where n is the number of blood pressure readings, k ranges from 1 to n−1, and w k is the time interval between BP k and BP k + 1. ARV therefore accounts for the order of the measurements and weighs each value according to the measurement interval (Fig. 7.1).
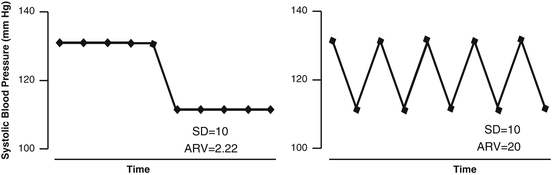
Fig. 7.1
Derivation of the ARV from blood pressure recordings. The ARV averages the absolute differences between consecutive readings and thereby accounts for the order of the blood pressure readings. For distinct blood pressure signals, SD can be the same, whereas ARV is not. ARV denotes average real variability . Reproduced with permission from Hansen TW et al. [17]
More recently, Rothwell and colleagues proposed blood pressure variability independent of the mean (VIM) as a new index [4, 11]. VIM [4, 11] is the within-subject SD divided by the within-subject mean blood pressure level to the power x and multiplied by the population mean blood pressure level to the power x. The power x is obtained by fitting a curve through a plot of SD against mean blood pressure level, using the model SD = a × mean x , where x is derived by nonlinear regression. The correlation of VIM with the other indexes of blood pressure variability is high [30], but VIM does not correlate with the blood pressure level [4, 11]. VIM therefore allows assessing association of outcome with blood pressure variability with little confounding by blood pressure level [4, 11]. Meanwhile, VIM is tied to the population being examined and cannot be compared across the population because VIM is derived from the distribution of the blood pressure values in each population.
Inconsistent Definitions and Poor Reproducibility of Variability Indexes
The morning surge in blood pressure is a good example to highlight how inconsistent definitions and poor reproducibility limit the clinical applicability of indexes of blood pressure variability. In 2003, Kario and colleagues introduced two definitions of the morning surge in blood pressure [18]. The sleep-trough morning surge is the difference between the morning pressure (the average blood pressure during the 2 h after awaking) and the lowest night-time blood pressure (the average of the lowest pressure and the readings immediately preceding and following the lowest value). The pre-awaking morning surge is the difference between the morning blood pressure (the average blood pressure during the 2 h after waking up) and the pre-awakening blood pressure (the average blood pressure during the 2 h before waking up). Other investigators redefined the pre-awakening morning surge as the blood pressure differences over 1-h intervals prior and after awakening or as the blood pressure difference between all readings during sleep and those obtained over 2 h after awakening [31]. Several investigators reported that an exaggerated morning surge predicted outcome [18–20]. However, using a variety of definitions of a single index of blood pressure variability induces confusion and raises the suspicion that definitions were revised to serve the hypothesis to be proven.
In 2008, Wizner and colleagues analyzed the substudy [32] on ambulatory blood pressure monitoring to the Systolic Hypertension in Europe (Syst-Eur) trial [33]. Patients underwent 24-h ambulatory blood pressure monitoring twice before randomization at a 1-month interval and once in 10 months after randomization to double-blind placebo [32]. In 173 patients with repeat recordings within 33 days (median), the short-term repeatability coefficients, expressed as percentages of maximal variation, ranged from 35 to 41 % for the day-time and night-time blood pressure, but from 52 to 75 % for the sleep-trough and the pre-awakening morning surge, higher values represent worse reproducibility. In 219 patients with repeat recordings within 10 months (median), the corresponding long-term estimates ranged from 45 % to 64 % and from 76 % to 83 %, respectively. In categorical analyses of the short-term repeatability of the sleep-trough morning surge and the pre-awakening morning surge, using the 75th percentile as arbitrary cut-off, surging status changed in 28.0 % and 26.8 % of patients (κ-statistic, ≤0.33). In the long-term, these proportions were 32.0 % and 32.0 %, respectively (κ-statistic, ≤0.20). The κ-statistic indicating moderate reproducibility is 0.4. Stergiou and associates confirmed the poor intra-individual reproducibility of the blood pressure surge in the morning after sleep and in the evening after siesta [31]. Using the four definitions described above [18, 31], the κ-statistics were consistently less than 0.20 [31].
The poor reproducibility of the morning surge and per extension blood pressure variability in general can be ascribed to several factors. Within individuals, blood pressure levels differ between rapid-eye-movement (REM) sleep and non-REM sleep. REM sleep is accompanied by neural sympathetic and electroencephalographic activity similar to that when awake, with distinct cardiovascular effects. In contrast, non-REM sleep is characterized by a suppression in neural sympathetic activity, resulting in a decrease in blood pressure [34]. Ambient temperature and season influence blood pressure levels during sleep and day-time. Cold conditions result in higher surges in the morning blood pressure, later sleep stage transition, and delayed sympathetic activation [35]. The position of the cuff relative to the heart level introduces variability, in particular during sleep, when subjects cannot consciously control body position. Methods of awakening, such as using an alarm clock or natural awakening, affect blood pressure rising at awakening. For patients on antihypertensive drugs, the times of dosing (e.g., morning vs. evening) and the duration of action of the drugs administered influence blood pressure level and the diurnal blood pressure variability, including the magnitude of the morning surge.
Recent Evidence on Blood Pressure Variability
Morning Blood Pressure Surge
Kario and coworkers studied stroke prognosis in 519 patients with hypertension on office measurement (63.6 % women; mean age, 72.5 years) [18]. They assessed silent cerebral infarction by magnetic resonance imaging. For analysis, patients were dichotomized according to the 90th percentile of the sleep-trough distribution (≥55 mmHg). During an average follow-up of 41 months (range, 1–68 months), 44 patients experienced a stroke, of whom two had a silent stroke. The 53 patients in the top tenth of the sleep-trough morning surge distribution, compared with the 466 remaining patients, had a higher baseline prevalence of multiple infarcts (57 % vs. 33 %; P = 0.001) and a higher stroke incidence (19 % vs. 7.3 %, P = 0.004) than the 466 remaining patients [18]. The top-ten patients were also older (77 vs. 72 years), had higher office (171 vs. 163 mmHg) and 24-h (143 vs. 138 mmHg) systolic blood pressures, and were followed for a longer period (41 vs. 37 months) [18]. Because of these disparities, 46 patients with exaggerated morning surge were matched with 145 control patients for age and 24-h systolic blood pressure. After matching, the relative risk of stroke in the morning surge compared with the control group was 2.71 (95 % confidence interval [CI], 1.05–7.21; P = 0.047) [18].
Studies published shortly after this seminal report [18] were not confirmatory [19, 20]. Among 1430 Japanese recruited in the framework of the Ohasama population study, the pre-awakening morning surge in systolic blood pressure marginally predicted cerebral hemorrhage (hazard ratio [HR] per 1−SD increase [+13.8 mmHg], 1.34; CI, 0.95–1.89), whereas the prognostic value for ischemic stroke was far from significant (HR, 0.97; CI, 0.79–1.19). Gosse and colleagues [20] recorded 31 cardiovascular events among 507 White hypertensive patients with a mean follow-up of 92 months [20]. With adjustments applied for age and 24-h systolic blood pressure, the risk of cardiovascular events was not associated with the pre-awakening systolic blood pressure, calculated as the difference of the first systolic blood pressure after standing up minus the last supine systolic blood pressure at awakening. For each 1-mm Hg increase, the estimate of relative risk amounted to 3.3 % (95 % CI, 0.8–5.8 %) [20].
Verdecchia and colleagues [22] investigated the relation between the day–night blood pressure dip and the early morning surge in a cohort of 3012 initially untreated subjects with essential hypertension [22]. The day-to-night reduction in systolic blood pressure showed a direct association (P < 0.0001) with the sleep-trough (r = 0.56) and the pre-awakening (r = 0.55) morning surge in systolic blood pressure [22]. Over a mean follow-up period of 8.4 years, 220 patients died and 268 experienced a cardiovascular event. A blunted sleep-trough (≤19.5 mmHg; the lowest quartile) and pre-awakening (≤9.5 mmHg) blood pressure surge were both associated with an excess risk of events (HRs, 1.66 [CI, 1.14–2.42] and 1.71 [CI, 1.12–2.71], respectively). However, neither patients with a high sleep-trough (>36.0 mmHg; the highest quartile) nor those with a high pre-awakening (>27.5 mmHg) systolic blood pressure had an increased risk of death or a cardiovascular complication.
Li and colleagues analyzed the International Database on Ambulatory blood pressure monitoring in relation to Cardiovascular Outcomes (IDACO) [21]. This resource included 12 randomly recruited population cohorts with follow-up of both fatal and nonfatal outcomes [21]. During a median follow-up of 11.4 years, 785 deaths and 611 fatal and nonfatal cardiovascular events occurred in 5645 IDACO participants (mean age, 53.0 years; 54.0 % women) [21]. While accounting for covariables and the night-to-day ratio of systolic blood pressure, the HR expressing the risk of all-cause mortality in the top tenth of the sleep-trough morning surge distribution (≥37.0 mmHg) compared with the remainder of the study population was 1.32 (CI, 1.09–1.59; Fig. 7.2). For cardiovascular and noncardiovascular mortality, the corresponding HRs were 1.18 (CI, 0.87–1.61) and 1.42 (CI, 1.11–1.80), respectively; for all cardiovascular, cardiac, coronary, and cerebrovascular events, the HRs amounted to 1.30 (CI, 1.06–1.60; Fig. 7.2), 1.52 (CI, 1.15–2.00), 1.45 (CI, 1.04–2.03), and 0.95 (CI, 0.68–1.32), respectively. Analyses of the risk associated with the top tenth of the distribution of the pre-awakening systolic morning surge (≥28.0 mmHg) generated similar results (Fig. 7.2). Furthermore, the risk of death or a major cardiovascular event in the 50th percentile group of the sleep-trough morning surge was over 35 % lower (P < 0.01; Fig. 7.2) than the average risk in the whole study population [21].
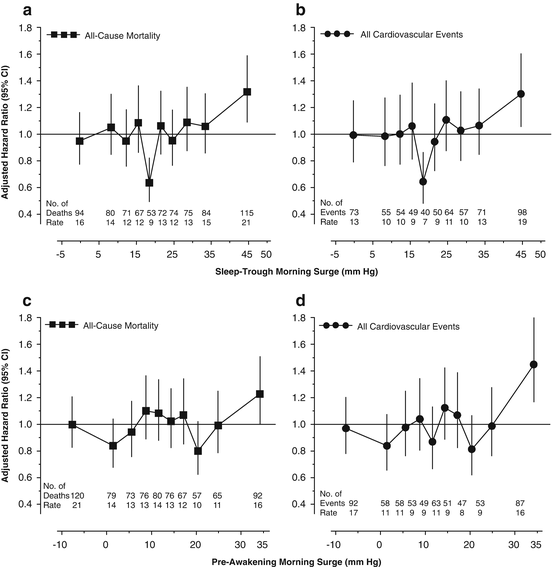
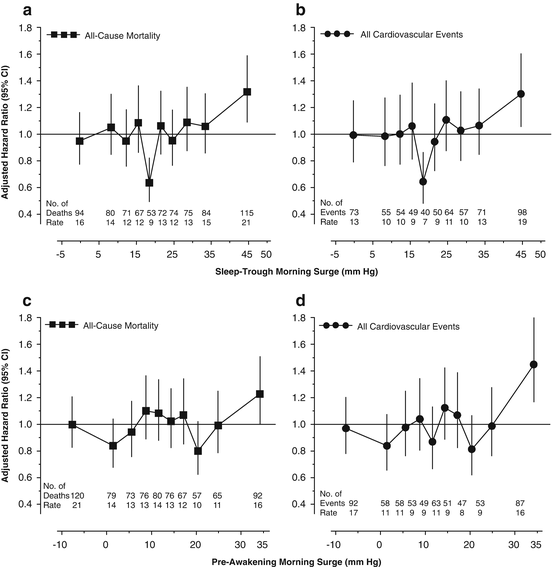
Fig. 7.2
Multivariable-adjusted hazard ratios (95 % CIs) for all-cause mortality (a, c) and for all fatal combined with nonfatal cardiovascular events (b, d) by ethnic- and sex-specific deciles of the sleep-trough (A and B) and the pre-awakening (c, d) morning surge in systolic blood pressure in 5645 participants. The hazard ratios express the risk in deciles compared with the average risk in the whole study population and were adjusted for cohort, sex, age, body mass index, smoking and drinking, serum cholesterol, history of cardiovascular disease, diabetes mellitus, antihypertensive drug treatment, 24-h systolic blood pressure, and the systolic night-to-day blood pressure ratio. The number of events and incidence rates (events per 1000 person–years) are also given for each decile. CI denotes confidence interval. Reproduced with permission from Li Y et al. [21]
In the Pressioni Arteriose Monitorate E Loro Associazioni (PAMELA) study, Bombelli and colleagues [23] analyzed ambulatory blood pressure data of 2011 people. Cardiovascular mortality showed a positive relation with the sleep-trough morning surge in unadjusted analyses (HR, 1.3; CI, 1.1–1.6), which disappeared after adjustment for covariables (HR, 0.9; CI, 0.7–1.1). Cardiovascular mortality, irrespective of adjustment, was unrelated to the pre-awakening morning surge (P ≥ 0.12). Along similar lines, in this Italian population study [23], there were no differences in the risks of total and cardiovascular mortality when the bottom and top tenths of the distributions of the sleep-trough and pre-awakening morning surge were compared (P ≥ 0.39).
Diurnal Blood Pressure Variability
Diurnal blood pressure variability encompasses the day-to-night changes in the blood pressure level and reading-to-reading blood pressure variability in 24-h ambulatory blood pressure recordings. In 1988, O’Brien reported for the first time that an abnormal circadian blood pressure profile with decreased night-time dipping had a more frequent history of stroke [36]. Subsequent studies of populations [37–40] and hypertensive cohorts [41–47] usually corroborated that an elevated nocturnal blood pressure is a harbinger of an unfavorable outcome. In spite of the apparent concordance between these previously published large-scale outcome studies [37–47], several potential limitations required further clarification of the prognostic accuracy of the day-time versus the night-time ambulatory blood pressure. Many studies considered only fatal outcomes [37, 38, 45, 46] or did not have the power to study cause-specific cardiovascular endpoints [37, 38, 40, 44]. Investigators dichotomized the night-to-day blood pressure ratio or applied widely different definitions of dipping status or of the day-time and night-time intervals.
The IDACO consortium therefore assessed the prognostic accuracy of day versus night ambulatory blood pressure in 7458 people enrolled in prospective population studies in Europe, China, and Uruguay [48]. Median follow-up was 9.6 years. Adjusted for day-time blood pressure, confounders, and cardiovascular risk factors, night-time blood pressure predicted (P < 0.01) total (n = 983) cardiovascular (n = 387) and noncardiovascular (n = 560) mortality [48]. Conversely, adjusted for night-time blood pressure and other covariables, day-time blood pressure predicted only noncardiovascular mortality (P < 0.05), with lower blood pressure levels being associated with increased risk. Both day-time and night-time blood pressure consistently predicted (P < 0.05) all cardiovascular events (n = 943) and stroke (n = 420) [48]. Adjusted for night-time blood pressure, day-time blood pressure lost prognostic significance for cardiac events (n = 525; P ≥ 0.07). Adjusted for 24-h blood pressure, the night-to-day blood pressure ratio predicted mortality, but not fatal combined with nonfatal events. Participants with a systolic night-to-day blood pressure ratio value of ≥1 were older, at higher risk of death, and died at an older age than those whose night-to-day ratio was normal (≥0.80 to <0.90) [48].
In contrast to commonly held views, the IDACO analysis showed that day-time blood pressure adjusted for night-time blood pressure predicted fatal combined with nonfatal cardiovascular events, except in treated patients, in whom antihypertensive drugs probably reduced blood pressure during the day, but not at night [48]. The increased mortality in patients with higher night-time than day-time blood pressure probably indicated reverse causality. The IDACO findings confirmed that both day-time and night-time blood pressure hold valuable prognostic information [48]. They supported the conclusion that recording blood pressure during the whole day should be the standard in clinical practice. A 2014 IDACO publication [49] highlighted that identification of truly low-risk white coat hypertension requires setting thresholds simultaneously to 24-h, day-time, and night-time blood pressures. In line with the 2007 report [48], Fan and colleagues also demonstrated that isolated nocturnal hypertension predicted cardiovascular outcome even in patients who are normotensive on office or on ambulatory day-time blood pressure measurement [50].
Reading-to-Reading Blood Pressure Variability
Hansen and colleagues also assessed blood pressure variability from the SD and ARV (Fig. 7.3) in 24-h ambulatory recordings in the IDACO population [17]. Higher diastolic ARV in 24-h ambulatory blood pressure recordings predicted (P ≤ 0.03) total (HR, 1.13; CI, 1.07–1.19) and cardiovascular (HR, 1.21; CI, 1.12–1.31) mortality and all types of fatal combined with nonfatal endpoints (HR, ≥1.07) with the exception of cardiac and coronary events (HR, ≤1.02; P ≥ 0.58). Similarly, higher systolic ARV in 24-h ambulatory recordings predicted (P < 0.05) total (HR, 1.11; CI, 1.04–1.18) and cardiovascular (HR, 1.17; CI, 1.07–1.28) mortality and all fatal combined with nonfatal endpoints (HR, ≥1.07), with the exception of cardiac and coronary events (HR, ≤1.03; P ≥ 0.54). SD predicted only total and cardiovascular mortality. The incremental cardiovascular risk explained by adding ARV to models already including 24-h ambulatory blood pressure level and other covariables was less than 1 %. This report established that reading-to-reading blood pressure variability is an independent risk factor, significant in a statistical but not in a clinically meaningful manner. It highlighted that the level of the 24-h blood pressure remains the primary blood pressure-related risk factor to account for in clinical practice [17].
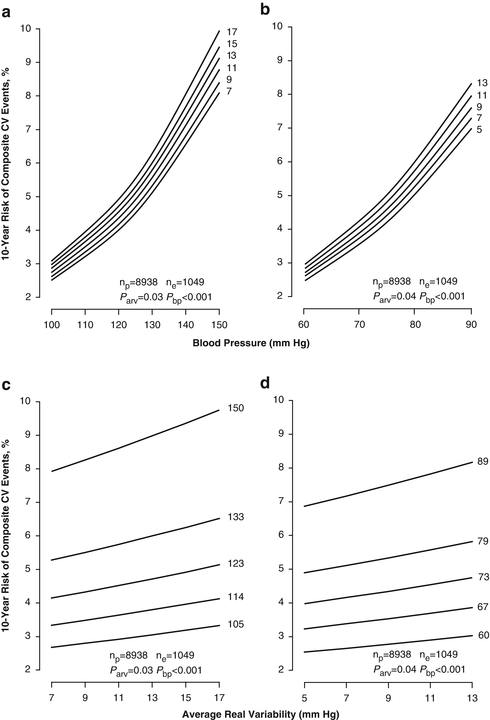
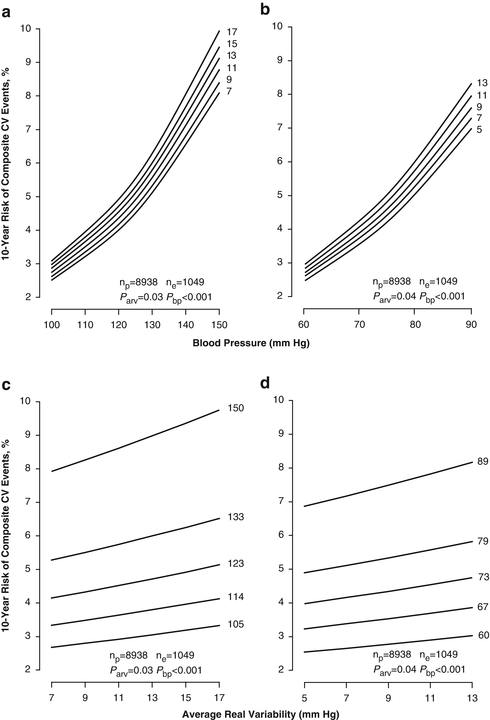
Fig. 7.3
Ten-year absolute risk of combined cardiovascular events in relation to 24-h blood pressure (a, b) at different levels of systolic and diastolic ARV24 and in relation to ARV24 (c, d) at different levels of 24-h systolic and diastolic blood pressure. The analyses were standardized to the distributions (mean or ratio) of cohort, sex, age, 24-h heart rate, body mass index, smoking and drinking, serum cholesterol, history of cardiovascular disease, diabetes mellitus, and treatment with antihypertensive drugs. In panels a and b, the risk functions span the 5th–95th percentile interval of the 24-h blood pressure and correspond to the 5th, 25th, 50th, 75th, and 95th percentiles of ARV24. In panels c and d, the risk functions span the 5th–95th percentile interval of ARV24 and correspond to the 5th, 25th, 50th, 75th, and 95th percentiles of the 24-h blood pressure. P-values are for the independent effect of ARV24 (P arv) and 24-h blood pressure (P bp). n p and n e indicate the number of participants at risk and the number of events. ARV24 denotes average real variability over 24 h. Reproduced with permission from Hansen TW et al. [17]
Palatini and associates analyzed 7112 untreated hypertensive participants (43.8 % women; mean age, 50.8 years) with day-time and night-time ambulatory blood pressure enrolled in 6 prospective cohorts [51]. During a median of 5.5 years of follow-up, 130 fatal and 455 nonfatal cardiovascular events occurred. In a multivariable-adjusted Cox model, the SD of the night-time systolic blood pressure predicted cardiovascular mortality (HR, 1.48; CI, 1.20–1.84) and morbidity (HR, 1.83; CI, 1.17–2.86), whereas SD of day-time systolic blood pressure did not (P ≥ 0.096). They proposed as optimal cutoff limits for the night-time SD 12.2 mmHg systolic and 7.9 mmHg diastolic. Participants above these arbitrary thresholds had a 41–132 % higher risk of a composite cardiovascular endpoint than those below these cut-off limits (P ≤ 0.028). As outlined above, SD as an index of blood pressure variability is closely correlated with blood pressure level. The authors stated that they obtained similar results if they used CV instead of SD, but did not show the data [51].
Within-Visit and Between-Visit Blood Pressure Variability in a Prospective Study
In a randomly recruited Flemish population sample (n = 2944; 50.7 % women; mean age, 44.9 years), highly trained observers measured blood pressure five times consecutively at each of two home visits and recorded the incidence of adverse health outcomes in relation to the variability of systolic blood pressure at enrolment [3]. Schutte and colleagues computed VIM, MMD, and ARV for within-visit variability (WVV), between-visit variability, and overall—within-visit combined with between-visit—variability. Over a median follow-up of 12 years, 401 deaths occurred and 311 participants experienced a fatal or nonfatal cardiovascular event. Overall (ten readings over two visits), systolic blood pressure variability averaged (SD) 5.45 (2.82) units for VIM, 15.9 (8.4) mm Hg for MMD, and 4.08 (2.05) mmHg for ARV. In multivariable-adjusted analyses, overall and within- and between-visit blood pressure variability did not predict total or cardiovascular mortality or the composite of any fatal plus nonfatal cardiovascular endpoint. For instance, the HRs for all cardiovascular events combined in relation to overall variability as captured by VIM, MMD, and ARV were 1.05 (CI, 0.96–1.15), 1.06 (CI, 0.96–1.16), and 1.08 (CI, 0.98–1.19), respectively. By contrast, mean systolic blood pressure level was a significant predictor of all endpoints under study, independent of blood pressure variability [3]. These findings suggest that, in the general population, within-subject blood pressure variability does not have any prognostic significance over and beyond systolic blood pressure level [3].
Within-Visit and Between-Visit Blood Pressure Variability in Syst-Eur
Results from randomized clinical trials constitute the strongest evidence for the role and reversibility of any cardiovascular risk factor. In the Syst-Eur trial, Hara and colleagues investigated whether systolic blood pressure variability determines prognosis over and beyond level. Using a double-blind design, 4695 patients (≥60 years) with isolated systolic hypertension (160–219/<95 mmHg) were randomly allocated to active treatment or matching placebo. Active treatment consisted of nitrendipine (10–40 mg/day) with possible addition of enalapril (5–20 mg/day) and/or hydrochlorothiazide (12.5–25.0 mg/day) [52, 53]. They assessed whether on-treatment systolic blood pressure level, visit-to-visit VIM, or WVV predicted total (n = 286) or cardiovascular (n = 150) mortality or cardiovascular (n = 347), cerebrovascular (n = 133), or cardiac (n = 217) endpoints [54].
Before randomization, patients of the placebo and active-treatment groups had similar characteristics. Of 4695 participants, 3138 (66.8 %) were women. Age averaged 70.2 years and blood pressure 173.8 mmHg systolic and 85.5 mmHg diastolic. Assessed during the run-in period, visit-to-visit blood pressure variability, as captured by SD (mean, 6.4 mmHg), CV (3.65), MMD (12.1 mmHg), and ARV (7.2 mmHg), increased across fourths of the distribution of systolic blood pressure before randomization (P < 0.0001). WVV (mean, 3.4 mmHg) during the run-in period also increased with higher systolic blood pressure (P < 0.0001), whereas VIM (6.3 units) did not increase with higher run-in systolic blood pressure (P = 0.084) [54]. In all 4695 patients, the correlation coefficients of VIM with systolic blood pressure level were 0.01 (P = 0.59) during the run-in period and -0.01 (P = 0.75) during follow-up after randomization.
At 2 years (median follow-up), active treatment lowered systolic blood pressure by 10.5 mmHg (P < 0.0001) more than placebo, whereas the between-group differences in blood pressure variability were not significant, averaging 0.29 units (P = 0.20) for VIM and 0.07 mmHg (P = 0.47) for WVV (Fig. 7.4) [54]. Active treatment reduced (P ≤ 0.048) cardiovascular (-28 %), cerebrovascular (-40 %), and cardiac (-24 %) endpoints. In analyses dichotomized by the median, patients with low vs. high VIM had similar event rates (P ≥ 0.14). Low vs. high WVV was not associated with event rates (P ≥ 0.095), except for total and cardiovascular mortality on active treatment, which were higher with low WVV (P ≤ 0.0003). In multivariable-adjusted Cox models, systolic blood pressure level predicted all endpoints (P ≤ 0.0043), whereas VIM did not predict any adverse outcome (P ≥ 0.058). Except for an inverse association with total mortality (P = 0.042), WVV was not predictive (P ≥ 0.15). Sensitivity analyses, from which the investigators excluded blood pressure readings within 6 months after randomization, 6 months prior to an event, or both, were confirmatory [54]. The double-blind placebo-controlled Syst-Eur trial irrefutably demonstrated that blood-pressure lowering treatment reduces cardiovascular complications by decreasing systolic blood pressure level but not systolic variability and that higher systolic blood pressure level predicted risk without material contribution of variability.
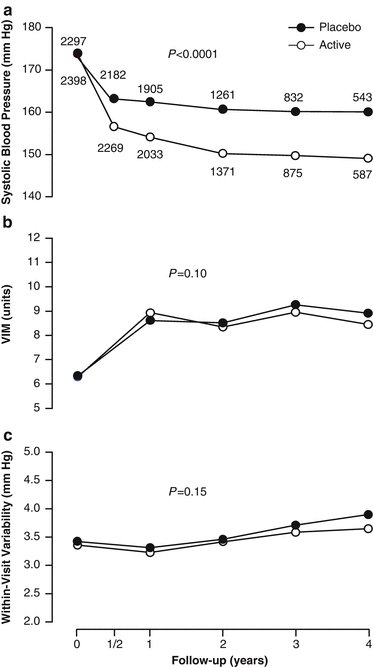
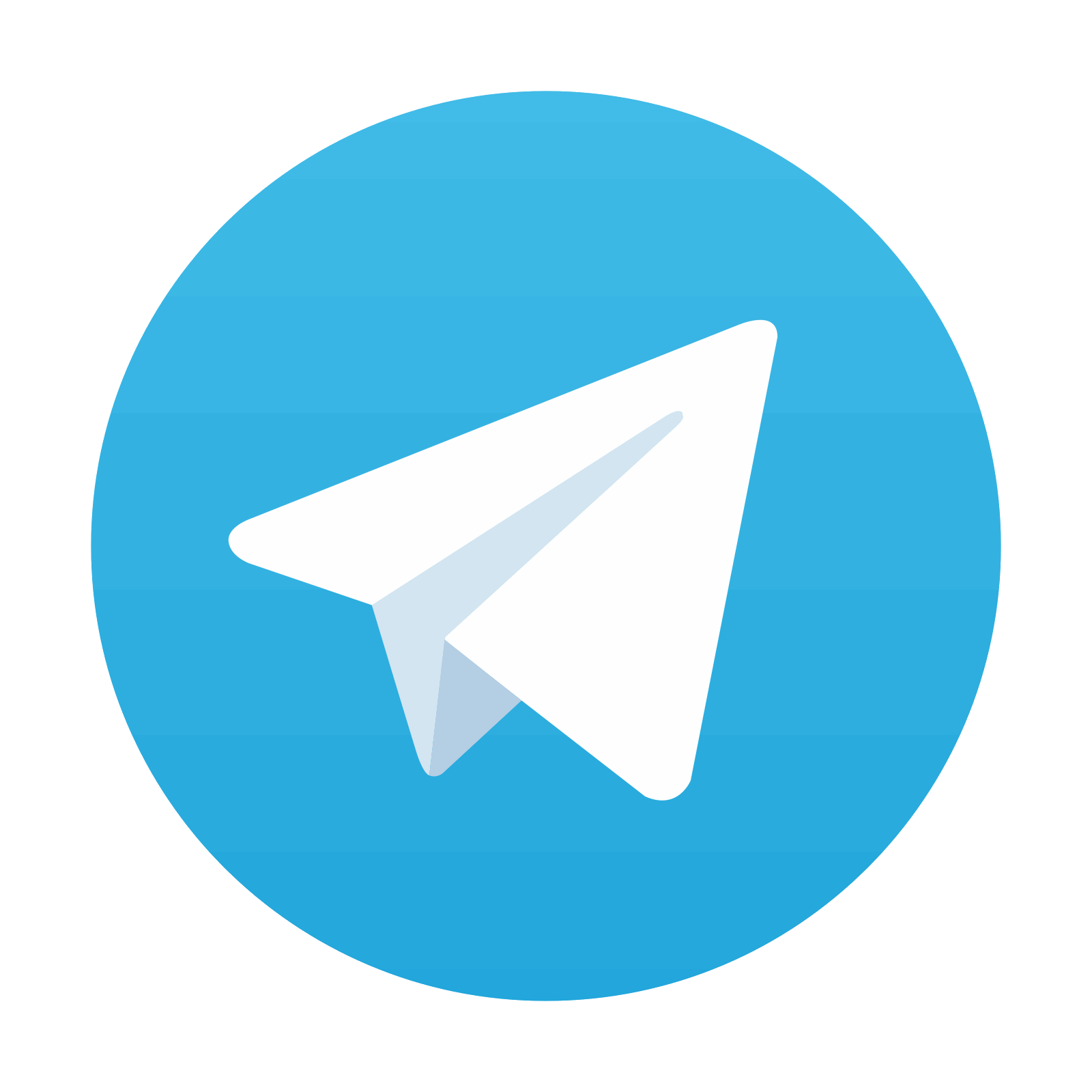
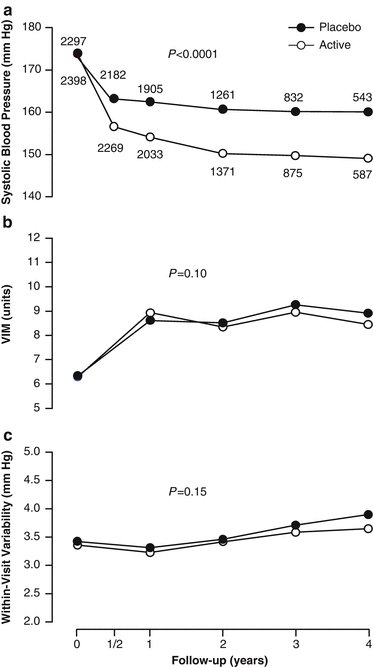
Fig. 7.4
Systolic blood pressure level (a), VIM (b), and within-visit variability (c) at randomization and during follow-up. Values at randomization and at annual intervals during follow-up were derived from at least six blood pressure readings, two at each of three consecutive visits. The blood pressure level at 6 months is the average of four blood pressure readings at two consecutive visits. The computation of variability requires at least three visits. Variability is therefore not plotted at 6 months. P values indicate the significance of the average between-group difference throughout follow-up. VIM denotes variability independent of the mean. Reproduced with permission from Hara A et al. [54]
< div class='tao-gold-member'>
Only gold members can continue reading. Log In or Register a > to continue
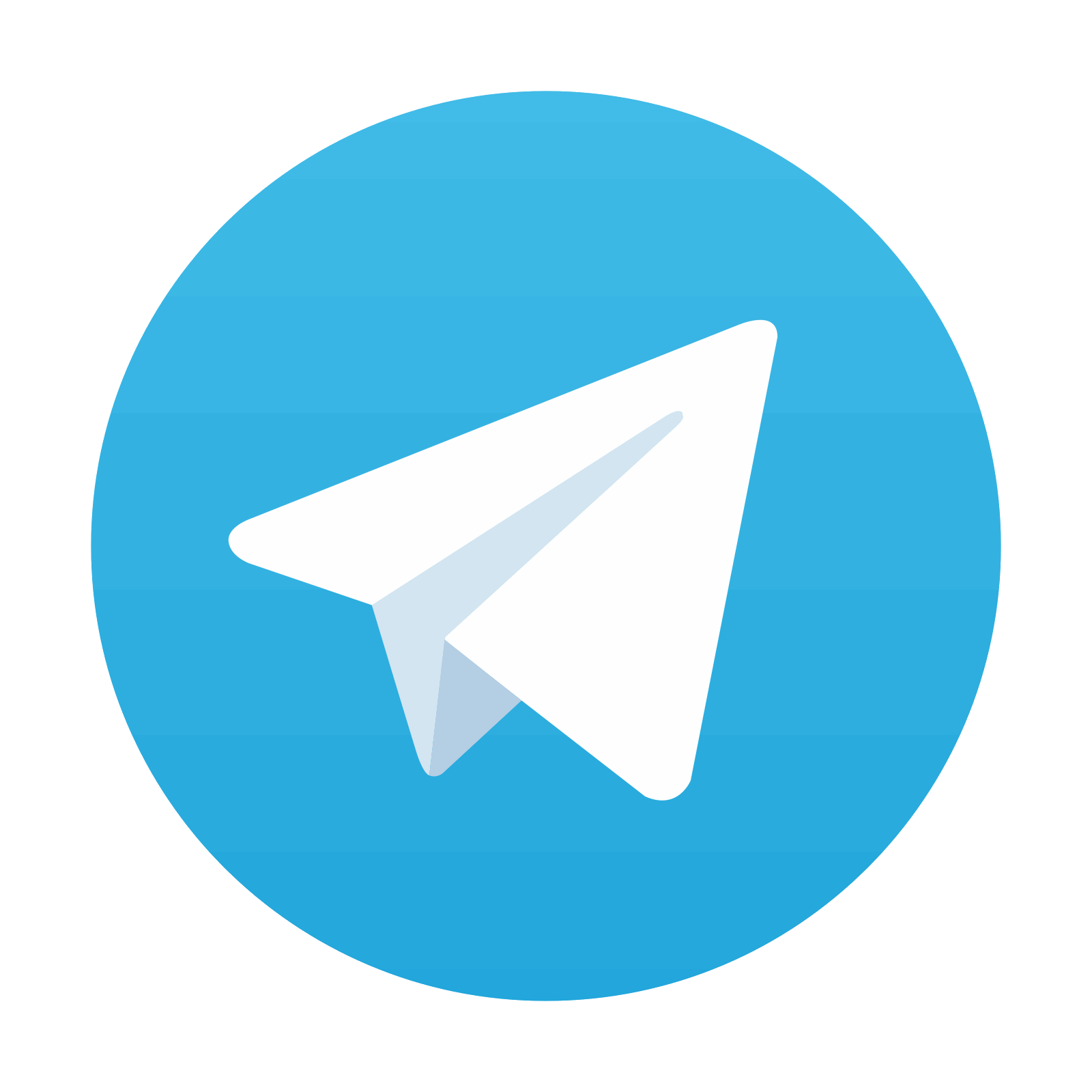
Stay updated, free articles. Join our Telegram channel

Full access? Get Clinical Tree
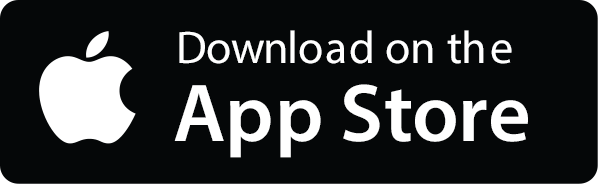
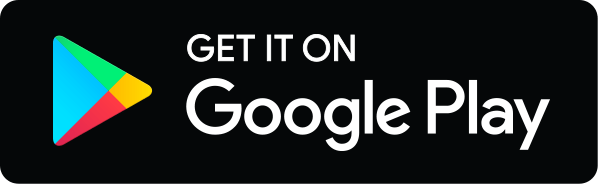
