Pulmonary veins
The right and left paired superior and inferior pulmonary veins enter the posterior lateral walls of the fibrous pericardium before entering the left atrium. They are covered anteriorly by serous pericardium but posteriorly they are continuous with the parietal pericardium and, along with the SVC, IVC and the fold of serous pericardium connecting them, help form the borders of the oblique and transverse sinuses ( Moorjani, Viola & Ohri 2011b; Drake, Vogl & Mitchell 2015).
Aorta
The aorta arises from the left ventricle, between the SVC and pulmonary trunk. It travels vertically for a few centimetres before exiting the pericardium and arching backwards and to the left, at the level of T4, to travel inferiorly within the left hemithorax. Within the pericardium it is covered, together with the pulmonary trunk, in a sheath of serous pericardium. On the arch, it typically gives off three branches: first the brachiocephalic trunk (innominate artery); then the left common carotid; then the left subclavian arteries. The brachiocephalic trunk splits into the right subclavian and common carotid arteries (Ellis 2006; Moorjani, Viola & Ohri 2011b; Drake, Vogl & Mitchell 2015).
Pulmonary trunk
The pulmonary trunk arises from the right ventricle to the left of the aorta. To do so, the right ventricular outflow tract passes anterior to the left ventricular outflow tract. The pulmonary trunk is therefore pointing slightly posteriorly as it arises and travels backwards, before splitting into the left and right pulmonary arteries, beneath the arch of the aorta, that come off in the horizontal plane at almost 180 degrees from each other. They exit the pericardium superior to the pulmonary veins (Moorjani, Viola & Ohri 2011b; Sinnatamby 2011).
Conduits used for coronary bypass grafting surgery
Internal mammary artery
Also known as the internal thoracic artery, the internal mammary artery (IMA) arises from the subclavian artery. Many patients have their left internal mammary artery harvested as a conduit. However, in young patients, where total arterial revascularisation is planned, both right and left internal mammary arteries may be used. In older patient with diabetes with an osteoporotic sternum, bilateral internal mammary artery harvesting may increase the risk of sternal wound infections or dehiscence.
This artery passes inferiorly and lateral to the sternum, dividing into the superior epigastric and musculophrenic arteries. The artery supplies the anterior intercostal arteries to intercostal spaces one to six. It can be a source of major haemorrhage in chest trauma if it is punctured by a fractured rib.
Radial artery
The radial artery courses inferolaterally and is covered by the brachioradialis muscle. The artery is visible when this muscle is pulled laterally. In the distal forearm the artery lies on the anterior surface of the radius where it is covered by skin and fascia only. The strong pulsations can be felt just lateral to the tendon of flexor carpi radialis. An Allen’s test needs to be carried out on every patient prior to harvesting the radial artery. This is essential to confirm if the patient has an intact palmar arch blood flow between the ulnar and radial artery which will prevent ischaemia in the limb (Moorjani, Viola & Ohri 2011b).
Greater saphenous vein
The greater saphenous vein is a superficial vein that courses anterior to the medial malleolus, ascending on the medial aspect of the lower limb. It can be found a hand’s breadth posterior to the patella and empties into the deep femoral vein. The superficial circumflex iliac, superficial epigastric and external pudendal veins also drain into the greater saphenous vein near its termination in the groin.
Note: This vein is readily accessible for coronary artery bypass grafting. It is reversed when removed so that the valves inside the vein do not obstruct coronary blood flow.
The saphenous nerve also accompanies the vein, and injury to this nerve may cause pain or numbness and a tingling sensation along the medial border of the foot.
Short saphenous vein
The short saphenous vein is harvested when there is little choice of conduits for bypass and it is more technically challenging to harvest. It may be necessary to use this conduit when a patient has no suitable radial arteries or if they have varicosities in the greater saphenous vein distribution. The short saphenous vein arises laterally on the foot and ascends posterior to the lateral malleolus, emptying into the popliteal vein in the popliteal fossa (Moorjani, Viola & Ohri 2011b).
Introduction
The thorax describes the uppermost portion of the body’s trunk, which encloses the thoracic cavity (see Figure 4.4). It is bounded superiorly by an open bony ring formed by the superior borders of the 1st ribs and manubrium, known as the ‘thoracic inlet’ or ‘superior thoracic aperture’. Inferiorly, the inferior borders of the 11th and 12th ribs, along with the combined costal cartilages of 7th to 10th ribs and xiphisternum, make up a larger ring, closed off by the diaphragm, known as the ‘inferior thoracic aperture’ (Ellis 2006; Drake, Vogl & Mitchell 2015; Moorjani, Viola & Walker 2016).
Figure 4.4: The thoracic cage, viewed from the front. The vertebral column has been removed and the right and left clavicles added
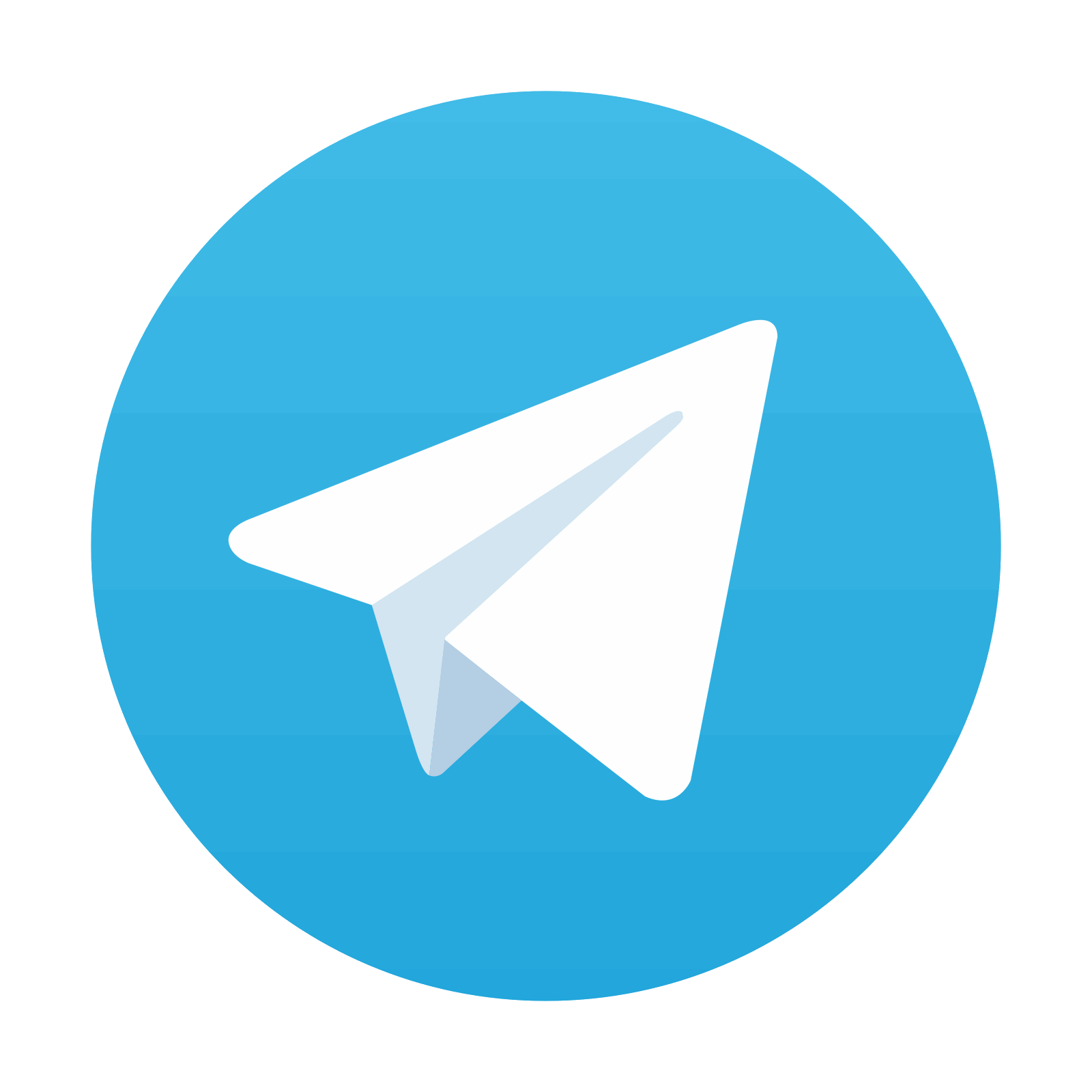
Stay updated, free articles. Join our Telegram channel

Full access? Get Clinical Tree
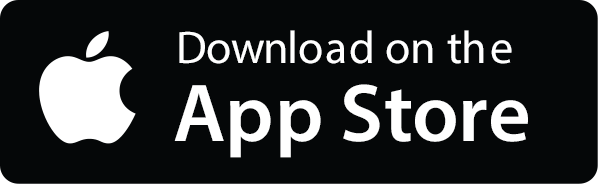
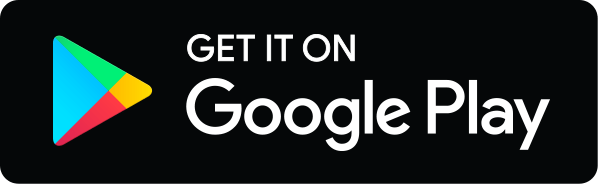