Fig. 12.1
Neural regulation of bronchial tone[2]. eNANC excitatory NANC, Ach acetylcholine. (Ref : Cazzola M, Pharmacol Rev 2012, 64:450–504.)
12.1.1 Cholinergic Pathway
Acetylcholine (ACh) is the “classic” neurotransmitter of the parasympathetic nervous system at both the level of ganglionic transmission and the neuroeffector junctions. ACh acts via activation of muscarinic receptors (mAChRs) that belong to the large seven-transmembrane family of G protein-coupled receptors (GPCRs). Five different subtypes of mAChRs have been identified by molecular biological techniques (M1–M5), but so far, a sufficient pharmacological and functional characterization has been provided for only four of them (M1–M4) [3]. The mAChRs are expressed in almost every cell type of the airway and lung tissue, including airway and vascular smooth muscle, different glandular and surface epithelial cells, endothelial cells, and various inflammatory cells. In humans, M1 mAChRs seem to be expressed particularly in peripheral lung tissue and in the alveolar wall, but they have not been detected in larger airways, where M2 and M3 mAChRs represent the major population of mAChRs. Under “physiological” conditions, the ASM contraction induced by ACh is mediated primarily via the M3 subtype. M2 mAChRs couple to adenylyl cyclase via Gi in an inhibitory manner. They functionally oppose the β-AR-mediated increase in cAMP, leading to attenuation of β-AR-induced relaxation of ASM and prevent activation of Ca2-dependent K (KCa) channels [2, 3].
12.1.2 Adrenergic Pathway
β-ARs are present in high concentration in lung tissue, and autoradiographic mapping and in situ hybridization studies show that they are localized to several cell types. β-ARs are subdivided into three types: β1, β2, and β3. They are members of the seven-transmembrane spanning family of GPCRs related to bacteriorhodopsin and are composed of 413 amino acid residues. There is a 65–70 % homology between β1/ β3– and β2-ARs. Binding studies show that approximately 70 % of pulmonary β-ARs are of the β2-AR subtype. These receptors are localized to ASM (3–4/104 per cell), epithelium, vascular smooth muscle, and submucosal glands [4], whereas β1-ARs in the lung are confined to glands and alveoli. There is a uniform distribution of β-ARs on the alveolar wall with a 2:1 ratio of β1/ β2-ARs. β2-AR density increases with increasing airway generation, and high levels are found in the alveolar region. Computed tomography scanning has confirmed that β2-AR distribution is greater for small rather than large airways. β2-ARs are also expressed on many proinflammatory and immune cells, including mast cells, macrophages, neutrophils, lymphocytes, eosinophils, epithelial and endothelial cells, and type I and type II alveolar cells [2].
GPCRs are dynamic proteins that switch between an inactive state and an active conformation that can engage G proteins (Fig. 12.2). Persistent activation of a GPCR is achieved through the binding of both an agonist and a G protein at opposite ends of the receptors relative to the lipid bilayer, where the combined binding interactions reduce the energy barriers to the formation of the active state. β2-ARs are coupled to Gs, where stimulation by a β2-AR agonist activates adenylyl cyclase and increases cAMP levels. cAMP increases protein kinase A (PKA) activity, which phosphorylates downstream protein modulators. The overall activation of this signal transduction pathway can lead to the inhibition of phosphoinositol hydrolysis, a fall in intracellular Ca2 levels, and the activation of large-conductance K channels. The hyperpolarization of airway smooth muscle as a result of opening K channels can lead to relaxation of airway smooth muscle. β2-AR stimulation produces airway relaxation, but prolonged β2-AR activation leads to a decrease in receptor responsiveness that differs depending on the cell type but is more readily demonstrable in inflammatory cells than ASM [5].


Fig. 12.2
Main intracellular pathways involved in the bronchomotor tone regulation [2]
PLC phospholipase C-, PIP2 phosphatidylinositol 4,5-bisphosphate, IP3 inositol 1,4,5-trisphosphate, DAG diacylglycerol, SR sarcoplasmic reticulum, MLCK myosin light-chain kinase, MLC myosin light chain, MLC-P myosin light-chain phosphorylation, CPI-17 C kinase-potentiated phosphatase inhibitor, Rho kinase Rho-associated serine/threonine kinase, AC adenylyl cyclase(Ref : Cazzola M, Pharmacol Rev 2012, 64:450–504.)
12.1.3 Non-adrenergic Non-cholinergic Pathway
Autonomic neural control of ASM tone cannot be fully explained by the functions of the cholinergic and adrenergic nervous systems alone [6]. For example, striking changes in ASM tone can be induced even in the presence of an anticholinergic agent (atropine) and a β-AR antagonist (propranolol). There is substantial evidence of contractile and relaxant NANC smooth muscle responses in mammalian airways. In fact, inhibitory NANC (iNANC) innervation is considered the primary neural mechanism mediating ASM relaxation. iNANC relaxation is thought to be generated by a combined effect of vasoactive intestinal peptide (VIP), VIP structure-related peptides (e.g., peptide histidine methionine), and nitric oxide (NO). Indeed, VIP, VIP-like peptides, and NO synthase have been identified in the parasympathetic ganglia and nerve fibers innervating ASM. Furthermore, endogenously released VIP-like and NO-like substances can attenuate ASM contraction induced by ACh. However, the precise anatomical pathways of iNANC innervation of human ASM are not clear. There is also a potent excitatory effect on the ASM involving the “efferent” functions of a specific subtype of bronchopulmonary sensory nerves containing tachykinins (e.g., substance P and neurokinin A) in guinea pig airways, although this is less evident in human airways. When these afferent endings are activated, the impulses trigger the release of tachykinins either locally or propagating antidromically to other peripheral branches via the axonal ramifications. These sensory neuropeptides can activate neurokinin-1 and -2 receptors located on the ASM membrane and produce intense and sustained bronchoconstriction [2, 7].
12.2 Muscarinic Acetylcholine Receptor Antagonists
12.2.1 History
Inhaled mAChR antagonists have been used as treatments for respiratory diseases for centuries. The smoking of plant alkaloids was recommended as a therapy for asthma in the literature of Ayurvedic medicine as early as the seventeenth century. Atropa belladonna and Datura stramonium are rich in anticholinergic alkaloids such as atropine and stramonium [8]. Unfortunately, atropine, which is a tertiary ammonium compound, is well absorbed into the systemic circulation and penetrates the blood–brain barrier. As a result, it has multiple systemic side effects that limit its clinical usefulness. However, a renewed interest in anticholinergic drugs as therapy for respiratory diseases has been sparked by the development of safe yet effective quaternary anticholinergic compounds (Fig. 12.3). Chemical modifications of the atropine molecule, in particular by making its nitrogen atom pentavalent, have yielded a number of synthetic congeners that are very poorly absorbed from mucosae and cross the blood–brain barrier with difficulty. When given by inhalation, these agents are as effective as atropine at improving lung function but longer acting and much less prone to side effects [9].


Fig. 12.3
Milestone development of β2-AR agonists (a), antimuscarinics (b), and xanthines (c)(Ref : Cazzola M, Pharmacol Rev 2012, 64:450–504.) [2]
12.2.2 Short-Acting Muscarinic Acetylcholine Receptor Antagonists
As well as atropine methonitrate, ipratropium bromide and oxitropium bromide are effective short-acting quaternary anticholinergic drugs that have been used in the treatment of respiratory diseases. Their duration of action is approximately 6–8 h, but compared with SABAs, they have a slower onset of action, although probably a longer duration of action [9].
12.2.2.1 Atropine Methonitrate
Atropine methonitrate, a quaternary ammonium congener of atropine, seems to be a more potent bronchodilator agent than its parent compound, atropine sulfate. In patients with asthma, atropine methonitrate produces a peak bronchodilator effect similar to that of albuterol, both administered in doses that produced close to maximum bronchodilation for the drug concerned, but the effect of atropine is more prolonged, the response being significantly greater at 4 and 6 h than with albuterol [10].
12.2.2.2 Ipratropium Bromide
Ipratropium bromide is unlike atropine; it has low lipid solubility and does not pass the blood–brain barrier. Ipratropium bromide is poorly absorbed by the oral and nasal mucosa, and the swallowed drug is poorly absorbed from the gastrointestinal tract. It is a nonselective antagonist of M1, M2, and M3 mAChRs. Serum ipratropium bromide concentrations are extremely low after inhalation of the drug, peak serum concentrations being achieved at approximately 3 h after administration. The elimination half-life is 3.2–3.8 h, and ipratropium metabolites have little or no anticholinergic activity. Ipratropium bromide starts to act within 15–30 min, but maximal bronchodilation may take up to 90 min in patients with COPD. The duration of action is approximately 6 h, so in comparison with β2-AR agonists, its broncholytic effect is slower in onset and probably longer in duration. On the basis of the duration of action, ipratropium bromide is given four times daily, and the maximum number of doses (40 μg) per day should not exceed 12 [11].
12.2.2.3 Oxitropium Bromide
Oxitropium bromide is another quaternary anticholinergic compound but is based on the scopolamine molecule instead of atropine. The peak bronchodilation of oxitropium bromide may take 60–90 min, and its duration is 5–8 h. Oxitropium bromide is considered to have twice the strength of ipratropium bromide per dose. In patients with severe COPD, the FEV1 and forced vital capacity (FVC) plateau attained at a total cumulative dose of 600 μg of oxitropium bromide was slightly higher than the plateau achieved with 280 μg of ipratropium bromide [12].
12.2.3 Long-Acting Muscarinic Acetylcholine Receptor Antagonists
12.2.3.1 Tiotropium Bromide
Tiotropium bromide is a once-daily, long-acting mAChR antagonist (LAMA) with high potency and kinetic selectivity at the mAChRs. It displays a 6- to 20-fold higher affinity for mAChRs than does ipratropium bromide. Although tiotropium bromide binds to all three mAChRs, it dissociates much faster from the M2 mAChRs, which results in a more selective antagonist action for M1 and M3 mAChR subtypes. Its prolonged pharmacologic activity is the result of its slow dissociation from M1 and M3 mAChRs. The half-life of the tiotropium bromide M3 mAChR complex is approximately 35 h, compared with 0.3 h for ipratropium bromide. The mechanism allowing for the long residency of tiotropium bromide at M3 mAChRs is not completely known. The increased duration of binding at the M3 mAChRs results in prolonged improvement in lung function, allowing a once-daily dose compared with the three to four doses per day previously necessary with ipratropium bromide. Tiotropium bromide is rapidly absorbed into the circulation with a peak plasma concentration within 5 min followed by a rapid fall within an hour to a steady state and a terminal half-life of 5–6 days that is independent of dose. The peak onset of bronchodilation with tiotropium bromide occurs between 1 and 3 h with improvements in FEV1 for more than 24 h [13].
12.2.3.2 Glycopyrronium Bromide
Glycopyrronium bromide is a mAChR antagonist that has a dissociation half-life from the human M3 mAChR that is significantly shorter than tiotropium bromide or aclidinium bromide. In line with this finding, in isolated human bronchi, glycopyrronium bromide elicits a duration of action intermediate between that produced by tiotropium bromide and that of ipratropium bromide. Clinical studies have shown that glycopyrronium bromide has a fast onset of effect that is sustained over 24 h, although another recent study with nebulized glycopyrronium bromide shows that this was probably shorter acting, at least at doses below 50 μg. The safety and efficacy of glycopyrronium bromide have been documented in patients with moderate-to-severe COPD. Glycopyrronium bromide is well tolerated at doses of up to 100 μg in this patient population [14].
12.2.3.3 Aclidinium Bromide
Preclinical studies have demonstrated that aclidinium bromide exhibits M3/M2 mAChR kinetic selectivity. This mAChR antagonist dissociates from humanM3 mAChRs at a rate that was similar to that of ipratropium bromide and 2.6 times faster than that of tiotropium bromide. On the contrary, it dissociates from the same receptors slightly faster than tiotropium bromide and, in line with these findings, is equivalent to ipratropium bromide for speed of onset but with a longer duration. However, in human isolated bronchi, aclidinium bromide has a faster onset and shorter duration of action than tiotropium bromide [15]. Aclidinium bromide has the advantage of rapid hydrolytic inactivation once absorbed into the plasma, thereby enhancing its safety profile.
12.2.3.4 Umeclidinium Bromide
This agent umeclidinium is a novel high-affinity-specific mAChR antagonist. It has similar affinity to the subtypes of muscarinic receptors M1–M5. In the airways, it is a potent agent that demonstrates slow functional reversibility at cloned humanM3 mAChRs and at endogenous mAChR in isolated human bronchus. The competitive and reversible nature of antagonism was shown with human and animal origin receptors and isolated organ preparations. In preclinical in vitro as well as in vivo studies, prevention of methacholine- and acetylcholine-induced bronchoconstrictive effects was dose dependent and lasted longer than 24 h. The clinical relevance of these findings is unknown. The bronchodilation following inhalation of umeclidinium is predominantly a site-specific effect [16].
12.2.4 Novel Long-Acting Muscarinic Acetylcholine Receptor Antagonists
Several other mAChR antagonists are also under development. Unfortunately, the available public information on these bronchodilators is still limited. New agents like CHF 5407, inhaled trospium (ALKS27), and PF-4522971 are under development in 2016 now [2].
12.2.5 Oral Muscarinic Acetylcholine Receptor Antagonists
It is believed that oral anticholinergics are not a treatment option for COPD because of unacceptable side effects [11], whereas inhaled anticholinergics have virtually no systemic absorption. Mepenzolate bromide is one of the oral anticholinergics but has high affinity to M3 muscarinic acetylcholine receptor and is under redevelopment of COPD.
12.2.6 Side Effects
Anticholinergic drugs are poorly absorbed which limits the troublesome systemic effects seen with atropine. But all of the currently approved inhaled mAChR antagonists have a very wide therapeutic margin and are very well tolerated, in part because they are very poorly absorbed after inhalation. However, if any of these agents makes inadvertent contact with the eye, they can cause papillary dilation.
In older men, who may have prostatic hyperplasia, mAChR antagonists should be used with caution because they can cause urinary retention. Men using both short- and long-acting inhaled mAChR antagonists had a significantly higher risk of acute urinary retention compared with nonusers. Patients with moderate-to-severe renal impairment (creatinine clearance of < 50 ml/min) under treatment with tiotropium bromide should be closely monitored, because tiotropium bromide is predominantly excreted by the kidneys through active secretion.
Paradoxical bronchoconstriction to ipratropium bromide has been reported in humans. It is possible that this results from blockade of prejunctional M2 mAChRs on airway cholinergic nerves, which normally inhibit ACh release; when the drug is given by nebulizer, however, it is largely explained by the hypotonicity of the nebulizer solution. Paradoxical bronchoconstriction may also occur with other mAChR antagonists.
Concerns have been raised about possible associations of mAChR antagonists with cardiovascular morbidity and mortality. However, the results of the UPLIFT trial and a robust and extensive analysis of more than 19,000 patients participating in placebo-controlled clinical trials with tiotropium bromide indicate that there is no real increased risk for death or cardiovascular morbidity during treatment with this inhaled anticholinergic agent in patients with COPD [13, 17, 18].
12.3 β-Adrenergic Receptor Agonists
12.3.1 History
In traditional Chinese medicine, the botanical ma huang (the plant Ephedra equisetina), from which the active material, an alkaloid identified as ephedrine, is extracted, was used for more than 2000 years for the short-term treatment of respiratory symptoms. Beginning at the turn of the last century, the nonselective α-AR and β-AR agonist epinephrine was introduced into clinical practice and administered by the subcutaneous route for the treatment of acute asthma [19]. In the 1940s, the nonselective β-AR agonist isoproterenol was introduced for the treatment of airway disease and became the standard-of-care bronchodilator, although its use was complicated by adverse effects that were due to activation of the β1-AR in the heart, which elicits tachycardia and predisposes patients to cardiac dysrhythmias. Metaproterenol, a noncatechol resorcinol derivative of isoproterenol, was subsequently developed in the early 1960s. It was an effective bronchodilator when inhaled but also did not discriminate between β1– and β2-ARs and thus produced cardiac side effects [20].
The noncatecholamine β2-AR agonists such as fenoterol, albuterol, and terbutaline differ in their substitutions in the amine group and benzene ring. These structural modifications, conferring resistance to metabolism by COMT, result in a longer half-life and also reduce their potency for β1-ARs, making them relatively more selective for β2-ARs (Fig. 12.3). A major limitation of the β2-AR agonists in use during the 1960s and 1970s was their short duration of action, typically 4 to 6 h. Therefore, the next advance in the development of β2-AR agonists was the development of the long-acting drugs salmeterol and formoterol, the duration of action of which is approximately 12 h, which made their use for maintenance treatment. A pure R-isomer of albuterol, levalbuterol, and the R,R-enantiomer of formoterol, arformoterol, have been developed. It is claimed that they have a better safety profile than the racemic mixture because they do not have the S-enantiomer, which, at least for (S)-albuterol, is now known to have unwanted effects in the lung. At present, several once-a-day ultra-long-acting β2-AR agonists are in different stages of clinical development [2].
12.3.2 Short-Acting Agents
The principal action of β2-agonists is to relax airway smooth muscle by stimulating β2-AR, which increases cyclic AMP and produces functional antagonism to bronchoconstriction. The bronchodilator effects of short-acting β2-agonists usually wear off within 4–6 h. Regular and as-needed use of short-acting β-agonists improve FEV1 and symptoms. There is little difference in the time course of fenoterol, albuterol, and terbutaline, although there is some evidence that fenoterol might have a slightly longer duration of action. The use of high doses of short-acting β2-agonists on an as-needed basis in patients already treated with long-acting bronchodilators is not supported by evidence, may be limited by side effects, and cannot be recommended. For single-dose, as-needed use in COPD, there appears to be no advantage in using levalbuterol over conventional bronchodilators [1, 21].
12.3.3 Long-Acting β2-Adrenergic Receptor Agonists
Long-acting inhaled β2-AR agonists show duration of action of 12 or more hours [22]. The duration of action of β2-AR agonists in the human bronchus is in the following order: salmeterol > > formoterol > albuterol > terbutaline > fenoterol. Formoterol and salmeterol significantly improve FEV1 and lung volumes, dyspnea, health-related quality of life, and exacerbation rate, but have no effect on mortality and rate of decline of lung function. A systematic review of trials of salmeterol and formoterol showed a significant reduction in the numbers of patients requiring treatment for exacerbations and the number requiring hospitalization [23, 24]. Salmeterol reduces the rate of hospitalization. Although formoterol and salmeterol are both potent and effective β2-AR agonists, their different chemical structures confer markedly different pharmacological characteristics.
12.3.3.1 Formoterol
Formoterol is a full agonist of β2-ARs and has been shown to provide a rapid-onset bronchodilating effect that occurs within minutes after inhalation. A number of studies have shown a comparable clinical effect of formoterol compared with the SABAs albuterol and terbutaline in stable patients with asthma [2]. But an oral formulation of formoterol did not seem to offer a clear advantage to albuterol. In effect, formoterol has a long duration of action when given by inhalation but not when given orally. A dose–response comparison of formoterol in patients with asthma suggested that after inhalation and 50 times more potent after oral administration [25].
12.3.3.2 Salmeterol
Salmeterol is a drug resulting from a specific research program designed to achieve prolonged duration of action by molecular modification of albuterol. Salmeterol is a partial agonist with ~60 and 85 % of the efficacy of isoprenaline, respectively. In contrast to its effects on β2-ARs, at cardiac β1-ARs, salmeterol is >10,000-fold weaker than isoprenaline and has a very low efficacy (4 %). The onset of action of salmeterol on ASM is slower than that of other β 2-AR agonists, such as albuterol and formoterol, but it seems to be inherently long acting, in that its effects are independent of concentration as a result of exosite binding, whereas albuterol, fenoterol, and formoterol have shorter durations of action, but this can be prolonged by increasing the concentration of the β2-AR agonist applied to the tissue [26].
12.3.4 Ultra-Long-Acting β2-Adrenergic Receptor Agonists
A variety of β2-AR agonists with longer half-lives are currently in development, with the hope of achieving once-daily dosing. These agents include indacaterol, olodaterol, vilanterol, carmoterol, PF-610355, and AZD-3199, the structure of which has not yet been disclosed.
12.3.4.1 Indacaterol
Indacaterol is the first ultra-long-acting β2-agonist approved for the treatment of COPD that allows for once-daily administration. It is rapidly acting, with an onset of action in 5 min, like salbutamol and formoterol but with a sustained bronchodilator effect, that lasts for 24 h, like tiotropium. In long-term clinical studies in patients with moderate-to-severe COPD, once-daily indacaterol improved lung function significantly more than placebo, and improvements were significantly greater than twice-daily formoterol and noninferior to once-daily tiotropium bromide. Indacaterol was well tolerated at all doses and with a good overall safety profile. These findings suggest that indacaterol can be a first choice drug in the treatment of the patient with mild/moderate stable COPD [27].
12.3.4.2 Olodaterol
Olodaterol is a new long-acting β2-agonist for COPD. Olodaterol statistically significantly improved lung function in people with moderate to very severe COPD compared with placebo over 24 weeks and was not statistically significantly different from formoterol. Although olodaterol appears to improve lung function as well as formoterol, little evidence is available comparing it directly with other LABAs and LAMAs for COPD, particularly in terms of patient-oriented outcomes such as exacerbations, breathlessness, and quality of life. Olodaterol was often coadministered with tiotropium and ICS and only limited data support its use alone [28].
12.3.4.3 Vilanterol
Vilanterol is a potent, selective β2-AR agonist in human functional cellular assays. Vilanterol produced rapid bronchodilation in patients with COPD that was maintained over 24 h at all doses. After dosing with vilanterol, there were no serious adverse events or withdrawals due to adverse events. Vilanterol in patients with COPD significantly improved FEV1 in a dose-dependent manner. Clinically relevant treatment differences of >130 ml in mean FEV1 were observed. Clinical doses of vilanterol were associated with a low incidence of treatment-related side effects. Vilanterol was often co-administered with umeclidinium and ICS, and only limited data support its use alone [29].
12.3.4.4 Carmoterol
Carmoterol has been demonstrated to be a highly potent and selective β2-AR agonist. Carmoterol has a similar onset of action compared with albuterol and formoterol and a faster onset of action compared with salmeterol. The duration of tracheal smooth muscle relaxation is longer for carmoterol compared with both formoterol and salmeterol. In COPD, a single use of carmoterol had an effect on 24 h trough FEV1 that was better than that of two uses of salmeterol given 12 h apart. After a 2-week treatment period, once-daily doses of 2 and 4 μg of carmoterol resulted in placebo-adjusted improvements compared with baseline in trough FEV1 of 94 and 112 ml [30].
12.3.4.5 LAS100977, PF-610355, and AZD-3199
Abediterol (LAS100977), PF-610355, and AZD-3199 are novel, selective, potent, once-daily LABA in development for treatment of asthma and chronic obstructive pulmonary disease.
12.3.5 Intravenous β2-Adrenergic Receptor Agonists
An interesting new option is the development of a β2-AR agonist to be administered intravenously. Bedoradrine (MN-221) is a novel, highly selective β2-adrenoceptor agonist under development for the treatment of acute exacerbation of asthma and COPD. A preliminary small trial showed that bedoradrine added to standard therapy for severe acute asthma exacerbations was safe and provided additional clinical benefit [31].
In a small group of patients with COPD, bedoradrine seemed to improve lung function at all dose levels and reached statistical significance at both 600 and 1200 μg compared with placebo [32].
12.3.6 Transdermal β2-Adrenergic Receptor Agonists
Tulobuterol is the first bronchodilator to be available as a transdermal patch. This drug delivery system ensures that the time at which the peak drug concentration in the blood is reached coincides with the morning dip in respiratory function. The use of the patch also prevents excessive increase in blood drug concentrations, thereby reducing the incidence of systemic adverse reactions. But the efficacy and potentials are not fully recognized, and the tulobuterol patch has been used in the limited regions in the world [33].
12.3.7 Side Effects of β2-Adrenergic Receptor Agonists
Stimulation of β2-AR can produce resting sinus tachycardia and has the potential to precipitate cardiac rhythm disturbances in susceptible patients, although these seem to have remarkably few clinical implications. Exaggerated somatic tremor is troublesome in some older patients treated with higher doses of β2-agonists, whatever the route of administration, and this limits the dose that can be tolerated. Although hypokalemia can occur, especially when treatment is combined with thiazide diuretics [34], and oxygen consumption can be increased under resting conditions [35], these metabolic effects show tachyphylaxis unlike the bronchodilator actions. Mild falls in PaO2 can occur after administration of both short- and long-acting β2-agonists, but the clinical significance of these changes is doubtful. Despite the concerns raised some years ago related to β2-agonists in the management of asthma, further detailed study has found no association between β2-agonist use and an accelerated loss of lung function or increased mortality in COPD [1].
12.4 Methylxanthines
12.4.1 History
One of the earliest reports of the efficacy of methylxanthines in asthma was published in 1859, where Henry Hyde Salter, himself an asthmatic, described his experience that “one of the commonest and best reputed remedies of asthma… is strong coffee.” The first analysis of a xanthine derivative extracted from tea leaves was accomplished by Kossel, who was able to extract not only caffeine but also another xanthine derivative, a dimethylxanthine. The name theophylline was then applied to a compound that has two methyl groups (1,3-dimethylxanthine) (Fig. 12.3). Until 1930, xanthine derivatives were used in clinical practice only because of their diuretic and cardiotonic properties. Years later, a combination of theophylline and aminophylline was used intravenously as an effective bronchodilator in acute asthma. Since then, several other xanthines have been synthesized and are used clinically in various parts of the world [2].
12.4.2 Aminophylline
Aminophylline is the ethylenediamine salt of theophylline with higher solubility at a neutral pH. In vivo intravenous aminophylline has an acute bronchodilator effect in patients with asthma that is most likely to be due to a relaxant effect on ASM. Aminophylline also increases diaphragmatic contractility and reverses diaphragm fatigue. However, side effects such as nausea and vomiting are common, and the benefit/risk ratio is unknown. Aminophylline is also widely used for the treatment of acute exacerbations of COPD despite a lack of evidence to support this practice. It remains possible that aminophylline confers a small but clinically significant benefit when added to standard therapy. However, it was unable to find evidence for any clinically important additional effect of aminophylline treatment when used with high-dose nebulized bronchodilators and oral corticosteroids in patients with nonacidotic COPD exacerbations [36].
12.4.3 Theophylline
Theophylline, the most commonly used methylxanthine, is metabolized by cytochrome P450 mixed-function oxidases. Clearance of the drug declines with age. Many other physiological variables and drugs modify theophylline metabolism. Changes in inspiratory muscle function have been reported in patients treated with theophylline, but whether this reflects changes in spirometry or a primary effect on the muscle is not clear. Despite its extensive use in the treatment of respiratory disease, the precise molecular actions of theophylline have not been fully elucidated. Its efficacy in the treatment of patients with COPD or asthma has traditionally been attributed to nonselective phosphodiesterase (PDE) inhibition, resulting in an increase in cAMP by inhibition of PDE3 and PDE4 and an increase in cGMP by inhibition of PDE5. All studies that have shown efficacy of theophylline in COPD were performed with slow-release preparations.
Theophylline is less effective and less well tolerated than inhaled long-acting bronchodilators and is not recommended if those drugs are available and affordable. However, there is evidence for a modest bronchodilator effect compared with placebo in stable COPD. There is also some evidence of symptomatic benefit compared to placebo. Addition of theophylline to salmeterol produced a greater improvement in FEV1 and breathlessness than salmeterol alone. Low-dose theophylline reduces exacerbations but does not improve post-bronchodilator lung function [37, 38].
Theophylline can also act as a respiratory stimulant. Moreover, theophylline is able to improve diaphragmatic contractility and has anti-inflammatory properties. There is good evidence for inhibitory effects of theophylline on airway inflammation in patients with asthma and COPD, and these effects are seen at plasma concentrations below 10 mg/l [39].
12.4.4 Novel Xanthine
The positive clinical effects of theophylline in airway disease, combined with its advantageous oral bioavailability, has spurred the development of other xanthines, such as bamifylline, acebrophylline, and doxofylline, for the treatment of respiratory disease, with the anticipation that such drugs would have greater efficacy than theophylline but with an improved side effect profile.
12.4.5 Side Effects
Toxicity is dose related, a particular problem with the xanthine derivatives because their therapeutic ratio is small and most of the benefit occurs only when near-toxic doses are given. Methylxanthines are nonspecific inhibitors of all phosphodiesterase enzyme subsets, which explains their wide range of toxic effects. Problems include the development of atrial and ventricular arrhythmias (which can prove fatal) and grand mal convulsions (which can occur irrespective of prior epileptic history). Other side effects include headaches, insomnia, nausea, and heartburn, and these may occur within the therapeutic range of serum theophylline. These medications also have significant interactions with commonly used medications such as digitalis, Coumadin, etc. Unlike the other bronchodilator classes, xanthine derivatives may involve a risk of overdose (either intentional or accidental) [1].
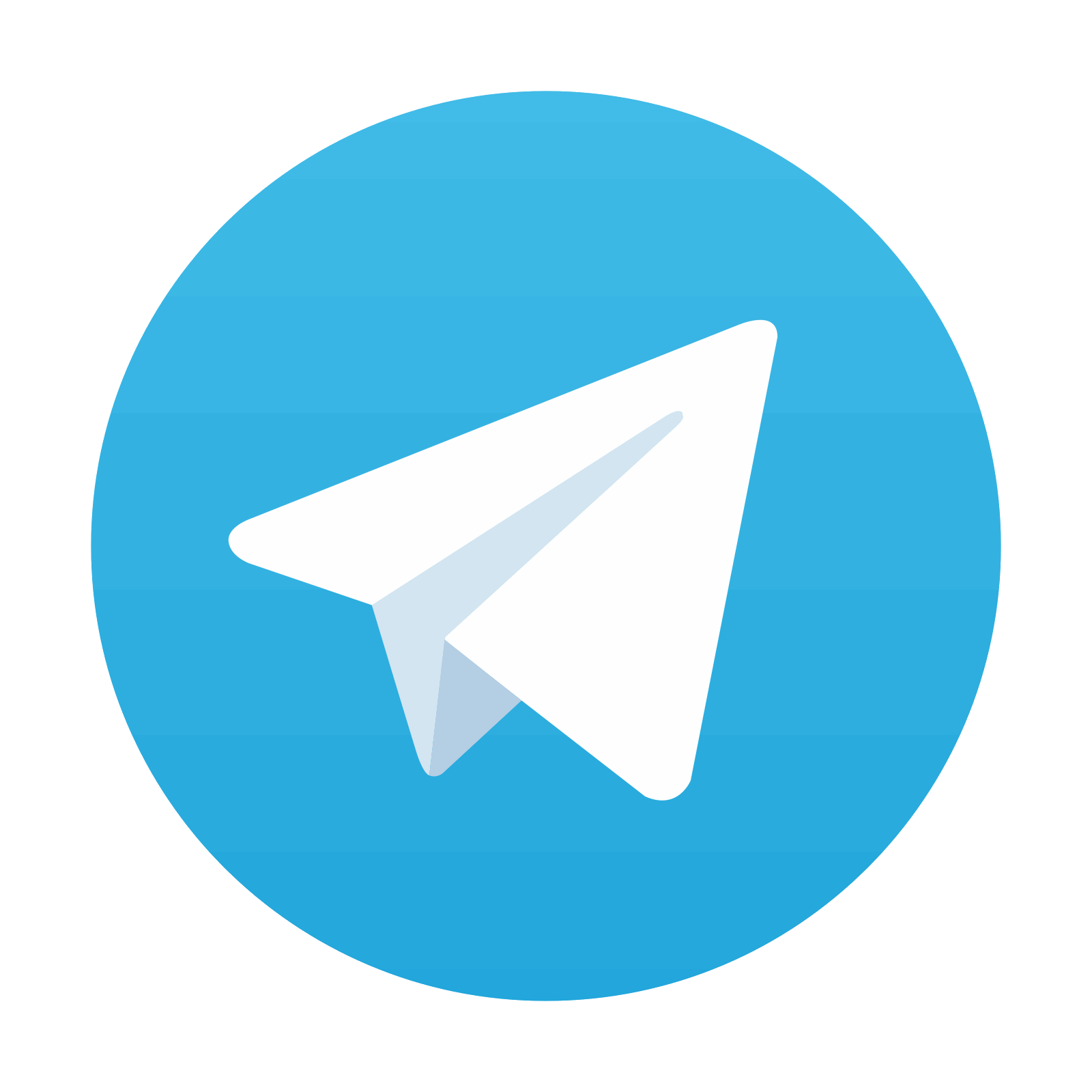
Stay updated, free articles. Join our Telegram channel

Full access? Get Clinical Tree
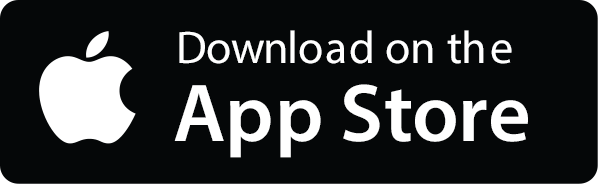
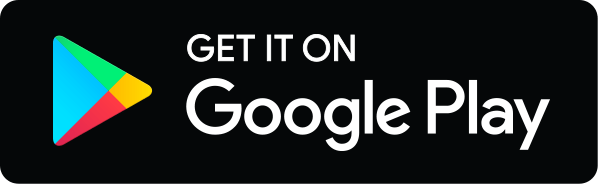