CAPWA: Computerized Arterial Pulse Wave Analysis
Daniel Duprez, MD, PhD
Jay N. Cohn, MD
Arterial Elasticity (1/Arterial Stiffness) and Blood Pressure
The clinical assessment of the arterial pressure wave, which is a powerful marker for cardiovascular disease, has for more than a century been dependent on sounds or arterial wall motion depicting the peak (systolic) and trough (diastolic) pressure.1 The guidelines for the diagnosis of arterial hypertension have changed over time, but they are still based on the two extreme points of the arterial blood pressure waveform.1 During the last decade, more emphasis has been placed on the systolic hypertension because of the growing elderly population with elevated systolic blood pressure.2 The recent blood pressure guidelines have targeted a lower pressure, such as 120/80 mm Hg, as an ideal goal of management.3
This emphasis on pressure rather than vascular health has deprived the care givers from access to information that can provide far more insight into the health of the vasculature. It is known that arterial hypertension is associated with endothelial dysfunction, one of the most powerful regulators of vascular tone, especially in the small arteries4 (Figure 13.1). Therefore, assessment of endothelial dysfunction and microvascular functional and structural abnormalities is important in the early detection of vascular changes that raise blood pressure in asymptomatic people at risk for cardiovascular disease. This diagnostic approach may be based on the morphology of the arterial pulse waveform.
Arterial stiffness and arterial elasticity (1/arterial stiffness) are important markers for vascular health and disease.4 These parameters can be obtained noninvasively using different techniques such as the measurement of the difference in arrival time of the pulse at the carotid and femoral artery (carotid-femoral pulse wave velocity) or radial artery pulse wave analysis (systolic or diastolic part). To implement one of these techniques in daily practice the following criteria need to be addressed: (1) Proof of concept—does the novel marker level differ between subjects with and without adverse outcomes? (2) Prospective validation—does the novel marker predict development of future outcomes in a prospective cohort study? (3) Incremental value—does the novel risk marker change predictive information from established, standard risk markers? (4) Clinical utility—does the novel marker change predicted risk sufficiently to change recommended therapy? (5) Clinical outcome prediction—does use of the novel marker for therapeutic guidance improve clinical outcomes, especially when tested in a randomized trial? (6) Cost-effectiveness—does use of the marker improve clinical outcomes sufficiently to justify additional costs of testing or treatment?
Although some data are available to support wider use of measures of arterial stiffness or elasticity in both the small and large arteries, critical documentation of its incremental value still requires further validation.
Radial artery pulse contour analysis is the continuous analysis of either the systolic part or the diastolic part of the radial artery and is convenient for patient and technician. In this chapter, we describe specifically the diastolic pulse contour analysis methodology.
Technique Diastolic Radial Artery Pulse Contour Analysis
Radial artery waveforms are recorded using the Hypertension Diagnostics PulseWave CR-2000 instrument. A solid-state pressure transducer array (tonometer) is placed over the radial artery of the dominant arm to record the pulse contour. Once a stable measurement is achieved, a 30-second analog tracing of the radial waveform, excluding the dicrotic notch, is digitized at 200 samples per second, with accompanying automated, oscillatory blood pressure measurement. Measures of small artery elasticity (SAE) and large artery elasticity (LAE) (change in arterial volume per change in
arterial pressure) are produced by the device. C1 (defined as LAE or capacitive compliance or proximal arterial compliance) and C2 (defined as SAE or oscillatory compliance or distal compliance) are estimated by the device from the waveform modeled as a sinusoidal function dampened by a decaying exponential.5
arterial pressure) are produced by the device. C1 (defined as LAE or capacitive compliance or proximal arterial compliance) and C2 (defined as SAE or oscillatory compliance or distal compliance) are estimated by the device from the waveform modeled as a sinusoidal function dampened by a decaying exponential.5
C2 is also an indicator for endothelial function. Gilani et al studied the effect of the inhibitor of nitric oxide release arginine NG-nitro-L-arginine-methyl ester (L-NAME).6 The magnitude of effect of L-NAME on SAE (−31.2% ± 18.4%) was significantly greater and more consistent than its effect on other vascular measurements. LAE index, brachial artery caliber, and brachial artery compliance were unchanged. Flow-mediated brachial artery dilation was blunted slightly, and aortic pulse wave velocity increased slightly but significantly because of the rise in pressure. Reduction in SAE is a sensitive marker for endothelial dysfunction and may serve as a useful marker for prehypertensive patients at risk for cardiovascular morbid events.
Validation Small Artery Elasticity and Large Artery Elasticity
A diagnostic challenge is to detect abnormal function in the vascular system before the development of symptoms or signs of cardiovascular disease. Variability in arterial elasticity may help account for race/ethnic and sex differences in cardiovascular (CVD) risk, and knowledge of arterial elasticity might improve risk stratification and help identify individuals with early vascular damage who are predisposed to future vascular events. The SAE and LAE have been studied and validated in the multiethnic study of atherosclerosis (MESA).7,8 The MESA study cohort consists of 6814 men and women who were recruited between 2000 and 2002 from six US communities and identified themselves as white, African American, Hispanic, or Chinese and were aged 45 to 84 years, free of clinically apparent CVD and never treated for cancer. The results of this multiethnic study at baseline showed that African Americans had the lowest SAE, with somewhat higher values in Hispanics. SAE values were higher in Whites and Chinese. Women in general had lower SAE than men, which was mainly because of the difference in height. LAE did not differ by race/ethnicity.
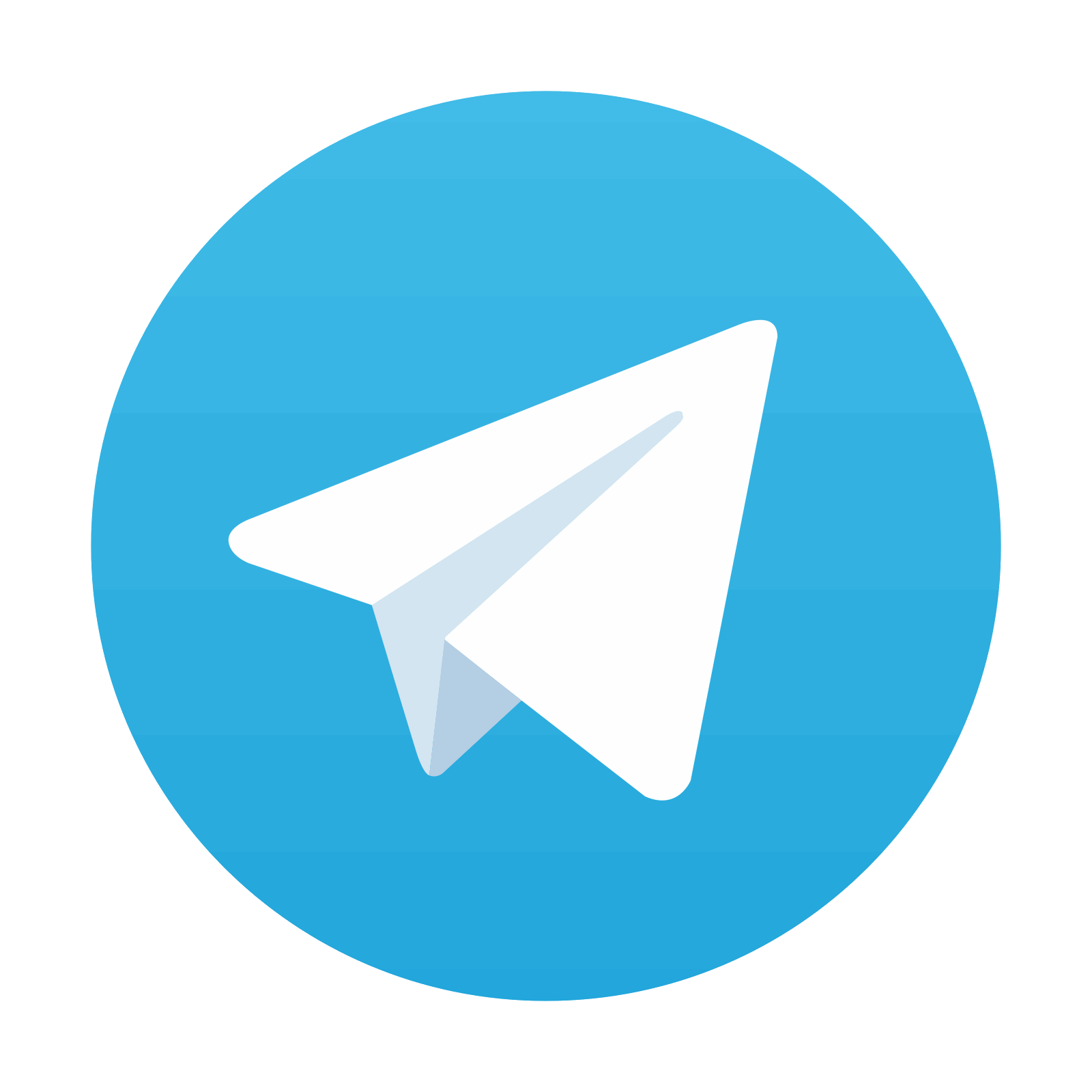
Stay updated, free articles. Join our Telegram channel

Full access? Get Clinical Tree
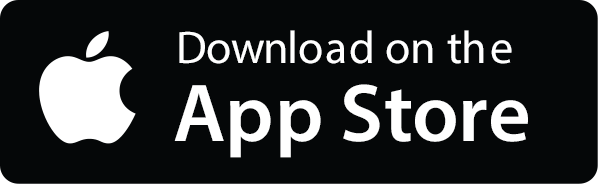
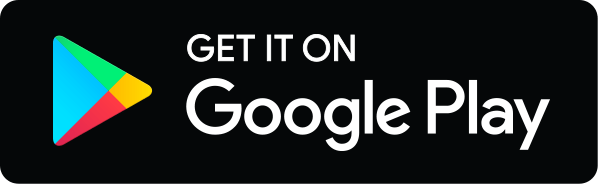