, Dilip R. Karnad2 and Snehal Kothari3
(1)
Cardiac Safety Services Quintiles, Durham, North Carolina, USA
(2)
Research Team, Cardiac Safety Services Quintiles, Mumbai, India
(3)
Cardiac Safety Services Global Head, Cardiac Safety Center of Excellence Quintiles, Mumbai, India
A variety of therapeutic agents or chemical substances may increase blood pressure… When use of a chemical agent which increases blood pressure is mandatory, anti-hypertensive therapy may facilitate continued use of this agent (Grossman et al. 2015).
11.1 Introduction
This chapter focuses on unintended drug-induced blood pressure responses. While both increases and decreases are legitimate areas of investigation (Kane-Gill et al. 2014; Kennelly and Esaian 2013), our attention falls on increases. As was noted in Sect. 3.4.4, high blood pressure is authoritatively regarded as the greatest threat to the global burden of disease (Horton 2013; Das and Samarasekera 2013; Lim et al. 2013). As hypertension has been studied across several decades, we have learned that there are multiple ways in which increased blood pressure may occur (see Frohlich 1977; Page 1982; Dustan 1990; Harrison 2013). It has now also become clear that noncardiovascular drugs can lead to off-target increases in blood pressure and can also do so via a variety of mechanisms of action. As Grossman and Messerli (2012) observed, “Some agents cause either sodium retention or extracellular volume expansion, or activate directly or indirectly the sympathetic nervous system. Other substances act directly on arteriolar smooth muscle or do not have a defined mechanism of action. Some medications that usually lower BP may paradoxically increase BP, or an increase in pressure may be encountered after their discontinuation.”
Two issues are addressed in this chapter. First, as we write this chapter, there is not a regulatory landscape in place addressing the prospective exclusion of unacceptable blood pressure increases. However, what might sensibly be done during drug development to identify signals of drug-induced blood pressure increases is addressed. Second, when such increases are identified, what strategies might be employed by physicians in clinical practice? Currently there are no authoritative guidelines, and so we provide expert opinion from Professor Michael Weber.
11.2 Drug-Induced Blood Pressure Changes
We noted in the previous chapter that some oncologic agents are associated with blood pressure increases, and examples were provided in Table 10.1. Table 11.1 provides additional examples from multiple drug classes.
Table 11.1
Examples of drugs that affect blood pressure
Drug/drug category | Blood pressure effects |
---|---|
Antidepressants | Several antidepressant agents may increase blood pressure by activating the sympathetic nervous system (SNS). These effects may be more pronounced in older patients and dose dependent |
Anti-HIV therapy | Highly active antiretroviral therapy can cause a rise in blood pressure but not usually before 6 months |
Drugs activating the SNS | Drugs causing hypertension include phenylephrine hydrochloride (used in upper respiratory decongestants and ophthalmic drops), dipivalyl adrenaline hydrochloride (ophthalmic drops systems), epinephrine (used as bronchodilator, decongestant, in antihemorrhoidal treatment), and phenylpropanolamine (anorexic agent and upper respiratory decongestant) |
Drugs for treating addiction | Disulfiram for the management of alcoholism may cause a slight increase in BP, and severe hypertension may occur in patients with alcoholic-induced liver disease |
Drugs for treating malignancy | Antivascular endothelial growth factor drugs for the treatment of various malignancies may cause hypertension. About 1 % of all patients on antiangiogenic therapy develop a life-threatening hypertensive crisis |
Erythrocyte-stimulating agents | Erythrocyte-stimulating agents such as erythropoietin increase BP in as many as 20 % of patients with anemia or chronic kidney disease |
Immunosuppressive agents | The incidence of cyclosporine-associated hypertension after renal, bone marrow, and cardiac transplantation varies between 30 % and 100 %. It is also common in patients with autoimmune disease and in patients with psoriasis treated with cyclosporine. Cyclosporine-induced hypertension is characterized by disturbance of the circadian rhythm, with the absence or reversal of the normal nocturnal fall in blood pressure. Hypertension usually decreases after the withdrawal or substitution of cyclosporine immunosuppression but may not remit completely |
Nonsteroidal anti-inflammatory | NSAIDs can induce an increase in blood pressure and interfere with antihypertensive treatment, mitigating or abolishing their effect. NSAIDs interfere with some antihypertensive agents such as diuretics, beta-blockers, and angiotensin-converting enzyme inhibitors but do not interact with calcium antagonists and central-acting drugs. Significant blood pressure elevations have been seen with acetaminophen in patients with coronary artery disease |
Recombinant human erythropoietin (r-HuEPO) | r-HuEPO, which is effective in correcting anemia in patients with end-stage renal failure and patients with malignancies, induces hypertension or worsens existing hypertension in 20–30 % of patients |
Sex hormones | Around 5 % of women taking estrogen-containing oral contraceptives develop hypertension (this does not occur with progesterone-only anovulant drugs). Any rise in blood pressure is usually small and reversible on cessation of therapy, but severe hypertensive episodes, including malignant hypertension, may occur |
Steroids | Hypertension occurs in at least 20 % of patients treated with synthetic corticosteroids in a dose-dependent fashion; oral cortisol at doses of 80–200 mg⁄d can increase systolic blood pressure as much as 15 mmHg within 24 h |
Stimulants | Stimulant drugs for the treatment of attention deficit hyperactivity disorder (amphetamine and dextroamphetamine, atomoxetine, methylphenidate) can raise blood pressure significantly |
There are clear reports of clinical concern. Grossman and Messerli (Grossman and Messerli 2012), for example, observed that “severe hypertension involving encephalopathy, stroke, and irreversible renal failure have been reported.” However, there is still a lack of systematic study of this area, and the clinical relevance of small, transient drug-induced changes in blood pressure to symptomatology, morbidity, or mortality is not well characterized (O’Brien and Turner 2013).
Interactions between noncardiovascular drugs and antihypertensives are also potential sources of unwanted changes in blood pressure: such interactions may either negate or potentiate the efficacy of an antihypertensive agent. Rifampicin considerably reduces the plasma concentrations and the renin-inhibiting effect of aliskiren and some calcium antagonists by decreasing oral bioavailability. Another example is sitagliptin, a dipeptidyl peptidase IV inhibitor used to reduce hyperglycemia in patients with diabetes, which has been shown to attenuate the blood pressure-lowering effect of high-dose enalapril by stimulating the sympathetic nervous system (Grossman and Messerli 2012).
11.3 Preapproval Investigations of Drug-Induced Blood Pressure Responses
Similarly to the investigation of a noncardiac drug’s proarrhythmic liability, investigation of a noncardiovascular drug’s liability to increase blood pressure can occur in both nonclinical and clinical development programs. These avenues of research are discussed in turn.
11.3.1 Nonclinical Investigations
While detection of large, obvious changes is not challenging, detecting small and subtle changes is considerably more difficult. Authier and colleagues commented as follows (Authier et al. 2015, p. 222):
Nonclinical evaluation of a drug’s effects on blood pressure can involve multiple approaches, ranging from in vitro subcellular assays to fully integrated in vivo animal models (Sager et al. 2013). Early exploratory safety pharmacology studies may involve receptor-binding assays (frequently followed by function-based assays) to identify potential off-target effects of drug candidates at sites recognized for modulating vascular tone. Investigations of any potential mechanism(s) to alter kidney sodium handling are also common.
Cardiovascular safety pharmacology has been preoccupied with drug-induced changes in the electrocardiogram, and by comparison there has been little in the way of contemporaneous improvements in the level of complexity and sophistication involved in blood pressure assessment. Thus, it is important to understand the nature of drug-induced changes in blood pressure, appreciate the plethora of agents currently used clinically (and over the counter) that alter blood pressure, and understand safety pharmacology study design in order to optimize assessment of a new chemical entity or biological agent in this context.
The employment of anesthetized animals facilitates the administration of higher exposures and the use of more extensive instrumentation. It also enables a more thorough hemodynamic evaluation, including assessment of vascular resistance, myocardial contractility, and cardiac output. However, anesthesia itself can have an effect on responses to the drug, generally being associated with greater hemodynamic responses: both conscious and anesthetized animal models can therefore be informative (Authier et al. 2008). Dedicated cardiovascular studies evaluate acute blood pressure effects of drugs in conscious dogs or nonhuman primates: these studies incorporate continuous recording of blood pressure using indwelling catheters.
The use of telemetry systems may prove useful for detecting delayed effects or those resulting from metabolites. The use of trained animals and well-controlled environments can increase the sensitivity and precision of cardiovascular evaluations. The conscious beagle dog model can be useful here due to the peaceful temperament of this animal.
11.3.2 Clinical Investigations of Drug-Induced Blood Pressure Responses
Clinical evaluations of a drug’s off-target impact on blood pressure are predicated on several considerations related to the presumed mechanism of action of the blood pressure effects and the intended treatment population (Sager et al. 2013). In this area of investigation, it may not be possible to design a single dedicated study analogous to the employment of a TQT study or a QTc exposure–response study in the domain of proarrhythmic cardiac safety. Identifying potential hemodynamic effects in young healthy participants with heightened sympathetic nervous system activity, for example, may not provide useful information concerning the likely effects seen in older populations, given their lower levels of sympathetic sensitivity (Bhide et al. 2012). The opposite concern is true in the case of nonsteroidal anti-inflammatory drugs: the impact of sodium and volume retention on blood pressure is much less in younger individuals with normal renal handling of sodium and water than in the population most likely to be prescribed chronic therapy, i.e., older individuals with osteoarthritis and pain. Therefore, a “totality of evidence” approach may be the most informative strategy for a comprehensive clinical assessment of off-target blood pressure effects, with attention being paid to both magnitude and duration (Sager et al. 2013).
Several characteristics of Phase I trials have been discussed in previous chapters, and the same advantages and limitations of their nature are seen in this realm of investigation. With regard to advantages, these studies often see the highest levels of drug exposure. Second, the controlled environment in which they are conducted makes it easier to perform thorough blood pressure assessment: measuring equipment and techniques can be standardized, and measurements timed to blood withdrawals to permit pharmacokinetic information to be collected. Third, collection of repeated blood pressure measurements over time facilitates analysis of change from baseline levels not only at the end of the trial but also at various time points during dosing intervals. Exposure–response relationships can be assessed at both peak and trough drug concentrations.
Limitations of Phase I studies include the small number of participants employed and the consequent limited statistical power and their relatively short duration. Given that the participants are typically relatively young healthy adults (in this setting, the pertinent characteristics of “healthy” are having normal blood pressures and normal renal function), care must be taken when attempting to extrapolate findings to populations most likely to be prescribed treatment, such as older individuals and patients with cardiovascular disease, and to longer durations of exposure to the drug.
Phase II trials still provide the opportunity for rigorous standardization of blood pressure monitoring equipment and technique (something that is more difficult to achieve in Phase III trials). One goal is further investigation of any signal detected in the completed Phase I studies. A second goal results from the employment in Phase II development of individuals with the disease for whom the drug is intended: depending on the mechanism of action of an off-target blood pressure increase, it is therefore possible that a blood pressure signal may be seen in this setting for the first time. Additionally, treatment duration is typically longer than in Phase I studies, the reversibility of any blood pressure effects can be easily determined by programmed assessments after the active treatment period, and since placebo is a commonly used comparator, assessment of the drug’s effects on blood pressure can control for regression to the mean and other common confounders (Sager et al. 2013). Phase II is also an opportune time to perform dedicated blood pressure studies that employ out-of-office blood pressure measurement modalities, i.e., home blood pressure monitoring and ambulatory blood pressure monitoring.
Various blood pressure measurement modalities that may be useful in clinical investigations are presented in Table 11.2.
Table 11.2
Blood pressure measurement modalities
Modality | Strengths | Limitations |
---|---|---|
Clinic blood pressure measurement using auscultation by observer | Traditional standard used in epidemiologic and primary and secondary cardiovascular trials | Poor reproducibility caused by white-coat and masked blood pressure effects. Observer bias. Concern regarding environmental mercury |
Clinic blood pressure measurement using noninvasive auscultation devices | Removes environmental concern regarding mercury. Enhanced validation and easier to read the blood pressure column | Poor reproducibility caused by white-coat and masked blood pressure effects. Observer bias |
Clinic oscillometric blood pressure measurement (digital) | Removes observer bias. Can select inflation sequence. Improves reproducibility | Precision can be a concern for certain devices, but it is likely improved compared with the first two modalities discussed |
Ambulatory blood pressure monitoring | Provides a large number of readings over a 24-h period, thereby enhancing reproducibility. Minimal placebo effect. Obtains blood pressure readings during sleep | Expensive compared with other modalities. Participant adherence related to multiple monitoring sessions can be problematic. Can be a relatively high preponderance of unusable recordings in some studies |
Centralized office blood pressure monitoring | Removes some observer bias. Can select inflation sequence. Improves reproducibility. Provides early visibility into blood pressure trends in the clinic | Same as other office-based measurements. Study site education is required to implement process |
Self-measured home blood pressure (digital) | Removes white-coat and masked effects. Provides out-of-office readings that can be timed to drug dosing. Correlates to daytime ambulatory blood pressure measurements | Device precision can be a concern with some devices. Requires patient training and requires them to transcribe blood pressure readings into a logbook |
Self-measured home blood pressure measurement using telemonitoring | Removes white-coat and masked blood pressure effects. Avoids loss of data by the participants (failure to transcribe readings into the logbook). Ability to transfer and process large amount of data easily. Trend analysis can be performed. Alert criteria can be built into the system | Requires additional training and working with telecommunication providers to move to a data transmission focus |
Findings during Phase II development often determine the clinical safety monitoring needs for studies in Phase III development. In this case, they may identify what (if any) additional cardiovascular/hemodynamic evaluations or substudies might be informatively conducted during Phase III development.
Multiple influences in Phase III trials, generated by the use of multiple investigator sites with the likelihood of less rigorous standardization of measurement modalities and techniques, may make it more difficult to evaluate mean blood pressure responses accurately across the larger sample sizes used in these trials. However, they offer a compensatory advantage: the likelihood of the identification of individuals showing particularly large blood pressure increases (outliers) is increased, as is the likelihood of identifying individuals experiencing adverse events that may be due to the drug’s effects on their blood pressure. Thus, a richer picture of individual differences can emerge in Phase III trials.
Studies employing out-of-office assessments increase the complexity of Phase III development, but they should be incorporated for participant safety and to better characterize the drug’s effects if a signal was detected in Phase I and/or Phase II development (Sager et al. 2013).
Assuming that blood pressure data have been ascertained in a consistent manner across the entire Phase III development plan, data pooled across all studies can provide useful additional insights regarding any drug-induced blood pressure changes over and above those gleaned from individual studies: these include the long-term effects of the drug, mean effects for a participant population, and assessment of individual variation of the effect, most notably a description of the extent of occurrence of outliers. In addition to these blood pressure data themselves, other metrics are informative. For example, discontinuation rates, adverse event reports of hypertension, and the frequency of changes of concomitant antihypertensive medications can be compared between treatment groups. In a large Phase III program, prespecified analyses may yield additional insights regarding important patient subgroups (e.g., the elderly, those with treated hypertension, and African and Hispanic Americans).
11.4 Learning from the Employment of Ambulatory Blood Pressure Monitoring in the Clinical Development of Antihypertensive Agents
The use of ambulatory blood pressure monitoring in pharmacologic trials assessing the efficacy of blood pressure-lowering drugs is now reasonably well established (O’Brien 2011a, 2012). While the intents of trials examining on-target and off-target blood pressure responses differ, i.e., provide compelling evidence of efficacy for antihypertensives and prospectively excluding unacceptable increases in blood pressure for noncardiovascular drugs, the methodological considerations for the employment of ambulatory blood pressure monitoring in the two contexts are very similar, as are the advantages conveyed. We can therefore learn from its use in trials of antihypertensive agents, and the following case study is illuminating.
Since the mechanisms determining blood pressure may differ at different times within the 24-h cycle, it is not surprising that drugs can vary in their effects in these windows of time. The ability of ambulatory blood pressure monitoring to detect blood pressure changes that clinic blood pressure measurement had failed to identify was well illustrated in the Heart Outcomes Prevention Evaluation (HOPE) study (Yusuf et al. 2000; Williams 2000; Svensson et al. 2001). In the main study, the group receiving ramipril had approximately 35 % fewer cardiovascular events, despite an insignificant mean reduction in systolic blood pressure of 3 mmHg and in diastolic blood pressure of 2 mmHg (which can be written as 3 ⁄ 2 mmHg): the outcome benefit was attributed to angiotensin-converting enzyme inhibition, which was recommended in all high-risk individuals regardless of baseline blood pressure. However, it became evident from a later analysis of the ambulatory blood pressure monitoring substudy that ramipril was actually taken in the evening, while clinic blood pressure measurement occurred around 10–14 h later the following morning. The reported insignificant change in blood pressure in the main study gave no indication of a “whopping 17 ⁄ 8 mmHg reduction in BP during the evening hours,” which translated into a 10 ⁄ 4 mmHg average reduction over the entire 24-h period (O’Brien 2011b).
O’Brien and Turner (2013) commented on this case study as follows: “The HOPE study was designed to prove the beneficial effect of a drug on cardiovascular outcome, and were it not for the ambulatory blood pressure monitoring study, the interpretation of the results could have had a major impact on clinical practice.” It is not unreasonable to make the case that a similar misinterpretation could occur for blood pressure effects of a noncardiovascular drug if only routine blood pressure measurements during clinical trials were used to assess the drug’s effects.
11.5 What Might a Regulatory Landscape Look Like Here?
In an influential Letter to the Editor of the Drug Information Journal (now renamed Therapeutic Innovation & Regulatory Science), the question of “whether all systemically available drugs intended for chronic use merit a careful assessment of their effects on vital signs by [ambulatory blood pressure monitoring]” was raised (Stockbridge 2011). This question has since been discussed in various venues, including a CSRC Think Tank (Sager et al. 2013).
As noted in Sect. 11.1, as we write this chapter there is not a regulatory landscape in place addressing the prospective exclusion of unacceptable blood pressure increases. It is legitimate, however, to speculate what questions a regulatory guidance document would address. Perhaps the following are reasonable possibilities.
Is a pattern of change in both systolic and diastolic blood pressure more important than a (potentially greater) change in either one of them? While it can be reasonably argued that changes induced by drugs taken chronically are of greater concern than those induced by short-term pharmacotherapy, are issues of concern influenced by other variables such as sex and age? Is an increase of X mmHg from a baseline of Y mmHg more, less, or equally concerning than an increase of X mmHg from a baseline of Z mmHg? Is increase of X mmHg from a baseline of Y mmHg or Z mmHg more relevant in the nocturnal period (when risk of cardiovascular events is greatest) than in the daytime period (O’Brien and Turner 2013)?
These questions concern just systolic and diastolic blood pressure levels: we can also add questions concerning pulse pressure (the difference between systolic and diastolic blood pressures), blood pressure variability, and perhaps characteristics such as pulse wave velocity. As already noted, there are many mechanisms that can lead to blood pressure changes, so an additional question arises: is a blood pressure increase caused by mechanism A of more, equal, or less concern than a change of the same magnitude caused by mechanism B, C, or D? The answers to these questions are not readily apparent at this time.
11.5.1 Incorporation of Ambulatory Blood Pressure Monitoring
O’Brien and Turner (2013) offered some thoughts on the inclusion of ambulatory blood pressure monitoring in preapproval clinical development programs, noting that this blood pressure measurement modality provides powerful evidence concerning a drug’s blood pressure liability and that it should therefore be a central component of a “totality of evidence” approach: it facilitates assessments of both magnitude and duration of off-target blood pressure increases.
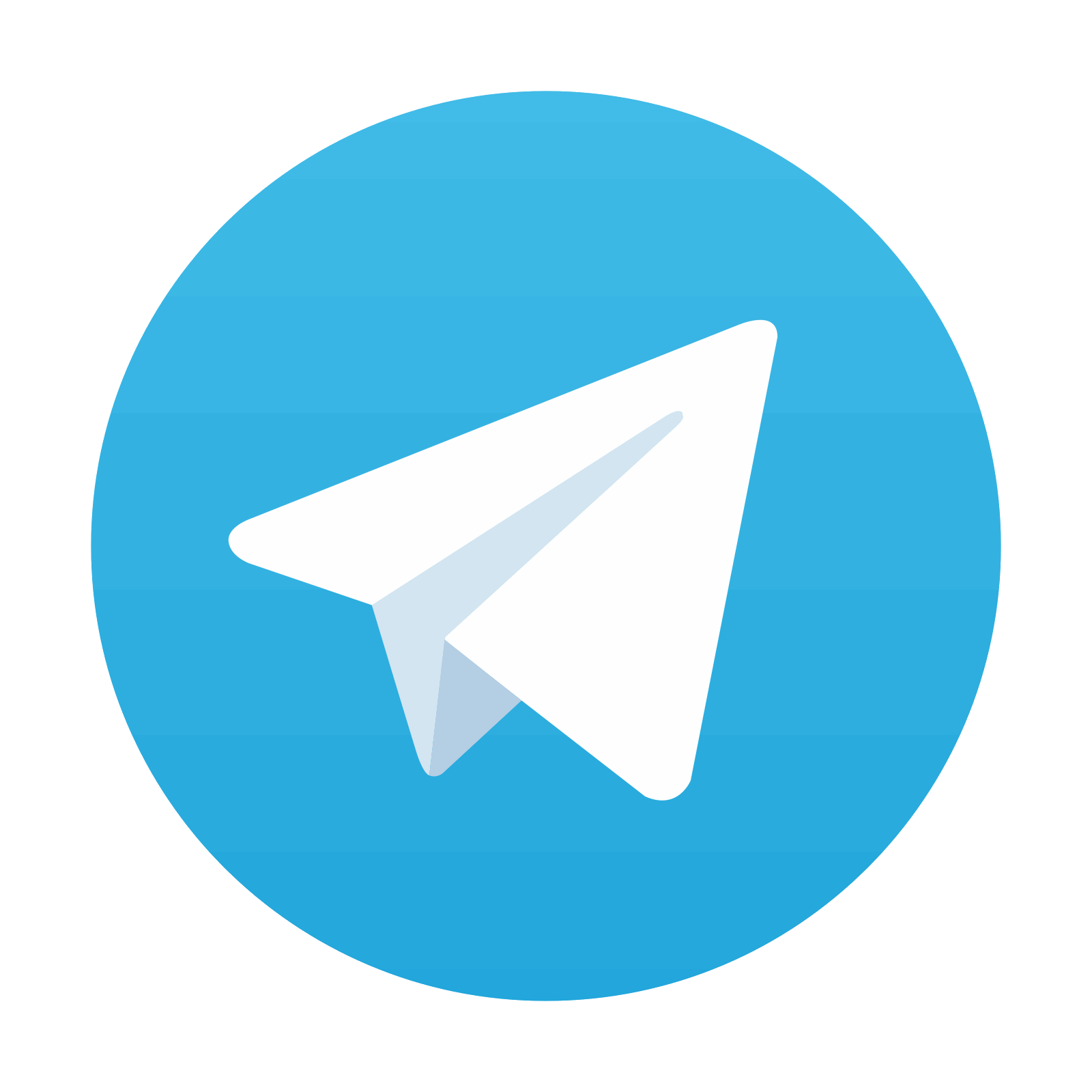
Stay updated, free articles. Join our Telegram channel

Full access? Get Clinical Tree
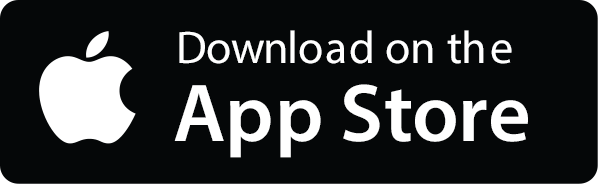
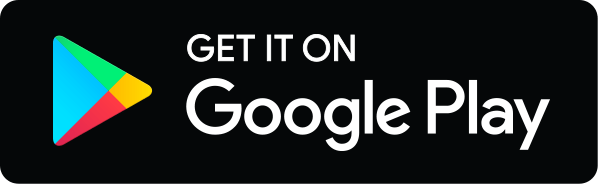