Background
Reference values for left ventricular mass (LVM) are important echocardiographic tools for the follow-up of pediatric patients with cardiac disease. Cardiac magnetic resonance (CMR) imaging is currently regarded as the gold standard. The aims of this study were to validate LVM calculated using real-time three-dimensional echocardiography (RT3DE) and to establish pediatric reference values.
Methods
For validation, 40 subjects (20 patients) consecutively underwent CMR (3-T TX Achieva, 25 cardiac phases/slice) and RT3DE (iE33 or Vivid E9, four subvolumes) in a single-center approach. The quantification of CMR data by the disk summation method as standard (mean LVM, 84.2 ± 53 g; range, 17.7–231.7 g) was compared with RT3DE. In a multicenter prospective design, 434 healthy children were investigated using standard software (LV-Analysis version 3.1).
Results
In comparison with CMR, RT3DE provided a slight overestimation of LVM of only 2.5 ± 11.3% (r = 0.990, intraclass correlation coefficient = 0.995), and there was low intraobserver (mean, 0.9 ± 7.1%; scatter, 13.2% to −15.0%; r = 0.996; intraclass correlation coefficient = 0.998) and interobserver (mean, 1.5 ± 9.3%; scatter, 17.2% to −20.1%; r = 0.993; intraclass correlation coefficient = 0.996) variability. Feasibility of the multicenter approach was 76%, resulting in 332 healthy children (median age, 10.0 years; range, 0–18 years; group I range, 0–6 years; group II range, 7–18 years) with data sets providing adequate image quality. LVM was correlated with sex (group II), age (r = 0.901), height (r = 0.881), weight (r = 0.876), and body surface area (r = 0.898). Unisex percentiles for 0 to 6 years of age and separated according to gender from 7 to 18 years of age were established. Mean calculation time for RT3DE was <3 min.
Conclusions
In children, LVM calculation presuming excellent real-time three-dimensional echocardiographic data sets is accurate, quick, and reproducible. The percentiles provided are based on a large sample size and may be useful for clinical practice.
The assessment of left ventricular (LV) mass (LVM) is a clinically important tool in patients with acquired or congenital heart disease. The combination of localized or global LV hypertrophy and dilatation has both diagnostic and prognostic implications. Morbidity and mortality in adult and pediatric patients are increased if LV preload and afterload are elevated. In this scenario, LVM is an important marker to modify treatment strategies such as drug therapy and percutaneous or surgical interventions. However, LVM can also be influenced by many physiologic factors that we do not primarily associate with a disease state, implying an expectable variation of LVM even among healthy subjects.
Imaging modalities that can reliably assess this parameter are clinically important for further treatment and prognosis. Currently, cardiac magnetic resonance (CMR) imaging is the gold standard for the assessment of LV volumes and mass because of its accuracy, reproducibility, and independence of geometric assumptions. However, CMR cannot be used routinely in all clinical settings, because it is expensive, time consuming, in some cases even contraindicated, and it may require anesthesiology in smaller children. In comparison, echocardiography is quick, less expensive, and widely available. Not infrequently, sedation must be administered to minimize diagnostic errors, although in most cases there is no need for it even if small children are investigated. Established two-dimensional techniques are lacking in accuracy to determine LVM because they rely on geometric assumptions and use equations that cannot entirely compensate for the individual shape of a chamber. Therefore, real-time three-dimensional (3D) echocardiography (RT3DE) is a promising clinical alternative that has been proven in prior studies on adults, and improvements in hardware and evaluation software have played an important role in this field in recent years. The role of LVM measurements by RT3DE in pediatric patients has been investigated less extensively, but some studies have revealed progress regarding accuracy, reproducibility, and efficiency in normal as well as abnormally shaped ventricles.
There is a need for pediatric reference values, because LVM changes during maturation. Currently, there are few valid pediatric normative data available. Many have been determined by CMR, include only small sample sizes, or are reliable for adults only. The aims of this study were to validate the accuracy and reproducibility of LVM using RT3DE compared with the gold standard of CMR using new quantification software and to establish reference values in a large cohort of healthy children.
Methods
Study Population and Design
Validation Study
We prospectively carried out consecutive investigations by CMR and RT3DE in a single-center approach to assess the reproducibility and accuracy of LVM calculation by RT3DE in a group of 40 individuals. Ten patients with LV heart disease as well as 10 with abnormally shaped left ventricles ( Table 1 ), who were referred for routine CMR because of congenital heart disease, were included, as well as 20 healthy children, who also participated in the reference-values study. This selection was intended to display wide ranges of LVM, age, and shape. All investigations were carried out with the breath held; four patients were investigated under anesthesia.
Variable | Value |
---|---|
Sex (female) | 19 |
Age (y) | 2.3–43.9 (13.8 ± 8.3) (median, 12.7) |
Height (cm) | 84.0–196.0 (151.3 ± 27.9) |
Weight (kg) | 11.0–97.0 (49.4 ± 25.3) |
BSA (m 2 ) | 0.5–2.2 (1.4 ± 0.5) |
LVM by CMR (g) | 17.7–231.7 (84.2 ± 53.0) |
LVM by RT3DE (g) | 20.0–236.7 (86.0 ± 53.7) |
Reference-Values Study
For reference values, a prospective multicenter approach including three centers was performed after standardization of the acquisition procedure and interoperator training. Quantification of data was done in a core-laboratory design at one center. All healthy individuals mentioned in this study were in sinus rhythm, and cardiac disease was ruled out by history, physical examination, and echocardiography according to standard recommendations. Young children not able to hold their breath were examined under spontaneous breathing.
For the creation of reference values for LVM with RT3DE, 434 healthy children were included from April 2011 to November 2013. Age, sex, weight, and height were measured before the examination, and body surface area (BSA) was calculated using the formula of Du Bois and Du Bois: body weight (kg) 0.425 × height (cm) 0.725 × 0.007184. The study was approved by the local ethics institutional review committee (Registration No. 20/2011) and by the representative boards of all participating centers and conformed to the principles of the Declaration of Helsinki as well as German law. Informed written consent was obtained from all participants and/or their legal guardians before participation.
RT3DE
RT3DE was performed using iE33 (X7-2 or X5-1 transducer; Philips Medical Systems, Best, The Netherlands) or Vivid E9 (V4 transducer; GE Medical Systems, Milwaukee, WI) machines. Three real-time 3D echocardiographic volumes were acquired from an apical view with the LV apex next to the transducer, including the entire LV portion in the two-dimensional planning view using four subvolumes at 90° to 110° within a single end-expiratory breath-hold if possible to obtain one full-volume data set with a stable transducer position based on standard recommendations. The temporal resolution was between 17 and 65 volumes/sec, depending on angulation width, depth, transducer, age, and heart rate.
Evaluation of Real-Time 3D Echocardiographic Data
The real-time 3D echocardiographic volume with the best image quality was imported into the Image Arena platform (version 4.6, build 4.6.2.12; TomTec, Unterschleißheim, Germany) and analyzed using 4D LV-Analysis version 3.1 (build 3.1.0.258661; TomTec). Briefly, after definition of end-diastolic and end-systolic frames, the full-volume data set was adjusted using two-chamber, three-chamber, and four-chamber views, aligning the center of the mitral valve and apex of the left ventricle. After a first contour-tracking process, endocardial contour finding was manually revised if necessary, and the outer layer of the epicardium was manually determined ( Figure 1 ). The myocardial volume was calculated from a connection between the epicardial and endocardial surface model mesh in systole multiplied by 1.053 g/mL, corresponding to the density of the myocardium.

CMR Imaging
Volumetric measurements were performed using a clinical 3.0-T whole-body magnetic resonance imaging system (Achieva 3.0T TX; Philips Medical Systems) equipped with parallel radiofrequency signal transmission technology to enhance image uniformity (maximum gradient performance, 80 mT/m; slew rate, 200 T/m/sec) using vector electrocardiography and respiratory motion gating. A 32-element phased-array receive-only surface coil was used for signal detection. To assess the LV and right ventricular volumes, a stack of 15 to 21 short-axis slices was acquired by applying a segmented multislice, multiphase vector electrocardiographically triggered steady-state free precession gradient-echo sequence (repetition time, 2.7 msec; echo time; 1.35 msec; excitation angle, 40°; slice thickness, 5–6 mm; no slice gap; matrix size, 160 × 240; field of view, 384 mm; in-plane resolution, 1.6 × 1.6 mm; 25 cardiac phases under short breath-holding periods of <12 sec in duration).
Evaluation of CMR Data
Quantification of the volumetric CMR data was performed offline on a workstation using the threshold-based HDZ MR-Tools software package (HDZ, Bad Oeynhausen, Germany). LV muscle mass was calculated by summing the muscle areas and multiplying this by the slice thickness for all appropriate slices. Intracavitary trabeculations were excluded from the calculation of muscle mass to obtain an evaluation strategy comparable with RT3DE.
Statistical Analysis
Real-time 3D echocardiographic and CMR data, respectively, were quantified by a different single expert blinded to the results of the other. For measurements of interobserver variability, another investigator was introduced after intensive interoperator training, again blinded to the results of the first investigator. Reproducibility was previously assessed extensively using the CMR HDZ MR-Tools software package. Intraobserver comparisons for RT3DE were performed in a second approach blinded to the results of the first quantification, >2 weeks apart. Analysis of Bland-Altman plotting was performed to quantify the agreement between CMR and RT3DE as well as intra- and interobserver variability using the percentage of difference of the investigations as a measure to address the problem of high variety of masses. The latter were also expressed by the intraclass correlation coefficient (ICC).
Correlation analysis was undertaken using linear regression analysis and Pearson correlation using SPSS version 21 (SPSS, Inc, Chicago, IL). Descriptive statistics were obtained for all relevant continuous variables. Normal distribution was tested using the Kolmogorov-Smirnoff or Shapiro-Wilk test, depending on the sample size. The significance of the differences observed was tested using a two-sided paired Students t test. P values ≤.05 were considered statistically significant. For differences in homogeneity and/or the absence of normal distribution, the Mann-Whitney test was used as a nonparametric test. Reference centile curves were computed using Cole’s LMS method (lambda for the skew, mu for the median, and sigma for the coefficient of variation) in LMS version 1.27 software (Institute of Child Health, London, United Kingdom).
Results
Validation Study
All CMR and real-time 3D echocardiographic scans were completed successfully and could be included in the study. CMR evaluation of muscle mass provided a spectrum of 17.7 to 231.7 g (mean, 84.2 ± 53 g; Table 1 ). Image analysis for RT3DE required a mean evaluation time of <3 min for the investigator after >10 data sets of training.
Accuracy and Reproducibility of CMR versus RT3DE
Comparison of the methods showed good agreement, with only a minimal overestimation of LVM using RT3DE at 2.5 ± 11.3% (r = 0.990, ICC = 0.995; Figures 2A and 2B ) and moderate scatter (20.1% to −25.1%). Intraobserver variability was low at only 0.9 ± 7.1% (r = 0.996, ICC = 0.998), with acceptable scatter (13.2% to −15.0%; Figures 3A and 3B ). Interobserver variability resulted in comparably low mean differences of 1.5 ± 9.3% (r = 0.993; ICC = 0.996; scatter, 17.2% to −20.1%; Figures 3C and 3D ).


Reference-Values Study
Feasibility
Three hundred thirty-two of the 434 data sets provided adequate image quality and therefore could be included in the study, which resulted in feasibility of 76%. The dropout of 102 data sets was due mainly to the miss of major parts of the myocardium (51 data sets; missing apex in 89%, other regions missed in 11%); too strong reflections by the pericardium, limiting border differentiation (five data sets); breathing artifacts, especially in smaller children (14 data sets); or a frame rate that was too low (32 data sets).
Growth- and Sex-Dependent Interrelations of LVM
The entire group of healthy children was investigated concerning correlations ( Table 2 ).
Variable | All | Female | Male | Aged 0–6 y | Female, aged 7–18 y | Male, aged 7–18 y |
---|---|---|---|---|---|---|
( n = 332) | ( n = 162) | ( n = 170) | ( n = 106) | ( n = 110) | ( n = 116) | |
Age (y) | 0–18 (9.6 ± 5.2) (median, 10) | 0–18 (9.4 ± 4.9) (median, 9) | 0–18 (9.8 ± 5.4) (median, 10) | 0–6 (3.4 ± 2.0) (median, 4) | 7–18 (12.1 ± 3.4) (median, 12) | 7–18 (12.9 ± 3.2) (median, 13) |
Height (cm) | 47–194 (136 ± 33) | 47–181 (134 ± 31) | 50–194 (138 ± 36) | 47–127 (96 ± 20) | 114–181 (150 ± 17) | 118–194 (158 ± 20) |
Weight (kg) | 2.6–93 (36.3 ± 20) | 2.57–73 (34.0 ± 17.6) | 3.1–93 (38.5 ± 22.0) | 2.6–26.4 (15.0 ± 5.3) | 19.1–73 (42.8 ± 14.2) | 20.3–93 (49.6 ± 17.4) |
BSA (m 2 ) | 0.19–2.15 (1.16 ± 0.47) | 0.19–1.9 (1.12 ± 0.42) | 0.2–2.15 (1.2 ± 0.51) | 0.19–1.0 (0.62 ± 0.2) | 0.8–1.9 (1.34 ± 0.29) | 0.82–2.2 (1.5 ± 0.35) |
Heart rate (beats/min) | 50–160 (86 ± 20) | 51–160 (87 ± 21) | 50–147 (85 ± 20) | 66–160 (105 ± 22) | 51–109 (78 ± 12) | 50–110 (76 ± 12) |
Volume rate (vol/sec) | 17–65 (28.3 ± 7.1) | 17–61 (28.1 ± 7.1) | 17–65 (28.4 ± 7.2) | 20–61 (30.5 ± 8.7) | 17–44 (26.4 ± 5.2) | 17–65 (27.9 ± 6.6) |
LVM (g) | 6.2–197 (88.3 ± 44.3) | 6.2–182.8 (83.3 ± 39.2) | 7.8–196.7 (94 ± 48.2) | 6.2–75.9 (40.8 ± 16.8) | 38.7–182.8 (101.5 ± 31.3) ∗ | 53.7–196.7 (118.2 ± 36.3) |
LVM iBSA (g/m 2 ) | 33.2–122.9 (73.1 ± 15.7) | 33.2–122.9 (71.5 ± 15.2) | 34.4–115.5 (74.8 ± 16.0) | 33.2–90.4 (63.4 ± 13.5) | 44.2–122.9 (75.4 ± 14.9) ∗ | 50.7–115.5 (79.6 ± 14.2) |
LVM iHeight (g/cm) | 0.13–1.11 (0.61 ± 0.2) | 0.13–1.11 (0.58 ± 0.19) | 0.16–1.10 (0.63 ± 0.21) | 0.13–0.63 (0.41 ± 0.12) | 0.31–1.11 (0.67 ± 0.16) ∗ | 0.42–1.10 (0.74 ± 0.16) |
LVM iWeight (g/kg) | 1.30–4.61 (2.54 ± 0.54) | 1.43–4.25 (2.53 ± 0.52) | 1.30–4.61 (2.55 ± 0.56) | 1.42–3.86 (2.71 ± 0.51) | 1.43–4.25 (2.45 ± 0.54) | 1.30–4.61 (2.48 ± 0.54) |
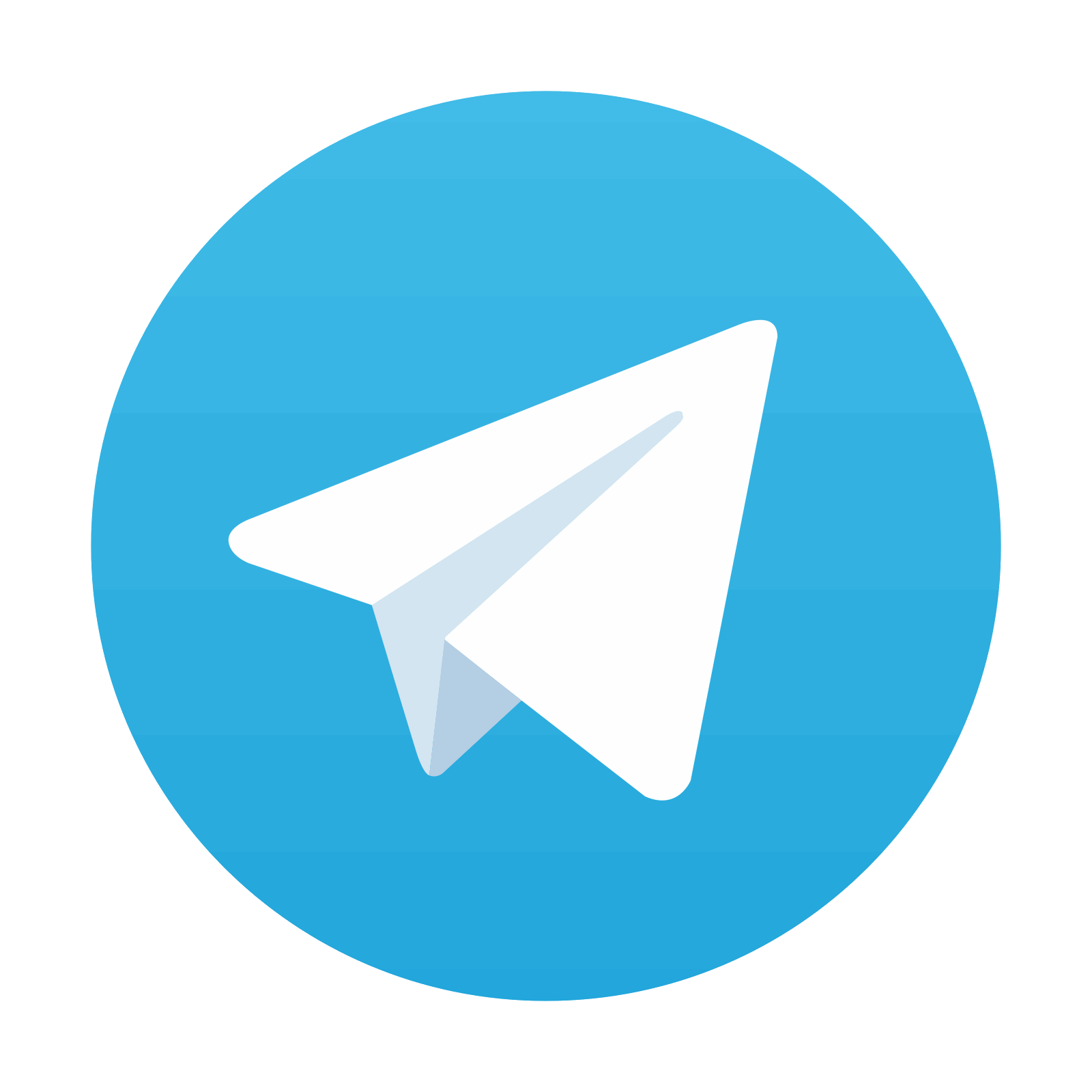
Stay updated, free articles. Join our Telegram channel

Full access? Get Clinical Tree
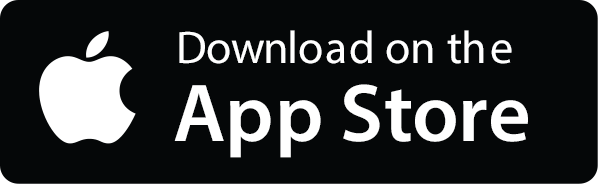
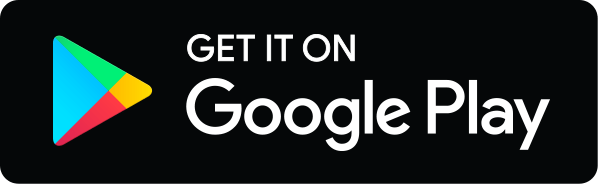
