(1)
Project-team INRIA-UPMC-CNRS REO Laboratoire Jacques-Louis Lions, CNRS UMR 7598, Université Pierre et Marie Curie, Place Jussieu 4, 75252 Paris Cedex 05, France
Abstract
Leukocytes, red blood capsules, and platelets constitute the pool of circulating cells and pseudocells (Table3.1). Red blood capsules, commonly called erythrocytes or red blood cells, are mainly devoted to blood gas transport, especially oxygen. When the demand for oxygen changes, appropriate signals are detected and transmitted so that the supply of oxygen by the cardiorespiratory system varies to match the demand. Leukocytes, or white blood cells, coordinate the immune response. Platelets, also named thrombocytes, are cell fragments that participate in blood coagulation and inflammation.
Leukocytes, red blood capsules, and platelets constitute the pool of circulating cells and pseudocells (Table 3.1). Red blood capsules, commonly called erythrocytes or red blood cells, are mainly devoted to blood gas transport, especially oxygen. When the demand for oxygen changes, appropriate signals are detected and transmitted so that the supply of oxygen by the cardiorespiratory system varies to match the demand. Leukocytes, or white blood cells, coordinate the immune response. Platelets, also named thrombocytes, are cell fragments that participate in blood coagulation and inflammation.
Table 3.1
Blood cell approximated geometry and relative concentration.
Blood | Quantity | Relative | Size | Cell volume |
---|---|---|---|---|
cell | (/mm3) | proportion | (μm) | percentage |
RBC | 5 ×106 | 1 | ∼ 8 | 97% |
Leukocyte | 5 ×103 | 10 − 3 | ∼ 15 | 2% |
Platelet | 3 ×105 | 6 ×10 − 2 | ∼ 3 | 1% |
3.1 Immunophenotyping of Leukocytes
Molecules on the surface of hematopoietic cells act in the development and functioning of these cells. Leukocytes possess at their cell surface many accessory molecules that cooperate with antigen-specific receptors. They participate in cell adhesion between lymphocytes and other cell types or cell signaling. Numerous accessory molecules belong to the immunoglobulin (IGSF) superfamily.
Cell-surface antigens expressed on hematopoietic and nonhematopoietic cells include members of the cluster of differentiation (CD1–CD339) class, lymphocyte antigens (Ly1–Ly81), as well as markers that do not pertain to these molecule class.
Members of the cluster of differentiation class encompassG-protein-coupled,protein kinase, andcytokine receptors, ligands of theTNF superfamily, plasmalemmal carriers, cell adhesion molecules, erythrocytic antigens, etc.
Lineage markers are cell-surface antigens used for immunophenotyping cells of a particular developmental lineage. Cells that do not express given lineage markers, or express these markers at very low levels, are lineage marker negative (Lin − , i.e., in the mouse, CD2 − , CD3 − , CD4 − , CD5 − , CD8 − , CD161c − , PTPRc − , Ly76 − , Ly6g − ).
Hematopoietic stem cells, early progenitor cells, and precursor cells do not yet express certain markers. The LSK (Lin − , SCA1 + , KIT + ) phenotype defines a primitive cell type that possesses self-renewal capacity, from which multipotential cells MMP without self-renewal capacity develop.
Immunophenotyping consists in determining expression level of cell-surface molecules on leukocytes. A molecule + or molecule − term (plus or minus symbol) indicates whether a given molecule type is expressed or lacking on the plasma membrane of a given leukocyte type. Some leukocyte types are also defined as moleculehigh (or moleculebright), moleculemid (or moleculeint [intermediate]), or moleculelow (or moleculedim), according to the expression level, as well as moleculeneg∕low and moleculemid∕low for negative-to-low and medium-to-low phenotypes.
In addition, cells can be characterized by intracellular markers such as certain transcription factors as well as plasmalemmal markers only when they are activated (moleculeact).
3.2 Vasculature as an Immunity Compartment
The vasculature participates in the detection and protection against pathogens. Blood vessels convey immunocytes to identify, prevent eventual dissemination of invading bacteria to different organs, and eradicate these pathogens.
Intravascular motion of immunocytes, such asneutrophils,monocytes, andinvariant natural killer T cells, starts by transendothelial migration at target sites. However, many bacterial products antagonize leukocyte recruitment, such as lipopolysaccharide that can inhibit leukocyte displacement [97].
Endothelial cells contribute to innate immune responses to bacteria, as they express Toll-like receptors, lymphocyte antigen Ly96 homolog,1 and appropriate adaptors [97]. Activatednuclear factor-κB primes synthesis of adhesion molecule on endothelial cells that promotes neutrophil infiltration.
Neutrophils, major effector cells in innate immunity against bacteria, undergo platelet-dependent activation in the vasculature during bacteremia. They can form neutrophil extracellular traps that consist of chromatin coated with peptidases to trap and kill bacteria within the vasculature. Complement activation, specifically anaphylatoxin C5a, contributes to the recruitment of neutrophils in particular to the lung [97].
3.3 Proliferation and Mobilization of Blood Cells
Blood cell genesis, growth, proliferation in their bone marrow niches, and mobilization in the blood stream and in the body’s tissues are regulated by numerous substances (Vol. 2 –Chaps. 2. Cell Growth and Proliferation and 6. Cell Motility).
Blood cells need to communicate between them and with other body’s cells. They secrete cytokines that have a broad activity spectrum. Cytokines allow communications between immunocytes as well as between immunocytes and other cell types. They thus target manifold cell types. Chemokines are inducible chemotactic cytokines, which mobilize nearby responsive cells according to a chemotactic direction (Vol. 2 –Chap. 6. Cell Motility andChaps. 10 and 11).
Three main action modes are used via suitable receptors according to the length scale: (1) intracrine 2 and autocrine 3 mechanisms act on the producing cell without or after secretion, respectively; (2) juxtacrine 4 and paracrine 5 regulation happens on apposed and neighboring cells, respectively; and (3) endocrine 6 activity is exerted on remote cells.
3.3.1 Cytokines
Cytokines are growth factors implicated in immunity and hematopoiesis (Table 3.2;Chap. 2; Vol. 2 –Chap. 3. Growth Factors)). Cytokines exhibit both pleiotropy and redundancy. Pleiotropic actions of cytokines can be explained by the presence of receptors for a given cytokine on multiple cell lineages and the activation of multiple signaling pathways contributing to different functions. Overlapping actions of different cytokines can be explained by similar cellular distributions of specific receptors for different cytokines, as well as by share of signaling pathways. Moreover, cytokines can signal via several types of receptors and several cytokine types can bind to given receptors. Also, cytokine receptors have common structural components.7 Cytokines are downregulated by cytokine antagonists.
Table 3.2
Cytokines in immunity and hematopoiesis. Erythropoietin, thrombopoietin, growth hormone, and prolactin have structures and signaling mechanisms similar to those of interleukins and colony-stimulating factors, referred to as type-1 cytokines. Interferons are type-2 cytokines. Many cytokines are pleiotropic. Different cytokines have overlapping activities (TGFβ: transforming growth factor-β; TNFα: tumor-necrosis factor-α).
Immunity | Hematopoiesis |
---|---|
Interleukins | Interleukins |
TGFβ | Colony stimulating factors |
Interferons | |
TNFα | |
Chemokines |
After enzymatic cleavage of the extracellular domain of cytokine receptors, soluble cytokine receptor fragments in blood and extracellular fluid can bind to cytokines, thereby neutralizing their activity.
Mostcytokine receptors, as well as growth hormone8 and prolactin receptors of the immune and hematopoietic systems belong to the family of class-1 cytokine receptors. Most class-1 cytokine receptors are dimers, with a cytokine-specific and a signal-transducing subunit, which is usually not specific for the cytokine. Certain receptors are trimers.
Class-1 cytokine receptors are subdivided into subsets composed of receptors with identical signal-transducing subunits: (1) granulocyte–monocyte colony-stimulating factor (gmCSF) subset includes the receptors forIL3, IL5, and CSF2; (2) IL6 subset comprises the receptors for IL6, IL11, and IL12; and (3) IL2 subset encompasses the receptors for IL2, IL4, IL7, IL9, and IL15.
Ligands for class-2 cytokine receptors are interferons Ifnα, Ifnβ, and Ifnγ. The TNF receptor family includes TNF receptor-1 (TNFR1 or TNFRSF1a), TNF receptor-2 (TNFR2 or TNFRSF1b), TNFRSF5, and TNFRSF6a, among numerous other members (Vol. 3 – Chap. 11. Receptors of the Immune System). Certain cytokine receptors belong to the immunoglobulin superfamily, such as receptors for IL1 and macrophage colony-stimulating factor (mCSF or CSF1).
Signal transduction via class-1 and -2 cytokine receptors begins with cytokine-induced dimerization of receptor subunits. These cytokines use the JaK–STAT pathways. PlasmalemmalJanus kinases phosphorylate tyrosine residues ofsignal transducers and activators of transduction. These STAT factors then activate genes via transcription factors. The JaK–STAT pathway is also used by other growth factors and hormones.
3.3.2 Chemokines
Chemokines form a family of inflammatory inducible cytokines. Chemokines have potent chemotactic activity. Most of them belong to 2 main subsets according to their architecture, i.e., the presence of 4 cysteine (C) residues with first 2 adjacent (C–C) or separated by an amino acid (C–X–C). Chemokines of the CXC category (α-chemokines) are encoded by human chromosome 4q12-21, whereas chemokines of the CC category (β-chemokines) map to human chromosome 17q11-32.
Most CXC-chemokines are chemoattractants for neutrophils, whereas CC-chemokines generally attract monocytes, lymphocytes, basophils, and eosinophils. Two additional subsets are composed of C-chemokines, more or less lymphocyte specific, and CX3C-chemokines, which target leukocytes. Thechemokine receptors are composed of seven transmembrane proteins. They transduce signals via G-proteins. Chemokine receptors are classified into specific, shared, promiscuous, and viral groups.
3.4 Scaffold Proteins in Immunocytes
Signal transduction relies on location of signaling mediators inside the cell and their activation kinetics.Scaffold proteins bind to other signaling molecules to regulate signal transduction (Table 3.3), as they localize signaling effectors in specific regions within the cell to trigger and optimize signaling by acting as assembly platforms for enzymes, their regulators, and their substrates.
Table 3.3
Scaffold proteins in immune system signaling (Source: [99]; ASC: apoptosis-associated speck-like protein containing a caspase activation and recruitment domain [CARD]; BCL: B-cell lymphoma; CARMA: CARD–MAGUK domain-containing protein; CBL: Casitas B-lineage lymphoma; CDC: cell-division cycle; CTAK: CDC25c-associated kinase; DLg: disc large homolog; ERK: extracellular signal-regulated kinase; GSK: glycogen-synthase kinase; HSP: heat shock protein; IP3R: inositol trisphosphate receptor; IRAK: interleukin-1-receptor-associated kinase; JNK: Jun N-terminal kinase; KSR: kinase suppressor of Ras; MAGUK: membrane-associated guanylate kinase; MALT: mucosa-associated-lymphoid-tissue lymphoma-translocation gene; MAP2K: mitogen-activated protein kinase kinase; MyD: myeloid differentiation primary-response gene; NFκB: nuclear factor-κB; NIK: NFκB-inducing kinase, or MAP3K14; NLR: nucleotide-binding domain and leucine-rich-repeat-containing receptor; NLRP: NLR/pyrin-domain-containing; PLC: phospholipase-C; PKC: protein kinase-C; PP: protein phosphatase; RSG: regulator of G-protein signaling; S100: S100 calcium-binding protein; SMAD: small mothers against decapentaplegic homolog; STAT: signal transducer and activator of transcription; SuGT: suppressor of G2 allele of S-phase kinase-associated protein SKP1; TAK: TGFβ-activated kinase, or MAP3K7; TCR: T-cell receptor; TGF: transforming growth factor; TLR: Toll-like receptor; TNFR: tumor-necrosis factor receptor; TRAF: TNFR-associated factor; WASP: Wiskott-Aldrich syndrome protein; ZAP: ζ-chain-associated protein kinase).
Scaffold proteins | Binding partners |
---|---|
Ahnak1 | Calcium channels, caveolin-1, |
PKC, PLCγ, S100a | |
BCL10 | CARMA1, MALT1, TAK1 (MAP3K7), |
MAP2K7, JNK2 | |
DLg1 | CBL, LCK, Vav1, WASP, ZAP70, |
P38MAPK | |
Homer | IP3R, Homer, |
group-1 metabolic receptors | |
KSR | CDC37, CTAK1, PP2a, 14-3-3, HSP70, HSP90, |
Raf, MAP2K1, ERK | |
NLRP | ASC, HSP90, SuGT1, |
caspase-5, BCL2, BCLxL | |
Pellino | BCL10, IRAK1/4, MyD88, NIK (MAP3K14), |
(Ub ligase) | SMAD6, TAK1, TRAF6 |
Spinophilin | Factin, PP1, RGS |
(PP1r9) |
In immunocytes, scaffold proteins regulatecalcium signaling, activation ofmitogen-activated protein kinase modules, andcell polarity [99]. In T lymphocytes, calcium fluxes can oscillate repeatedly. Because scaffold proteins are able to coordinate positive and negativefeedback loops, they can induce oscillatory signaling patterns.
In the immune system, scaffold proteins include immunoreceptor tyrosine-based activation motif (ITAM)-containing receptors, such as T-cell and B-cell receptors, as well as cytoplasmic scaffold proteins, such as linker for activation of T cells (LAT), B-cell linker (BLnk),9 and lymphocyte cytosolic protein LCP2 [99].10 These scaffolds can be phosphorylated (hence their names “tyrosine phosphoproteins”).
Scaffold proteins that act inERK signaling compriseIQ motif-containing GTPase-activating protein-1 (IQGAP1), MAPK scaffold protein-1, Similar expression to FGF gene products (SEF), paxillin, β-arrestins, and kinase suppressor of Ras (KSR) [99].11
Scaffold B-cell lymphoma protein (BCL10) operates in theJNK pathway12 in immunocytes. Scaffold BCL10 participates in activation of nuclear factor-κB, in addition to JNK2, in the TCR axis. It complexes with mucosa-associated lymphoid tissue lymphoma translocation peptidaseMALT1 and CARMA1 to linkprotein kinase-Cθ withNFκB downstream from antigen receptors.
The scaffold Disc large homolog DLg1 intervenes with JNK-interacting proteins (MAPK8IP1–MAPK8IP4) inP38MAPK activation. This kinase is a sensor of pro-inflammatory stimuli primed by cytokines.
The AHNAK13 family of PDZ-containing scaffold proteins consist of 2 members:Ahnak1, or desmoyokin, and Ahnak2. Protein Ahnak1 is strongly expressed in spleen and lymph nodes, especially in splenic CD4 + T cells, but weakly in thymus [100]. In vitro, Ahnak1 fragments bind and activate phospholipase-Cγ1 in the presence of arachidonic acid. Partners of Ahnak1 include also calcium- and zinc-binding proteinS100b and the annexin-2–S100a10 complex. Incardiomyocytes, Ahnak1 associates with the β subunit of cardiac CaV channels at the plasma membrane.
Scaffold Ahnak in calcium signaling binds calcium channels,phospholipase-Cγ, andprotein kinase-C [99]. It is phosphorylated byprotein kinase-A in response to β-adrenoreceptor stimulation. Scaffold Ahnak participates in activation ofnuclear factor of activated T cells via calmodulin andPP3 phosphatase.
In T lymphocytes, scaffoldsHomer-2 and -3 inhibit nuclear factor of activated T cells by competing with PP3 phosphatase for NFAT binding.
Initiation of theToll-like receptor pathway involves recruitment of the adaptormyeloid differentiation primary-response gene MyD88 to liganded TLRs. Subsequently, activated IL1 receptor-associated kinases IRAK1 andIRAK4 prime formation of a complex that contains tumor-necrosis factor receptor-associated factor TRAF6, TRAF-interacting protein with forkhead-associated domain (TIFA), MAP3K7, and its binding partner MAP3K7IP1 to stimulate P38MAPKs, JNKs, and NFκB [99].
The PELLINO family includes 3 known proteins in humans that can associate with IRAK1, TRAF6, and MAP3K7 after IL1 receptor activation.
Members of the NLRP (NOD-like receptor [NLR] pyrin domain-containing) subfamily of the NLR family of cytoplasmic receptors of the innate immunity sense various pathogenic and chemical agents and trigger the formation ofinflammasomes. These NLRP scaffolds can bind adaptor and signaling proteins, such as apoptosis-associated speck-like protein containing a CARD (ASC), HSP90, suppressor of G2 allele of S-phase kinase-associated protein SKP1 (SuGT1), and caspase-5 [99]. The procaspase-1–NLRP1–ASC complex releases active caspase-1 that cleaves precursors of IL1β and IL18 interleukins.
Membrane-associated guanylate kinases (MAGuK) constitute a family of scaffolds that include Disc large homologs (DLg1–DLg4),14 zona occludens-1, CARMA (N-terminal caspase activation and recruitment domain [CARD]–MAGuK-like domain-containing), and MAGI1 (MAGuK-like, WW, and PDZ domain-containing with inverted domain organization protein-1) [99].Scaffold spinophilin, more precisely PP1r9 regulatory subunit (inhibitor) of PP1 phosphatase,15 is involved in dendritic cell functioning, as it can act in the formation of immunological synapses.
3.5 Red Blood Capsules
In the present textbook,erythrocyte, or red blood cell (RBC), is called red blood capsule, because it defines ahemoglobin (Hb) solution16 bounded by a thin flexible membrane (non-nucleated cell).
In its undeformed state, it has a biconcave disc shape with a greater thickness in its outer ring (diameter 7. 7 ± 0. 7 μm, central and peripheral thicknesses 1. 4 ± 0. 5 μm and 2. 8 ± 0. 5 μm, aspect ratio ∼ 0.4, surface ∼ 140 μm2, volume ∼ 90 μm3, membrane thickness ∼ 0.02 μm). It can then deform17 with a negligible bending resistance, in particular with a parachute shape when moving through tiny capillaries. Its mass density is equal to about 1100 kg/m3. It lives an average of 120 days. The RBC number is age- and sex-dependent (4.2–5.5 × 106/mm3 in women, and 4.5–6.2 × 106/mm3 in men).
3.5.1 Formation, Maturation, and Clearance
In adults, definitive erythropoiesis occurs in the bone marrow. Erythroblasts are associated with macrophages in anatomical units (blood islands). Nuclei are disconnected from reticulocytes, expelled, and then engulfed by macrophages. Phosphatidylserine, often used as an apoptosis signal, is also used for nucleus engulfment [102].
Enucleation results from asymmetrical cell division during which chromatin condensates and the nucleus surrounded by a part of the plasma membrane buds off from the immature precursor (erythroblast).MicroRNA miR191 that has minor effects on cell proliferation and differentiation is downregulated during this process [103]. In fact, the majority of microRNAs in CFUe erythroid progenitors are inhibited during terminal erythroid differentiation. In particular, miR144 and miR451, which are encoded byGATA1 target genes, are required for erythropoiesis, as they act on erythroidKrp̈pel-like factor KLF1 and GATA2, respectively. Gene transcripts that encode erythroid-enrichedRiO kinase RiOK3, which operates in chromatin condensation and enucleation, and class-C basic helix–loop–helix protein bHLHc11,18 a MyC antagonist, are miR191 targets.
Reticulocytes are slightly immature cells that are characterized by a filament network and by granules. Reticulocytes amount to 0.5 to 2% of the total RBC count (more in anemia).
In healthy human adults, ∼ 2.5 ×1011 new red blood cells are released from the bone marrow into the blood circulation per day, and about the same number are cleared. In the few days after exit from the bone marrow, erythrocytes undergo a reduction in both volume and hemoglobin content.
A model of RBC maturation and clearance has been developed [104]. This model relies on the splitting of volume (V ) and hemoglobin (Hb) dynamics of an average RBC over time into deterministic functions (ϕ) and random fluctuations (
), using the average cell age and population means to scale time, V, and Hb quantities. The deterministic component depends on 2 parameters related to fast (β) and slow (α) changes. The effect of parameter β dominates until the hemoglobin concentration is close to the mean corpuscular hemoglobin concentration (MCHC). The random fluctuation is modeled as a Gaussian random variable with a zero mean and diffusion (
)-dependent variance.


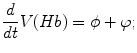
(3.1)
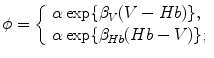
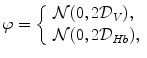
The dynamics of the complete circulating RBC population is described by an equation for time-dependent drift (
), diffusion (
), and birth (b) and death (d) rate of probability density of the joint volume-hemoglobin distribution (P(V, Hb, t)) that is approximated by a Fokker-Planck equation, using the differential vectorial operator ∇ [104]:

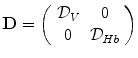



(3.2)
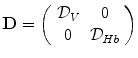
3.5.2 Shape
A red blood capsule experiences large reversible deformations during its 120-d lifespan. It recovers its initial shape after large deformations undergone in narrow capillaries owing to the composition of the membrane and membrane–cytoskeleton interaction. Its plasma membrane and the cell cortex, with thespectrin network tethered to the phospholipid bilayer, are the main load-bearing components. Membrane–spectrin interaction results from the phosphorylation of the interconnection protein-4.1R, or erythrocyte membrane protein band EPB4.1.
In thermal equilibrium, red blood cells, like lipid vesicles, can have various shape types according to the environmental conditions. The spectrin network enables RBCs to remain intact while deforming in blood flow through narrow capillaries. In normal conditions, RBCs adopt a biconcave disc shape with a given area (A)S and volume (V ; mean radius R m = (A/[4π])1 ∕ 2 ∼ 3.4 μm; reduced volume V/(4πR0 3/3) ∼ 0.6).
Membrane fluctuates over a short time scale ( ∼ 100 ms). Membrane fluctuations depend on its bending stiffness, ATP, and integrity of the spectrin cytoskeleton connected to the membrane by actin, glycophorin-C, and protein 4.1R. Phosphorylation of 4.1R protein byprotein kinase-C disassembles the EPB4.1–spectrin–actin trimer, hence spectrin–membrane connection. Inhibition and activation of this phosphorylation significantly affects tension and effective viscosity ( ∼ 100 × 10 − 3 Pa.s) [105].
In the microcirculation, RBC deformation reduces flow resistance. Deformation also provokesATP release from RBCs that requirescystic fibrosis transmembrane conductance regulator activated via the cAMP–PKA axis [106]. Release of ATP causesnitric oxide synthesis and augments the vascular caliber [107]. In addition, erythrocytes stimulate nitric oxide production inplatelets [108].
Spectrin tetramers in the unstressed state form dimers undershear. The cell periphery undergoes fluidization beyond a shear threshold [109]. Spectrins remodel into tetramers upon unloading and the RBC membrane stiffness rises. Stretching by optical tweezers estimates the shear modulus (4–10 μN/m) [110].
The RBC shape represents an equilibrium configuration that minimizes the curvature energy of a closed surface (a vesicle) for given surface area and volume with a geometrical asymmetry.19 Red blood cells respond toosmotic pressure (
) changes by swelling20 and shrinking (crenated RBC)21 in hypotonic and hypertonic fluids, respectively. RBC Membrane has thermal agitation. These spontaneous undulations are explained by the high membrane deformability. Furthermore, the membrane is excited by permanent impacts from water molecules.

Red blood cells defend against osmotic changes by controlling their intracellular ion content, especially keeping low [Na + ] i and high [K + ] i by the activities of the ATP-drivenNa + –K + pumps and ion exchangers. Hereditary stomatocytosis and spherocytosis are characterized by an uncontrolled excessive membrane permeability to Na + and K + associated with amino acid substitutions in the intramembrane domain of the anion exchangerSLC4a1 (or AE1) [112].
In narrow capillaries (conduit bore slightly > particle size), the 2 essential control parameters of the collective particle flow behavior are the hematocrit and flow velocity. At high H t values, 3 distinct phases can be predicted [113]: (1) disordered biconcave disc-shape; (2) parachute-shape, RBCs being aligned in a single file; and (3) slipper-shape, RBCs being arranged in 2 parallel interdigitated rows.
3.5.3 Plasma Membrane
The membrane is constituted by a phospholipid bilayer with protein inclusions, ion channels for chloride Cl − and bicarbonate HCO3 − in particular, and sialoglycopeptides (blood groups),22 with glucids ( < 10%), and with cytoskeleton meshing and mooring proteins. A peripheral skeleton, a mesh made of spectrin, actin, and other proteins (tubulin,23 among others), resides at the inner surface of the plasma membrane. It binds to RBC membrane (ankyrin–spectrin bonds) [114]. It gives the RBC shape. Large, repeated RBC deformation associated with maintenance of structural integrity is due to the cortical membrane-associated cytoskeleton.
3.5.3.1 Plasmalemmal Proteins
Transmembrane proteins include anion transporterSLC4a1;24glycophorin-A, -B, and -C; Rhesus blood group (Rh); Rh-associated glycoprotein (RhAG); Duffy;basal cell adhesion molecule (bCAM);25intercellular adhesion molecule ICAM4;26 heparan sulfate proteoglycanepican (Indian blood group; a.k.a. CD44); integrin-associated protein CD47; Kell blood group metalloendopeptidase; and X-linked Kx blood group (XK).
Erythrocyte markers Rh and RhAG could be gas transporter. In addition, RhAG and Rh form the Rh complex with CD47, LW, and glycophorin-B. Duffy serves as a chemokine receptor. Markers BCAM, ICAM4, and epican, are involved in cell adhesion. Kell blood group metalloendopeptidase has an endothin-3-converting enzyme activity.
Transporter SLC4a1 is the preponderant transmembrane constituent of the RBC membrane that operates as an anion exchanger. It binds protein-4.2, hemoglobin, glycolytic enzymes (aldolase, phosphofructokinase, and glyceraldehyde 3′-phosphate dehydrogenase), and carbonic anhydrase-2 to form themetabolon. Furthermore, SLC4a1 tetramer interacts with glycophorin-A to build the SLC4a1–ankyrin complex that is attached byankyrins to spectrin tetramers.
3.5.3.2 Connections between the Plasma Membrane and Cytoskeleton
The cell cortex lattice is composed ofspectrin tetramers formed by self-association of heterodimers made of α- and β-spectrin. Spectrin tetramers are attached to junctional complexes that comprise short Factin protofilaments and several actin-binding proteins, such as protein-4.1R, dematin (protein-4.9), adducin, tropomyosin, and tropomodulin.
Ankyrins link the spectrin–actin cytoskeleton to the membrane. Ankyrin deficiency is also known as hereditary spherocytosis. Ankyrins bridge the spectrin–actin cytoskeleton and SLC4a1 tetramers, as well as proteins involved in ion transfer, such asCl − –HCO3 − anion exchanger,Na + –Ca2 + exchanger,Na + –K + ATPase,IP3 receptor,ryanodine receptor–Ca2 + channel, andvoltage-gated Na + channels (NaV) [115].
Protein-4.1R, dimeric SLC4a1, adducin, spectrin, and actin form an additional multifunctional, multiproteic complex of the RBC membrane with transmembrane glycophorin-C, palmitoylated membrane protein MPP1,27 Rh, Duffy, Kell, and XK: the so-called 4.1R-based complex that surrounds connections between the cortical cytoskeleton and plasma membrane [116].
Plasma membrane deformability of red blood cells results from the coupling between the phospholipid bilayer and spectrin network.Adenosine triphosphate favors dynamical fluctuations in the RBC membrane [117]. Membrane vibrations (amplitude
[10 nm]) that use both metabolic and thermal energies are enhanced at the scale of spectrin mesh size to maintain the biconcave shape. Phosphorylation-dependent binding of the cytoskeleton to the plasma membrane can explain the correlation between dynamical fluctuations and biconcave shape. The spectrin mesh–membrane binding creates these dynamical vibrations via local remodeling of the spectrin junctions.

3.5.3.3 Pannexon and ATP Release
Erythrocytes expresspannexin-1 that forms a mechanosensitive, ATP-permeable channel (pannexon) in the plasma membrane [118]. These capsules releaseATP in response to hypoxemia (Sect. 9.10.9) as well asshear stress.
Adenosine triphosphate is an intra- and extracellular regulator. Red blood capsules succor blood pressure regulation, as they release ATP, a paracrine messenger that functions as a vasodilator, especially in arterial constrictions. It causes vasodilation by releasing nitric oxide from endothelial cells.
The release of ATP is delayed with respect to the onset of increased shear stress [119]. Remodeling of the spectrin–actin cytoskeleton network may launch the mechanosensitive (deformation-triggered) ATP release; a shear-dependent membrane viscosity may control the rate of release.
Flow-induced RBC deformation releases ATP that enables platelet adhesion to the endothelium. Adenosine triphosphate behaves as a platelet inhibitor below 0.16 amol per platelet;28 above this threshold, ATP is a platelet activator [120]. On the one hand, ATP stimulates the production of nitric oxide in endothelial cells via P2Y receptors and platelets; NO is vasodilator and platelet inhibitor. On the other, ATP activates platelet ATP-gated P2X1 ion channel, thereby causing a rapid Ca2 + entry that enables platelet aggregation. In particular, mild platelet stimulation with low concentrations of collagen rapidly releases ATP that primes the P2X1–Ca2 + –PKC–ERK2 pathway [121]. This event enhances further degranulation, thereby allowing complete platelet aggregation.
Proinsulin C-peptide increases glucose uptake by RBCs, intracellular Ca2 + concentration, and, subsequently, ATP release [122].29
Red blood capsules undergo tumbling, swinging, tank-treading, and deformation in the microvasculature.30 Tumbling-to-tank-treading transition, and not capsule deformation (i.e., stretching), contributes to the shear-thinning behavior (Vol. 7 –Chap. 5. Rheology) [126]. However, RBC deformation intervenes in ATP release, but not the transition from tumbling to tank-treading.
Both deformation-dependent and -independent ATP export occur through pannexin-1 hemichannel. Liberation of ATP through pannexin-1 mainly remains constant at shear stresses below a threshold (3 Pa), i.e., when RBC begins to deform [126]. Above this threshold, large RBC deformations occur and ATP release increases. Cystic fibrosis transmembrane conductance regulator contributes to deformation-dependent ATP release above the mechanical stress threshold. Large changes in local membrane curvature can dissociate spectrin from actin, thereby reducing the cell shear modulus and enabling actin binding to CFTR, hence its activation [127].
3.5.4 Influence of Oxygen Content in the Local Environment
Human RBCs circulate between lungs and tissues approximately every minute. In the lung, where O2 partial pressure is high, RBCs are exposed to oxidative stresses that must be controlled by accelerated production of reducing equivalents derived from the pentose phosphate pathway [128]. In tissues, where O2 partial pressure is low, deformed RBCs in capillaries undergo mechanical stresses that induce cation leaks. This leak and deoxygenation elevates glycolysis to produce ATP and restore intracellular ion balances and represses pentose phosphate pathway in RBCs.
Erythrocyte metabolism is regulated via the oxygen-dependent assembly of glycolytic enzymes into inhibitory complexes bound to the membrane anion transportSLC4a1 protein. In vivo, SLC4a1 acts as an oxygen-dependent metabolic regulator because it interacts with deoxyhemoglobin with high affinity, but not oxyhemoglobin [128]. Deoxyhemoglobin targets the same binding site on SLC4a1 as glycolytic enzymes. Therefore, upon deoxygenation, glycolytic enzymes are released and promote glycolysis. On the other hand, in oxygenated RBCs, glycolytic enzymes are inhibited, as they connect to SLC4a1 carrier.
3.5.5 Oxygen Sensor
The cardiovascular system responds to imbalances between oxygen supply and demand and adapts the blood flow. When oxygen demand changes, oxygen supply and/or extraction from circulating RBCs are adjusted to match the demand. Oxygen delivery is determined mostly by the vascular resitances of the arteriolar bed that irrigates the tissue of interest. Oxygen extraction occurs mainly in small arterioles and capillaries.
Erythrocytes have a dominant role in the supply of oxygen to tissues. Erythrocytic hemoglobin carries about 98% of blood oxygen. It releases oxygen to tissues owing to temperature, pH, and P
(that shift the oxygen dissociation curve).

According to the concept of metabolic control of the local blood flow, the concentration of vasodilators released from cells into the interstitial fluid that bathes resistance vessels results from the level of cell metabolism. Vasodilators, such as adenosine, nitrosothiols (e.g., Snitrosohemoglobin [HbSNO]) and nitrite, do not fill all criteria of a specific oxygen sensor [129].Nitric oxide can modulate both the supply of oxygen via its vasodilatory action on resistance vessels as well as the demand for oxygen via its inhibitory effect on oxygen consumption via cytochrome-C oxidase in mitochondria [129].
Erythrocytes can operate as mobile sensors for local oxygenation by the oxygen-dependent release ofATP vasodilator [130]. Insulin at normal concentrations inhibits the O2-dependent release of ATP from red blood cells, thereby impairing O2 delivery to fit the metabolic demand.
3.5.6 Blood Groups of the RBC Plasma Membrane
Erythrocyte surface contains proteins, glycoproteins, and glycolipids. Some define blood types. K. Landsteiner (1868–1943) described ABO blood types in 1900 from identification of blood agglutinins. Later (1937–1940), with A. Wiener, he worked on Rhesus blood group. The ABO and Rhesus blood groups are the main blood groups considered in transfusion. Many of the blood type antigens and corresponding antibodies have been discovered from transfusion incompatibilities. There are 30 identified blood groups with multiple distinct types. Some blood group molecules contribute to substance binding at the cell surface as well as chemical import or export.31
3.5.6.1 ABO Blood Group
The ABO blood group is related to the presence on the RBC plasma membrane (as well as on the surface of endothelial and most epithelial cells) of 2 monosaccharides at the termini of oligosaccharide chains on glycoproteins and glycolipids responsible for A- and B-antigen activity.32 Group A has anti-B antibodies that target B antigen; group B has anti-A antibodies; and group O has both as it is defined by the absence of A and B factors (i.e., group O red blood cells lack both A and B antigens). Group-O cells have at the termini of their oligosaccharide chains fucose (H antigen). Both A and B antigens are made from H antigen. The ABO blood group include subtypes A1, A1b, A2, and A2b. The Bombay blood group is related to rare Bombay phenotype (hh) characterized by the absence of H antigen.
3.5.6.2 Rhesus Blood Group
Five main Rhesus antigens are transmembrane proteins C, D, E, c, and e (genotype cde/cde – d means absence of D antigen – is RhD negative [RhD − ], whereas the others are RhD positive [RhD + ]).
3.5.6.3 Duffy Antigen–Chemokine Receptor
Duffy antigen (Dfy, GPFy, or CD234) is an acidic glycoprotein that is produced in greater amounts on reticulocytes than erythrocytes. Other isoforms can be detected in some epithelial cells (e.g., principal epithelial cells of collecting ducts in kidneys as well as type-1 epithelial cells of pulmonaryalveoli [131]), Purkinje cells of the cerebellum, andendothelial cells of capillaries (e.g., thyroid and kidney such as peritubular capillaries), postcapillary venules [132], and large pulmonary venules.
Duffy antigen receptor for chemokines (DARC) is targeted by members of both CC and CXC families (e.g., CCL2, CCL5, CXCL1, CXCL2, CXCL5, CXCL7, and CXCL8). However, DARC causes neither G-protein-coupled receptor signaling nor Ca2 + flux. The Duffy antigen receptor for chemokines (Darc or FY) gene (located on chromosome 1) encodes the Duffy blood group determinants that consist of 5 phenotypes (FyA, FyB, FyO, FyX, and FyY). Antigens FyA and FyB differ by a single amino acid (Gly43 and Asp43, respectively).
3.5.6.4 Diego Blood Group
The Diego blood group comprises 21 rare blood factors associated withchloride–bicarbonate exchanger (counter-transporter, also known as band-3 protein) that is encoded by theSLC4A1 gene (SLC: solute carrier family) on chromosome 17.
3.5.6.5 Kell and XK Blood Groups
The KEL gene encodes transmembrane glycoprotein CD238 or Kell protein. Several alleles exist, among which K1 (Kell) and K2 (Cellano) are the most common. The Kell protein binds to Xk protein that possesses the Kx antigen. The Kell blood group precursor (Kx) gene (XK) is located on X chromosome.
3.5.6.6 Kidd Blood Group
The Kidd blood group corresponds to an antigen on membrane of red blood cells as well as renal cells. It participates in urea transport. It is encoded by the JK gene (chromosome 18). Two common JK alleles are JKa and JKb. Human subjects with 2 JkA antigens (without JkB antigens) produce antibodies against JkB antigens.
3.5.6.7 Lewis Blood Group
The Lewis blood group relies on genes on chromosome 19. Two main types of Lewis antigens include Lewis-a and -b. Lewis antigens are released by exocrine epithelial cells and subsequently adsorbed onto RBC surface. Fucosyltransferase converts Lewis-a antigen into Lewis-b.
3.5.6.8 Glycophorin-Based MNS and Gerbich Blood Groups
The MNS blood group is based on 2 genes (on chromosome 4) that encodeglycophorin-A and -B that are associated with blood group MN and Ss antigens, respectively. More than 40 MNS antigens exist. The 5 most important MNS antigens are M, N, S, s, and U (U: universal, as it is extremely common).
The Gerbich (Ge) blood group is related to glycophorin-C33 encoded by a gene on chromosome 2 and its variant glycophorin-D. These glycophorins are unrelated to other glycophorins encoded by genes on chromosome 4 (loci 4q28-q31).
3.5.6.9 Miscellaneous
The Cartwright (Yt) blood group corresponds to acetylcholinesterase with 2 alleles, Yta and Ytb.
The Chido-Rodger (Ch/Rg) blood group is represented by 2 elements of the complement system: anaphylatoxin C4a and C3-convertase subunit C4b.
The Colton blood group is associated withaquaporin-1 with allelic diversity (Co-a and Co-b alleles). Aquaporin-3 is a determinant for the GIL blood group.
The Cromer blood group is related to decay accelerating factor (CD55) that prevents the assembly of the C3-convertase of the alternative pathway or accelerates the disassembly of preformed convertase.
The Dombrock blood group that comprises many variant alleles corresponds to ADPribosyltransferase Art4 (CD297) [133].
The Knops blood group squares with RBC complement receptor-1 (CR1, CD35, C3b/C4b receptor, and immune adherence receptor), a member of the the family of receptors of complement activation.
The Indian blood group (In) antigen tallies with glycoprotein CD44 that is involved in intercellular interactions.
The John Milton Hagen blood group (JMH) antigen coincides with semaphorin-7a that is expressed on RBCs and activated lymphocytes.
The OK blood group relies on glycoprotein CD147 that is also termed basigin and extracellular matrix metallopeptidase inducer.
The Ii antigen group corresponds to branched (I) or linear (i) polysaccharides, the synthesis of which depends on enzymes encoded by genes on chromosome 6. The unbranched i antigen, the precursor of I antigen, is synthesized by the sequential action of 2 glycosyl transferases, β(1,3)acetyl glucosaminyl transferase and β(1,4)galactosyl transferase. The I antigen is formed by branching initiated by a third transferase, the I β(1,6)Nacetylglucosamine transferase type 2. The GCNT2 gene encodes this Nacetylglucosaminyltransferase34 that converts active straight chains of carbohydrates to I-active branched chains. Three isoforms are created by alternative splicing (IGNT1–IGNT3 or IGNTa–IGNTc).
The i and I antigens are carbohydrate linear and branched repeats of Nacetyl lactosamine, i.e., n being the number of repeating lactosamine units (6 in average for i antigen, 8–25 for I antigen) and βi–j the linkage type: and
Gal | Gal | |||
| β1–4 | | β1–4 | |||
GlcNAc | GlcNAc | |||
| β1–6 | | β1–6 | |||
{ [Gal{ − − − − − − } {β1 − − 4} | GlcNAc ]} n – | –Gal{ − − − − − − } {β1 − − 4} | GlcNAc{ − − − − − − } {β1 − − 3} | Gal– |
{ [Gal{ − − − − − − } {β1 − − 4} | GlcNAc ]} n – | –Gal{ − − − − − − } {β1 − − 4} | GlcNAc{ − − − − − − } {β1 − − 3} | Gal– |
Adult human RBCs mostly express I antigens and contain only a few i antigens, whereas the latter predominates in fetal and neonatal erythrocytes. After birth, the quantity of I antigens gradually rises and the amount of i antigens falls; adult values are reached about at month 18.
The Raph blood group antigen dovetails with transmembrane glycoprotein GP27, a.k.a. platelet-endothelial tetraspan antigen PETA3, CD151, and tetraspanin-24.
The Scianna (Sc) blood group is determined by erythroblast membrane -associated protein (ERMAP) that is encoded by the Ermap gene.
The Landsteiner-Wiener (LW) blood group has been renamed intercellular adhesion molecule ICAM4.
The Lutheran (Lu) blood group with antigens LuA and LuB is based on genes on chromosome 19.
P blood group components (Pl) are encoded by genes on chromosome 22.
The XG blood group is encoded by the PBDX gene on X chromosome.
Other blood group antigens include globoside, a glycosphingolipid also named erythrocyte P antigen, and Rh-associated glycoprotein, a putative gas channel protein.
3.5.7 Hemoglobin Solution in Erythrocytic Capsule
Each RBC contains about 280 ×106 hemoglobin molecules [134]. Hb consists of four globin chains α and β. It contains four iron atom Fe2 + , in the center ofhemes.35 Hemoglobin carries oxygen (O2) from lungs to tissues and help to transport carbon dioxide (CO2) from tissues to lungs.36 Hemoglobin is also involved in pH regulation.
Hemoglobin is the iron-containing, oxygen-transport metalloprotein in solution in the RBC cytoplasm ( ∼ 97% of RBC dry content; ∼ 35% of total content). This tetramer contains 2 copies of 2 distinct globin types (α and β; hemoglobin-A; α2β2) and 4 binding sites, one per globin. Hemoglobin tetramer is a ellipsoidal protein (6.4 ×5.5 ×5.0 nm).
Hemoglobin carries 97 to 98% of oxygen to cells for metabolism. Hemoglobin oligomerization enables cooperativity between multiple binding sites (allostery). Binding of a first O2 molecule to any heme induces a shift in the corresponding F-helix. This structural change decreases the binding energy for subsequent O2 binding in the other 3 subunits (cooperative binding).
Oxyhemoglobin is formed when oxygen binds to Hb heme in RBCs of the pulmonary capillaries running along pulmonary alveolus wall. Deoxyhemoglobin corresponds to hemoglobin that has released its oxygen. Oxyhemoglobin and deoxyhemoglobin differ by their absorption spectra.37
3.5.7.1 Hemoglobin–Oxygen Association and Dissociation
Oxygen saturation is a relative measure of the amount of oxygen that is dissolved or carried in a given medium. Oxygen saturation in blood (
) yields the percentage of hemoglobin binding sites occupied by oxygen.

Functional oxygen saturation refers to the ratio of oxyhemoglobin to all types of functional hemoglobin:
oxyhemoglobin/(oxyhemoglobin + deoxyhemoglobin).
Fractional oxygen saturation is the ratio of oxyhemoglobin to the total amount of hemoglobin that is able to carry oxygen, including dyshemoglobins:
oxyhemoglobin/(oxyhemoglobin + deoxyhemoglobin + carboxyhemoglobin
+ methemoglobin).
Hemoglobin–oxygen association–dissociation curve has a sigmoidal shape that results from cooperative binding of oxygen to hemoglobin. At low partial pressures of oxygen (
), most hemoglobin is deoxygenated. When oxygen partial pressure reaches about 10 kPa, blood oxygen saturation approaches 100%.38

3.5.7.2 Hill Model
Measure of cooperativity of O2 binding relies on oxygen saturation of hemoglobin that is determined by measurement of the partial pressure of oxygen in solution using Hill cooperativity coefficient n (oxygen concentration is proportional to partial pressure). Because the hemoglobin saturation curve does not satisfy the hyperbolic relationship observed for myoglobin:
(p (50): oxygen partial pressure of O2 at which half of Hb molecules are in the oxy state), Hill assumed the following equilibrium in 1913:
The fractional saturation for the hypothetical reaction is given by:
![$$S_{\mathrm{O}_{2}} = \frac{[Hb(O2)n]} {[Hb(O2)n] + [Hb]} = \frac{p_{\mathrm{O}_{2}}^{n}} {p_{\mathrm{O}_{2}}^{n} + p_{(50)}^{n}}.$$](/wp-content/uploads/2016/09/A216847_1_En_3_Chapter_Equ3.gif)

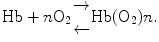
![$$S_{\mathrm{O}_{2}} = \frac{[Hb(O2)n]} {[Hb(O2)n] + [Hb]} = \frac{p_{\mathrm{O}_{2}}^{n}} {p_{\mathrm{O}_{2}}^{n} + p_{(50)}^{n}}.$$](/wp-content/uploads/2016/09/A216847_1_En_3_Chapter_Equ3.gif)
(3.3)
Hill plot corresponds to the trace of
(i.e., ratio of oxyHb to desoxyHb) vs.
. At small and large values of
, n = 1, whereas in the transition region of the relationship, n = 2. 8.
![$$\log [S_{\mathrm{O}_{2}}/(1 - S_{\mathrm{O}_{2}})]$$](/wp-content/uploads/2016/09/A216847_1_En_3_Chapter_IEq22.gif)
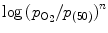

In tissues, β chains very easily lose O2, but α chains tightly hold oxygen. Diphosphoglycerate (DPG) of the anaerobic glycolysis pathway used by RBCs that lack mitochondria to produce enough ATP binds to Hb between 2 deoxygenated β chains. Conformational change that results promotes oxygen release from α chains. In lung capillaries, (2,3)-DPG is expelled from Hb molecules.39
3.5.7.3 Haldane and Bohr Effects
The Haldane effect states that deoxygenated Hb has a greater affinity for CO2 than does oxyhemoglobin. Oxygen loading and unloading assist CO2 release and pickup in lungs and tissues, respectively, and conversely.
The Bohr effect states that deoxygenated Hb has a greater affinity for H + than does oxyhemoglobin. Oxygen loading and unloading assist H + delivery and capture in lungs and tissues, respectively, and conversely.
The Bohr and Haldane effects are allosteric effects caused by binding of different ligands at different Hb spots that generates Hb conformational changes, thereby increasing and decreasing Hb affinity for O2 in lung and the body’s tissues, respectively.
3.5.7.4 Heme
Hemoglobin is a large protein, but its active site is a small non-proteic heme. Theheme consists of a flat porphyrin ring (4 nitrogen-containing pyrrole groups bound by methyl bridges) based on building block porphyrin. Each heme group binds a central ferrous iron ion (Fe2 + ) to become the so-called hemin (
–heme).40

Heme is an iron-containing porphyrin that serves as prosthetic group in hemeproteins. These hemeproteins are involved in: (1) oxygen binding (hemo- and myoglobin); (2) oxygen metabolism (oxidases, peroxidases, catalases, and hydroxylases); (3) electron transfer (cytochromes); and (4) signal transduction (nitric oxide synthases). This signaling messenger operates in erythropoiesis, globin synthesis, microRNA processing, and circadian rhythm regulation.
Heme binds reversibly to the nuclear receptor NR1d141 a heme sensor that coordinates the cellular clock, glucose homeostasis, and energy metabolism [136]. Transcription factor NR1d1 inhibits the circadian core clock and hepatic gluconeogenic gene expression.
In addition, the signaling mediator heme inactivates transcriptional repressors such as BTB and CNC homology-1, basic leucine zipper transcription factorBACH1.
Free heme is toxic. Therefore, its intracellular concentration is carefully regulated to yield a proper supply, but avoid heme toxicity. The rate of heme synthesis varies significantly among cell types. It is high in hepatocytes and erythroid cells, in which large amounts of heme are needed for cytochrome-P450 and hemoglobin, respectively. Heme is degraded by heme oxygenases to biliverdin, which is then converted to bilirubin by biliverdin reductase. Excess heme increases the transcription of heme oxygenase-1.
In addition, intracellular heme can be exported by plasmalemmal proteins,feline leukemia virus subgroup-C receptors (FLVCR1). This cytosolic heme export protein carries cytoplasmic heme as well as other cyclic planar porphyrins, such as protoporphyrin-9 and coproporphyrin, but not bilirubin.42
The FLVCR receptor is required for terminal RBC development [139]. It is also involved in proerythroblast survival. It facilitates macrophage recycling of heme iron [140]. Moreover, FLVCR intervenes in the recycling of heme iron from senescent RBCs.
The export of heme is preferentially boosted byhemopexin [140]. Hemopexin abounds in serum (concentration 6–25 μmol; 0.4–1.5 mg/ml in humans). It accepts heme from FLVCR and carries it to the liver, thereby ensuring iron homeostasis, in addition to its scavenger role during internal bleeding and hemolysis.
Heme synthesis must be coordinated with translation of globin proteins to avoid toxic accumulation of hemoglobin precursors. Heme synthesis begins and ends in mitochondria, but several intermediate steps happen in the cytoplasm. Hemoglobin precursors are then transported across the mitochondrial membrane. The terminal step of heme synthesis in mitochondria corresponds to insertion of an imported iron atom into protoporphyrin-9. It is catalyzed by mitochondrial ferrochelatase, an integral protein of the inner mitochondrial membrane.
Transporter SLC25a37, ormitoferrin (Mfrn1), transfers ferrous iron (Fe2 + ) across the mitochondrial membrane.The ATP-binding cassette transporter ABCb10 stabilizes the mitochondrial iron transporter SLC25a37 at least in erythroid precursors [141]. Both SLC25a37 and ABCb10 not only can homodimerize, but also complex with other partners. The SLC25a37–ABCb10 complex yields iron to ferrochelatase, thereby allowing erythroid precursors to accelerate their production of heme. Ferrochelatase, or protoheme ferrolyase, catalyses the terminal step (stage 8) of heme synthesis, converting protoporphyrin-9 into heme. Ferrochelatase is a partner for both SLC25a37 and ABCb10 transporters that forms the Fech–SLC25a37–ABCb10 complex [142]. Iron import in mitochondria is thus synergistically integrated with heme synthesis.
3.5.7.5 Functional and Dysfunctional Hemoglobin Types
Hemoglobin has many functional and dysfunctional types (Tables 3.4 and 3.5). The globin gene loci comprise mainly the α-globin locus on chromosome 16 and β-globin locus on chromosome 11. About 400 alteration types of hemoglobin genes have been detected.
Table 3.4
Human hemoglobin (HB) genes and its products (Hb). The genes in the α-globin locus consist of the transiently expressed (the first 12 weeks after conception) embryonic gene HBZ followed by HBM, HBA2, HBA1, and HBQ1 genes (from 5′to 3′end). The HBQ1 gene is expressed very early in embryos erythroid cells, but not in adult erythroid or other non-erythroid cells. The θ-globin family consists of the HBQ1 gene that encodes θ1-globin and at least a truncated, processed ψθ2 pseudogene located on chromosome 22, whereas HBZP (ψζ1) and HBAP2 (ψα2) pseudogenes localize downstream from the HBZ (ζ2) gene, and HBAP1 (ψα1) pseudogene downstream from the HBM gene. The genes in the β-globin locus are also arranged sequentially (HBE1, HBG2, HBG1, HBD, and HBB from 5′to 3′end), beginning with the gene expressed during embryogenesis and ending with the gene expressed in adults. The 2 HBG genes that produce hemoglobin-F are active during fetogenesis.
Gene | Protein | |
---|---|---|
α-Globin locus on chromosome 16 | ||
HBZ | Hemoglobin-ζ, ζ-globin | |
HBM | Hemoglobin-μ, μ-globin | |
HBA2 | Hemoglobin-α2, α2-globin, HbH | |
HBA1 | Hemoglobin-α1, α1-globin, CD31, HbH | |
HBQ1 | Hemoglobin-θ1, θ1-globin | |
β-Globin locus on chromosome 11 | ||
HBE1 | Hemoglobin-ε, ε-globin | |
HBG2 | Hemoglobin-γ2(G), γ2-globin, Gγ-globin (Paulinia) | |
methemoglobin | ||
HBG1 | Hemoglobin-γ1(A), γ2-globin, Aγ-globin | |
HBD | Hemoglobin-δ, δ-globin | |
HBB | Hemoglobin-β, β-globin |
Table 3.5
Functional and dysfunctional types of hemoglobins (FHQTL: fetal hemoglobin QTL on chromosome 8).
Category | Types | Tetramer composition |
---|---|---|
Developmental stages | ||
Embryo | Gower 1 | ζ2ε2 |
Gower 2 | α2ε2 | |
Portland | ζ2γ2 | |
Fetus | Hemoglobin-F | α2γ2 |
( ≥ week 9) | FHQTL | |
Adult | Hemoglobin-A (A1) | α2β2 |
Hemoglobin-A2 | α2δ2 | |
Variants responsible for hemoglobinopathies | ||
Hemolytic anemia | Hemoglobin-C | α2δ ![]() |
Hemolytic anemia | Hemoglobin-E | α2δ ![]() |
α Thalassemia | Hemoglobin-H | β4 |
α Thalassemia | Hemoglobin Barts | γ4 |
Sickle cell disease | Hemoglobin-S | α2δ ![]() |
Synthesis of δ chain begins late in the third trimester in utero. In the first 8 weeks, majority of the hemoglobin is embryonic hemoglobin (hemoglobin-E). In the embryo, hemoglobin variants comprise hemoglobin Gower-1 (ζ2ε2) and Gower-2 (α2ε2), as well as hemoglobin Portland (ζ2γ2). In the fetus, fetal hemoglobin, or hemoglobin-F (α2γ2), is produced. In newborns, hemoglobin-F is nearly completely replaced by adult hemoglobin in week 12 of postnatal life. Fetal hemoglobin binds oxygen with greater affinity than adult hemoglobin.
In adults, hemoglobin-F is restricted to a limited population of F reticulocytes and erythrocytes. Hemoglobin-A2 (α2δ2) is an adult variant (1.5–3.5%).
In humans, hemoglobin variants include variants of α-, β-, γ-, and δ-globin chains. An elevated proportion of hemoglobin-F and other variants, such as hemoglobin-S (α2β
) and -C (α2β
), correspond to hemoglobinopathies.


Thalassemia is caused by hemoglobin variants (deletional and non-deletional forms of gene mutations). Thalassemia has most often an autosomal recessive pattern of inheritance, both parents being unaffected carriers of a hemoglobinopathy trait.
α-Thalassemia results from deletions of one or both HBA1 and HBA2 genes. The − α determinant is associated with many different Hb variants (Table 3.6). Non-deletion mutations that are mainly associated with the HBA2 gene, affecting mRNA translation and processing, or causing post-translational instability, also generate α-thalassemia.
Variant | Amino acid subtitution in α-globin |
---|---|
Hb Evanston | Trp14 ![]() |
Hb Hasharon | Asp47 ![]() |
HbG Philadelphia | Asn68 ![]() |
HbQ Mahidol | Asp74 ![]() |
Hb Duan | Asp75 ![]() |
Hb Nigeria | Ser81 ![]() |
HbJ Capetown | Arg92 ![]() |
HbJ Tongariki | Ala115 ![]() |
Hemoglobin Bart’s hydrops fetalis syndrom is one of the major hemoglobinopathies responsible for α thalassemia. α Thalassemia can indeed cause hydrops fetalis when all 4 of the genetic loci for α-globin are deleted or affected by mutation. Hydrops fetalis is characterized by edema in at least 2 fetal compartments (hydrops allantois and hydrops amnion correspond to fluid accumulation in the allantoic and amniotic space respectively). Thalassemia can coexist with other hemoglobinopathies.
The most severe case of α-thalassemia is characterized by a defective or or lacking production of α-globin chains from the HBA1 and HBA2 genes, which encode hemoglobin α1 and α2 chains. Hemoglobin Barts (Bartholomew) consists of 4 γ chains. It has a very high affinity for oxygen, thus hampering oxygen delivery to cells.
Hemoglobin-H (HbH) is a β-chain tetramer. The excess β chains form unstable tetramers, which have abnormal oxygen dissociation curves. It has a higher affinity for oxygen than normal hemoglobin, thereby delivering a low quantity of oxygen to cells.
Inherited β-thalassemia is characterized not only by a reduced or absent synthesis of hemoglobin β chain, but also an elevated HbF concentration. In thalassemia minor, a single β-globin allele (among 2 alleles) bears a mutation that causes microcytic anemia; in thalassemia major, both alleles undergo mutations.
Sickle cell anemia, or drepanocytosis, is an autosomal recessive genetic disorder. The HBB gene that encodes 1 of the 2 types of polypeptidic chains of adult tetrameric hemoglobin is responsible for sickle cell anemia, characterized primarily by chronic anemia (sickled RBCs lifespan 10–20 d). Patients inherit either 2 copies of HBS variant or 1 copy of HBS and 1 copy of another variant. The substitution of amino acid in position 6 (hydrophobic amino acid valine rather than hydrophilic glutamic acid) creates a hydrophobic spot on the outside of the protein structure that links to the hydrophobic region of an adjacent hemoglobin β chain. This polymerization of HbS molecules that creates rigid structures causes the “sickling” of RBCs.43 Several hundred HBB gene variants are known.
The hemoglobin variant — hemoglobin-S (HbS) — is the most common cause of sickle cell anemia, in the case of homozygosity of a mutation in the HBB gene. In heterozygous subject with a single sickle (S) gene and a normal adult hemoglobin (A) gene (HbAS; i.e., hemoglobin genotype AS rather than normal hemoglobin genotype AA), the abnormality is called sicklemia or sickle cell trait, without disease symptoms (healthy carrier subjects).
Hemoglobin-C results from a variation in the HBB gene. In homozygotes, nearly all Hb is HbC that causes a mild chronic hemolytic anemia. A heterozygous form with one sickle gene and another encoding hemoglobin-C, i.e., the gene for HbS inherited from one parent and the gene for HbC inherited from the other parent, causes hemoglobin-SC disease.
Like hemoglobin-S and -C, hemoglobin-E is generated by mutations of the HBB gene. This variant causes a mild chronic hemolytic anemia.
Dyshemoglobins (dysfunctional Hb) are also caused by molecules that affect binding of oxygen to Hb by competing with oxygen at heme, such ascarbon monoxide (CO) that leads to carboxyhemoglobin (COHb; Table 3.7). Hemoglobin binding affinity for CO is 200 times greater than its affinity for oxygen. The stable complex COHb (blood half-life 4–6 h) does not release carbon monoxide. Therefore, hemoglobin cannot transport oxygen from lungs to the body’s cells. Tobacco smoking via carbon monoxide inhalation augments the blood COHb concentration. However, CO, a element of the gaseous triumvirate with nitric oxide (NO) and hydrogen sulphide (H2S), is a gasotransmitter (Vol. 4 – Chap. 10. Other Major Signaling Mediators) with anti-inflammatory, vasodilatory, and pro-angiogenic effects. Moreover, CO and NO are volatile and inhibitory coneurotransmitters [144].
Table 3.7
Functional and dysfunctional types of hemoglobin compounds. A single hemoglobin molecule can transport 4 oxygen molecules. Each erythrocyte contains about 3 × 108 hemoglobin molecules. The affinity of hemoglobin for oxygen depends on 4 primary regulators, CO2, hydrogen ion (H + ), chloride ion (Cl − ), and (2,3)-bisphosphoglycerate (BPG). Hemoglobin can bind, in particular, protons and CO2 that cause a Hb conformational change and support 02 release. In lung capillaries, H + and CO2 are liberated from hemoglobin. Higher BPG concentrations in blood enables the delivery of larger O2 amounts to cells under smaller blood O2 content (high altitude). Normally 1 to 2% hemoglobin is methemoglobin. NADH-dependent methemoglobin reductase converts methemoglobin to hemoglobin. Hemoglobin has a competitive binding affinity for cyanide that contains the cyano group (-CN − ), sulfur monoxide (SO), nitrogen dioxide (NO2), and sulfide (S2 − ), including hydrogen sulfide (H2S), that bind to iron (Fe2 + ) in heme and prevent O2 binding. Cyanide is a natural, potent cellular toxin. Acute cyanide toxicity results from accidental exposure or iatrogenic toxicity. Sulfide is a sulfur dianion in its lowest oxidation state. Hydrogen sulfide is a very toxic gas and yet another natural messenger.
Type | Associated compound |
---|---|
Functional hemoglobin types | |
Carbaminohemoglobin | Carbon dioxide |
( ![]() | Lowers hemoglobin affinity for 02 (Bohr effect) |
CO2 + Hb ![]() ![]() ![]() | |
Oxyhemoglobin | Oxygen |
( ![]() | Taut and relaxed forms have low and high |
oxygen affinity, respectively | |
Glycated hemoglobin | Glucose |
Nitrosyl hemoglobin | Nitric oxide |
(Hb ![]() | |
Nitrohemoglobin | Nitric oxide (S-nitrosylation) |
or nitrosohemoglobin | |
(HbSNO) | |
Dysfunctional hemoglobin types | |
Carboxyhemoglobin | Carbon monoxide (inhaled or metabolic product) |
(COHb) | Stable complex |
Cyanohemoglobin | CN − anion (via Fe2 + ) |
Unability to transport oxygen | |
Methemoglobin | Ferric rather than ferrous ion |
![]() | Cannot bind oxygen |
Sulfhemoglobin | Sulfur atom upon combination H2S and Fe3 + |
(SHb) | Usually caused by drugs |
Methemoglobins (MetHb) are caused by iron oxidation (Fe 3 + ) in Hb heme. Methemoglobin is unable to carry oxygen. NADH-dependent methemoglobin reductase converts methemoglobin to hemoglobin. Nitrogen dioxide (NO2) and nitrous oxide (N2O) can convert a small fraction of hemoglobin to methemoglobin. Normally, only 1 to 2% of hemoglobin is methemoglobin.
Other molecules compete to bind hemoglobin, such as cyanide (CN − ), sulfur monoxide (SO), nitrogen dioxide (NO2), sulfide (S2 − ), and hydrogen sulfide (H2S).
3.5.7.6 Nitric Oxide Carrier
Hemoglobin also carriesnitric oxide that improves oxygen delivery in the peripheral tissues and contributes to the control of respiration. Nitric oxide binds reversibly to a specific cysteine residue (highly conserved Cys93 in all mammals) in globin β chain. S-nitrosylated hemoglobin influences NO-mediated regulation of vascular resistances, blood pressure, and ventilation. Nitric oxide is transported by an anion exchangerSLC4a1 out of RBCs that then dispense NO activity.
Nitric oxide undergoes oxidative and reductive reactions to produce various chemical species, such as nitrite (NO
), nitrosothiols, nitrosoamines, nitrated lipids, and iron nitrosyl complexes. It is scavenged by reactions with hemoglobin in RBCs to form nitrate (NO
), methemoglobin (
Hb), and ferrous nitrosyl hemoglobin (
HbNO) after the reduction of nitrite by deoxyhemoglobin to NO and reaction of NO with deoxyhemoglobin (nitrite–deoxyhemoglobin reaction) [145]:

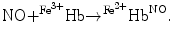
On the other hand, nitrate formation in RBCs results from the reaction of nitrite with oxyhemoglobin (
Hb; nitrite–oxyhemoglobin reaction) [145]:
![$$4{\bigl [\mathrm{NO}_{2}^{-} {+ }^{\mathrm{O}_{2} }\mathrm{{Hb}}^{2+} +\mathrm{ {H}}^{+}\bigr ]} \rightarrow4\bigl [\mathrm{NO}_{ 3}^{-} + {4}^{{\mathrm{Fe}}^{3+} }\mathrm{Hb} +\mathrm{ O}_{2} + 2\mathrm{H}_{2}\mathrm{O}.$$](/wp-content/uploads/2016/09/A216847_1_En_3_Chapter_Equ6.gif)
In addition, nitrite can bind to methemoglobin to produce a nitrite-bound methemoglobin intermediate that can interconvert to a ferrous–nitrogen dioxide species, which reacts with NO to form ferrous hemoglobin and dinitrogen trioxide (N2O3) [145]:
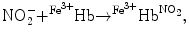

The overall nitrite–deoxyhemoglobin reaction then becomes [145]:






(3.4)
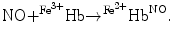
(3.5)

![$$4{\bigl [\mathrm{NO}_{2}^{-} {+ }^{\mathrm{O}_{2} }\mathrm{{Hb}}^{2+} +\mathrm{ {H}}^{+}\bigr ]} \rightarrow4\bigl [\mathrm{NO}_{ 3}^{-} + {4}^{{\mathrm{Fe}}^{3+} }\mathrm{Hb} +\mathrm{ O}_{2} + 2\mathrm{H}_{2}\mathrm{O}.$$](/wp-content/uploads/2016/09/A216847_1_En_3_Chapter_Equ6.gif)
(3.6)
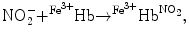
(3.7)

(3.8)

(3.9)
Nitrite can quickly enter RBCs (rate constant 0.25 /mn) [145]. With a nitrite dose of 800 μmol, erythrocytic uptake rate is approximately 4-fold higher than that in vitro. Iron nitrosyl hemoglobin is rapidly formed. The overall consumption of ferrous nitrosyl hemoglobin is fast in comparison with elimination of methemoglobin and plasma nitrate (elimination time ∼ 40, 105, and 300 mn, respectively).
In pulmonary arteries, hypoxemia normally causes vasoconstriction to match perfusion to ventilation.Nitric oxide synthases and Snitrosoglutathione reductase in RBCs determine intraerythrocytic content in nitric oxide andnitric oxide-thiol (Snitrosothiol). Normal erythrocytic ratio of SNO to hemoglobin is lower than 1:1000 [146]. Snitrosohemoglobin (HbSNO) deficiency aggravates hypoxemia-mediated pulmonary hypertension [147, 148]. However, individuals who are heterozygous for a βCys93 mutation are phenotypically silent (without clinical abnormality) [149].
3.5.8 Iron Acquisition and Storage
Iron is needed by iron-dependent enzymes. Most of body’s iron is found as heme in red blood cells. Iron can easily gain and lose electrons. This property is used not only by hemoglobin, but also by myoglobin, cytochromes, and various enzymes. However, it generates superoxide anions and hydroxyl radicals by electron donation to oxygen. Because reduced iron can catalyze formation of reactive oxygen species within cells, cells control the cytosolic amount of iron. Nevertheless, intracellular iron must be delivered to sites of storage and use, particularly target proteins, such as enzymes and carriers. Iron delivery is achieved by iron chaperones such as poly(rC)-binding protein-1 (PCBP1), which delivers iron to iron storage proteinferritin [150]. Ferritin can store up to 4,500 atoms of iron.
Iron balance is maintained between regulated absorption of dietary iron, iron loss via exfoliation of enterocytes and skin cells, and bleeding.44 Iron is absorbed in the gut, predominantly in the duodenum and upper jejunum, and transported into plasma by apical importer DMT1 and basolateral exporterferroportin, respectively [151].45
Iron in senescent RBCs that are ingested by macrophages and degraded is recycled back into plasma by ferroportin. Plasma iron is carried by glycoproteintransferrin that delivers iron to cells that express transferrin receptors.46 At blood pH, apotransferrin (transferrin iron-free form) can bind 2 Fe3 + (dissociation constant K d = 10–23 mol) in the presence of an anion (usually carbonate) that bridges iron and transferrin. Transferrin receptor-1 is expressed on all dividing cells.47 Erythrocyte precursors bind transferrin to incorporate iron into heme. Transferrin receptor-2 is expressed mainly in the liver and binds transferrin–iron complex with a much lower affinity than that of transferrin receptor-1.
Cellular iron is used or stored by ferritin.48 According to iron status, concentrations in iron transporters, carriers, and storage proteins, it is regulated transcriptionally by cytokines and post-transcriptionally owing to iron-regulatory proteins IRP1 and IRP2.
Ferritin is an intra- and extracellular protein. Plasma ferritin has a low iron content and distinct subunit composition. Ferritin binds high-molecular-weightkininogen to inhibit its cleavage bykallikrein, hence reducing production of bradykinin and cleaved high-molecular-weight kininogen. In addition, ferritin and high-molecular-weight kininogen colocalize in inflammation sites,49 where ferritin inhibits the cleavage of high-molecular-weight kininogen by tryptase and elastase. In addition, plasma light (L) and heavy (H) chain-enriched ferritin preferentially bind to plasmalemmal scavenger receptor ScaRa5 and T-cell immunoglobulin and mucin domain-containing protein TIMD2 that trigger endocytosis of the receptor–ferritin pair [152].
Small peptidic hormone hepcidin is a regulator of iron metabolism and systemic homeostasis to avoid pathological conditions of deficiency or overload. Hepcidin controls the surface expression of iron exporterferroportin (Fpn or SLC40a1) on the basolateral membrane of duodenal enterocytes and on iron-recycling macrophages that process senescent erythrocytes. Interaction of hepcidin with ferroportin precludes iron export from duodenal enterocytes and macrophages. Hepcidin is secreted by the liver50 to adapt iron absorption to the body’s need. Hepcidin transcription is controlled by iron and oxygen sensors, as well as inflammation. Hepcidin level increases with iron overload. Conversely, hepcidin expression is suppressed when iron is required for erythropoiesis. In addition, hepcidin is upregulated by inflammatory cytokines. Circulating hepcidin binds to ferroportin for degradation, and hence inhibits iron export into plasma.
Hepcidin expression depends onbone morphogenetic proteins (BMP2, BMP4, and BMP9 in vitro) and the SMAD4 pathway.51 On the hepatocyte surface, BMP6 forms a complex with BMP receptors (BMPR1a, BMPR1b, and BMPR2) and coreceptorhemojuvelin52 (Hjv) to activate SMADs. PhosphorylatedSMAD1, SMAD5, and SMAD8 complex with SMAD4 and translocates to the nucleus to activate HAMP gene promoter. Disruption of the Bmp6 gene in mice reduces hepcidin synthesis and rapidly causes a massive accumulation of iron in the liver,53 exocrine pancreas (acinar cells), heart, and renal convoluted tubules [153].
Hypoxia-inducible factors HIF1 and HIF2 bind to hepcidin HAMP gene promoter and block hepcidin expression. Inflammatory cytokines such as interleukin-6 activate signal transducer and activator of transcriptionSTAT3 that also binds to HAMP gene promoter and in the presence of SMAD4 induces hepcidin expression. Erythroid precursors secretegrowth differentiation factor GDF15. This cytokine, a member of the transforming growth factor-β family, may inhibit hepcidin gene transcription.
Known genetic defects of iron transport and metabolism involve proteins that act as: (1) iron transporters and exporters such as ferroportin; (2) receptors for iron-binding proteins, such as transferrin receptor and hemochromatosis; (3) regulators of iron transporters or iron-binding protein receptors such as hepcidin; and (4) and regulators of iron metabolism such as transcription factor SpiC that controls development of red pulp macrophages that recycle senescent RBC products.
3.6 Platelets
Platelets, or thrombocytes (TC),54 are small (size 2–4 μm) anucleate cellular fragments produced by megakaryocytes. The average lifetime is 10 days.55 The usual concentration is equal to 250–500 ×103/mm3. Platelets circulate in blood to ensure hemostasis and wound repair.
Platelets are generated from megakaryocytes in bone marrow. Megakaryocytes extend proplatelet-like protrusions into microvessels. These intravascular extensions are sheared and torn from their transendothelial stems by flowing blood [156]. Proplatelets are thus released in blood, at least partly due to applied hemodynamic forces.
Platelets are involved in bloodcoagulation in injured vessels and thrombosis in diseased conduits,56 as well as inflammation, immunity, and tumor progression. Platelet activation is also influenced by hemodynamic forces.
3.6.1 Platelet Cytoskeleton
Platelet cytoskeleton is involved in shape change, secretion, and clot contraction. Platelet shape rapidly changes on contact with foreign surfaces. Platelet actomyosin microfilaments (thrombostenin) organize in contractilestress fibers.
The cortex containsactin filaments and a circumferential bundle ofmicrotubules. An intracellular tubular system is open to the cell surface via invaginations of the plasma membrane. Dense tubules contain Ca2 + stores and enzymes involved in arachidonic acid metabolism that are required for platelet secretion and aggregation.
3.6.2 Translational Machinery
Platelets lack nuclear DNA but retain megakaryocyte-derived mRNAs and the translational machinery for protein biosynthesis, in particular transcription factors (e.g., peroxisome proliferator-activated receptors [nuclear receptors NR1c1–NR1c3], retinoid X receptors [NR2b1–NR2b2], glucocorticoid receptor [NR3c1], and nuclear factor-κB), spliceosome components to process mRNA, and the signal-dependent protein translation machinery. Post-transcriptional and translational mechanisms regulate platelet function.
Anucleate platelets released into the blood stream from bone marrow megakaryocytes contain not only mRNAs for protein synthesis, but alsomicroRNAs to regulate mRNA translation [157]. Platelets contain Dicer and Argonaute-2-containing ribonucleoproteic complexes to process microRNA precursors and control reporter transcripts.
3.6.3 Platelet Granules
The 2 major types of secretory granules include numerous α granules and large, dense granules (Table 3.8). Platelet dense granule membranes contain both granulophysin and P-selectin. α-Granule membrane containsgrowth factors,57 clotting factors,58 adhesion molecules,59 and chemotactic compounds.
Table 3.8
Platelet released substances (Sources: [159, 160]; 5HT: 5-hydroxytryptamine, or serotonin; HETE: hydroxyeicosatrienoic acid; PAF: platelet-activating factor; VWF: von Willebrand factor). Peptidase nexin-2, the secreted form of β-amyloid precursor protein, is an abundant platelet protein that, once secreted, is a potent inhibitor of activated coagulation factor XI. (Peptidase nexin-1, or serpin-E2, is a potent inhibitor of the serine peptidase thrombin).
Location | Substance | Effect |
---|---|---|
α granule | IGF, PDGF, TGFβ | Chemotaxis, ECM deposition |
CXCL4, β-thromboglobulin | GF activation | |
Fibronectin, fibrinogen, | TC aggregation | |
thrombospondin | ||
Albumin | ||
Nexin-2 | Peptidase inhibition | |
Tetraspanin-30 | ||
Dense granule | ADP, ATP, Ca2 + | TC aggregation |
(δ granule) | 5HT, catecholamines | Vasoconstriction |
Cytosol | vWF | TC adhesion |
Fibronectin | Coagulation | |
PAF | TC activation | |
TxA2, 12HETE | Vasoconstriction |
Granules fuse by exocytosis with the plasma membrane or membrane of the tubular system for content release. Vessel wall collagen and various molecules, such asplatelet-activating factor,serotonin,60 and products ofarachidonic acid metabolism, secreted by platelets and other cells, activate platelets. Activated platelets become spherical (contraction of actin filaments and microtubules), form pseudopodia for adhesion, secrete substances, and aggregate.
Activation of glycoprotein-6, a platelet-specific receptor for collagen, causes platelet-derived vesicle release and shedding in response to platelet activation [161]. These pro-inflammatory microparticles can transport molecules throughout the body, possibly in association with transmigrating leukocytes during inflammation, as they adhere to activated polymorphonuclear leukocytes and monocytes in blood circulation.
3.6.4 Platelet Activation
Circulating platelets are kept in an inactive state particularly byprostacyclin (PGi2) andnitric oxide released by endothelial cells (Sect. 9.8). Endothelial cells also express plasmalemmalectonucleotide pyrophosphatase–phosphodiesterase ENPP1 that inhibits platelet activation byadenosine diphosphate, as it converts ADP into AMP.
Platelet synthesizes and releases multiple molecules (caspases, osteonectin, ubiquitin, vimentin, tissue inhibitors of matrix metallopeptidases, etc.). Activated platelets promote blood coagulation on their surface, produce mediators of inflammation, and cause smooth myocyte proliferation.
In resting platelets, cholesterol is uniformly distributed on the cell surface and confined to multivesicular bodies, dense granules, and internal membranes of alpha granules [162]. Upon interaction with fibrinogen, cholesterol accumulates at the leading edge during platelet spreading on fibrinogen, especially at tips of filopodia. Upon stimulation with thrombin receptor-activating peptides (TRAP), which are synthetic parts of the liberated N-terminal tail of the cleaved, moderate-affinity, thrombin receptor, cholesterol also redistributes to filopodia. This process results from the concentration ofSrc kinase andtetraspanin-30 in these membrane nanodomains (rafts).
Thrombin, or blood coagulation factor II, is a component of the clotting cascade and strong activator of platelet aggregation. It targetspeptidase-activated receptors (PAR1–PAR4). The serine peptidasethrombin initiates various platelet responses (shape change, pseudopod extension, eicosanoid production, granule release, adhesive receptor expression, and aggregation) viaphospholipase-C, intracellular calcium flux, and activation of kinases. Another serine peptidase,trypsin, also activates platelets, stimulating their aggregation and phosphoinositide metabolism. Both thrombin and trypsin cleave the terminal region of the moderate-affinity thrombin receptor, thereby allowing the released C-terminal fragment to subsequently stimulate the processed receptor. In addition, thrombin acts by a non-proteolytic mechanism, possibly via a high-affinity receptor such as glycoprotein-1B, which is not cleaved by thrombin.
Arachidonic acid is enriched in platelet phospholipids, such as phosphatidylcholine, phosphatidylethanolamine, phosphatidylinositol, and phosphatidylserine. Upon platelet activation, arachidonic acid is released from membrane phospholipids, most likely bycytosolic phospholipase-A2, and converted by the cyclooxygenase axis tothromboxane-A2 that causes full platelet activation (positivefeedback loop) [163].
At injury sites, platelets adhere to the exposed subendothelium and interact with collagen, von Willebrand factor, and fibronectin via their receptors, integrins, and glycoproteic GP1b–GP9 complex. Glycoproteins GP1b (GP1bα–GP1bβdimer), GP5, and GP9 that are members of the leucine-rich glycoprotein (LRG) family complex at the platelet surface. Glycoprotein GP1bα (encoded by the GP1BA gene) binds tovon Willebrand factor and thrombin on the one hand, and platelet cytoskeleton via actin-binding proteins on the other [164].61 Binding of the GPIb–GP9 heterodimer to von Willebrand factor exposed in the subendothelium enables platelet adhesion at sites of injury. In addition, platelet GP5 has a high-affinity binding for thrombin.
Boththrombin andADP activate platelets, which then secrete ADP,platelet-derived growth factor, andfibrinogen from storage granules, as well as thromboxane-A2 by immediate synthesis.
Platelet α2Bβ3-integrin62 binds to fibrinogen, leading to platelet aggregation. With the simultaneous formation of a fibrin mesh, this process lead to the formation of a platelet thrombus.
3.6.5 Non-Genomic Function of Transcription Factors
Transcription factors in platelets can have non-genomic functions. Platelets possess ligand-regulated transcription factorsperoxisome proliferator-activated receptor-β and -γ (or nuclear receptors NR1c2 and NR1c3) and their binding partnerretinoid X receptor-α and -β (or nuclear receptors NR2b1 and NR2b2), as well as glucocorticoid receptor (or nuclear receptor NR3c1) [165]. The RXR ligands prevent platelet aggregation andthromboxane-A2 release on Gq activation triggered by ADP and TxA2 receptors. Nuclear receptors of the RXR category binds to Gq subunit of G protein, thereby hindering Gq-induced Rac activation and calcium release.
Factor PPARγ reduce platelet release of mediators such as tumor-necrosis factor superfamily member TNFSF5, in addition to thromboxane-A2 [166]. Moreover, collagen-stimulated platelet aggregation is attenuated by PPARγ via inhibition of the collagen receptor GP6 [167]. Ligands of PPARγ precludes phosphorylation of components of the GP6 signaling pathway. Factor PPARγ links toSYK kinase that phosphoryles Linker for activation of T cells after platelet activation. This association is impeded by PPARγ agonists.
Nuclear factor-κ light chain-enhancer of activated B cells (NFκB) exerts anegative feedback loop that limits platelet activation [168]. Factor NFκB builds a complex withprotein kinase-A that contains inhibitor of NFκB IκBα. The latter is phosphorylated byinhibitor of NFκB kinase. This event releases PKA that can then phosphorylate the actin-dynamicsinhibitor vasodilator-stimulated phosphoprotein. The NFκB–PKA pathway is stimulated by thrombin and collagen. It limits platelet activation and motility, hence thrombus stability, as it reduces cytoskeletal dynamics and early-stage aggregation.
Diverse elements of the NFκB family in platelets produce different associations of NFκB subunits that initiate different signalings according to the source of platelet stimulation. Fibrinogen-activated platelets cause platelet spreading via NFκB that influences fibrinogen binding to α2Bβ3-integrin [169].
Thrombin-activated platelets, but not resting cells, synthesize B-cell lymphoma-3 protein [170]. Protein BCL3 is a member of the atypical IκB subfamily that acts not only as a transcriptional coregulator, but also as a component of the autoregulatory loop via its association with NFκB. In activated platelets, BCL3 interacts withFyn kinase to produce a retracted fibrin clot, the clot consisting of aggregated platelets and a mesh of fibrins.
Furthermore,inhibitor of NFκB kinases have other substrates than inhibitors of NFκB, such asAMPK andMAP3K7 kinases [171]. In platelets, AMPK and MAP3K7 contribute to signaling for adhesion and aggregation of platelets as well as exocytosis of mediators.
3.6.6 Other Regulators of the Platelet Function
At least, 3 subtypes ofP2 receptors are implicated in platelet activation: P2X1, P2Y1, and P2Y12 (Vol. 3 – Chap. 2. Membrane Ion Carriers and 7. G-Protein-Coupled Receptors). Ion channel P2X1 activated by ATP during collagen-induced platelet activation generates a rapid influx of calcium, which leads to a change in platelet shape and platelet aggregation. Gq-coupled P2Y1 receptor also modifies platelet shape and provokes aggregation via calcium influx. Adenosine diphosphate binds to the P2Y1 receptor. Gi-coupled P2Y12 receptor that binds ADP inhibitsadenylate cyclase. The activation ofphosphatidylinositol 3-kinase by ADP G-protein-coupled receptors triggers platelet aggregation.
The Wnt–β-catenin signaling pathway functions in human platelets [172]. Platelets possess Wnt receptors such as Frizzled-4 and -6 receptors as well as LRP5 and LRP6 coreceptors and Disheveled-2 mediator. Wnt3a ligand prevents platelet activation, dense granule secretion, adhesion, and aggregation [172]. In addition, Wnt3a hampers the thrombin-induced activation ofRhoA GTPase (non-canonical Wnt signaling). Among non-genomic functions, β-catenins link adhesion receptors of the cadherin family to the actin cytoskeleton.
3.6.7 Inflammatory Platelet
Platelets can also be considered as inflammatory cells that synthesize proteins involved in inflammatory pathways (Chap. 11). Granules α of platelets indeed contain inflammatory modulators, such as P-selectin,63 tumor-necrosis factor superfamily member TNFSF5,64thrombospondin-1, CXCL4 chemokine,65transforming growth factor-β, and interleukin-1 precursors.
3.7 Granulocytes
Granulocytes are innate immunocytes that contain granules involved in immune defense. They are also called polymorphonuclear leukocytes, as their nuclei are usually lobed (2–4 lobes). The names of the 3 types of granulocytes are derived from neutrally, eosinophilic, and basophilic staining of cytoplasmic granules.
3.7.1 Basophils
Basophils (B
; size 12–15 μm; 20–80 μdm3) have numerous large basophilic granules. They represent less than 1% of all leukocytes. Basophils are the least abundant granulocytes. These short-lived cells have an expected half-life of a few days. Basophils express immunoglobulin-E receptors (FcεR1).

Circulating basophils originate from CD34 + , FcεR1αhigh,66 SCFR − hematopoietic progenitors in the bone marrow that express transcription factorsCCAAT–enhancer-binding protein-α andGATA2 [173]. Mature basophils exit the bone marrow.
Activated T lymphocytes produce interleukin-3 and other factors to enhance basophil production in the bone marrow. Because FcεR1 expression can change during immune response, PTPRc (or CD45) is a useful marker of basophils.67
Basophils are similar to mastocytes, but distinct. In normal conditions, they are not detected in the blood circulation.68 Basophils differ from mastocytes and eosinophils, as they do not express plasmalemmalstem cell factor receptor (SCFR)69 and chemokine receptor CCR3, respectively. Human basophils also express TLR2 and TLR4 Toll-like receptors.
The behavior of basophils in vivo can differ from that in vitro. Moreover, functioning differences exist between mammal species. For example, mouse basophils express peptidase-activated receptors, but human basophils do not. Basophils can be activated by many agents, such as cytokines, immunoglobulins, peptidases, TLR2 ligands, and parasite-associated antigens.
Committed basophils release 2 kinds of mediators: (1) preformed granule-associated mediators, such as vasodilator histamine, serotonin, bradykinin, anticoagulant heparin, and cytokines; and (2) newly generated mediators, such as prostaglandins and leukotrienes. Both transmembraneectonucleotide pyrophosph- atase-phosphodiesterase ENPP3 (or CD203c) andTspan30 (or CD63) are markers of activated human basophils.
Basophils release numerous potent inflammatory mediators from their cytoplasmic granules upon high temperature, mechanical stress, ionization, toxins, peptidases, cationic proteins derived from eosinophils and neutrophils, IgE-mediated processes as well as IgE-independent immune mechanisms, such as that primed by complement factors.
Low-molecular-weight mediators include histamine, serotonin, prostaglandins, leukotrienes. These mediators are released in response of allergens. They cause increased vascular permeability, smooth muscle constraction, vasodilation.
Basophils are the main source of interleukin-4 in allergen- and helminth-activated blood mononuclear cells [174]. Basophils highly express IL33 receptor (IL33R or IL1RL1) and produce IL4 and IL13 in response to IL33, a member of the IL1 family, usingMyD88 adaptor [173]. Interleukin-33 provokes airway hyper-responsiveness and goblet cell hyperplasia. Activated basophils not only secrete cytokines (Tables 3.9 and 3.10) to support the development of IL4-producing CD4 + T lymphocytes and IgE-secreting B lymphocytes associated with type-2 helper T immunity, but also produce chemoattractants for eosinophils and neutrophils into inflammatory sites.70 Ligands of TLR2 induce nuclear translocation of transcription factorNFκB and cytokine secretion. On the other hand, TLR4 ligand lipopolysaccharides remain inactive, as CD14 is not expressed by basophils [173].
Table 3.9
Leukocyte sources of cytokines, especially type-1 and -2 immunocytokines (Part 1; Sources: [175, 928]). Cytokines can be classified into type-1 cytokines (IL2, IL12, IL18, Ifnγ, TGFβ, TNFα and -β [or TNFSF2]) that promote cellular immune response and type-2 cytokines (IL4, IL5, IL6, IL9, IL10, IL13, and IL25, as well as granulocyte–macrophage colony-stimulating factor [gmCSF or CSF2]) that favor humoral (antibody-mediated) immune response. Type-2 response is defined as an attenuated cellular response, but with a strong B-cell activity such as hypergammaglobulinemia and an increase in the level of type-2 cytokines. Type-1 and -2 cytokines then refer to cytokines produced by CD8 + and CD4 + type-1 effector T cells, such as type-1 cytotoxic (TC1) and helper T (TH1) cells, respectively, and type-2 effector T lymphocytes (TC2 and TH2, respectively), respectively. Some of these type-1 and -2 cytokines are cross-regulatory: interferon-γ and interleukin-12 decrease the concentrations of type-2 cytokines, whereas IL4 and IL10 reduce the levels of type-1 cytokines. Type-1 cytokines, such as interferon-γ produced in particular by CD8 + and CD4 + type-1 effector T cells, interleukin-12 by antigen-presenting cells, and IL18 by activated macrophages, are required for effective responses to intracellular pathogens such as viruses. Type-2 cytokines produced by CD8 + and CD4 + type-2 effector T cells, mediate allergic responses. On the other hand, class-1 cytokines comprise IL1αand 1β, IL2–IL7, IL9, IL11, IL12a–IL12b, IL13, IL15, IL18, IL21, IL23, and IL33, CSF2, granulocyte colony-stimulating factor (gCSF or CSF3), B-cell stimulating factor-3, leptin, erythropoietin, growth hormone, and prolactin.
Cell | Cytokines | |
---|---|---|
Type 1 | Type 2 | |
B cell | IL12/18, TNFβ | IL6/10 |
NK cell | Ifnγ, TNFβ | |
CD4 + T cell | IL2/12, Ifnγ, TNFβ | IL4/5/6/10/13 |
CD8 + T cell | IL2, Ifnγ | IL4/5/10 |
Table 3.10
Leukocyte sources of cytokines, especially type-1 and -2 immunocytokines (Part 2; Sources: [175, 928]; CSF: colony-stimulating factor; gCSFR corresponds to CSF3R and CD114, gmCSFR to CSF2R and CD116; mCSFR to CSF1R and CD115; GH: growth hormone; GHR: growth hormone receptor; Ifn: interferon; IL: interleukin).
Cell | Cytokines | |
---|---|---|
Type 1 | Type 2 | |
Basophil | IL4/5/6/13 | |
IL1β/3/8 | ||
Receptors of IL1–IL6/8/13/16/17/29/31/33 | ||
CSF2, CSF2R | ||
Receptors of Ifnα/β | ||
Dendritic cell | IL12 | |
Eosinophil | IL2/12, Ifnγ | IL4/5/6/9/13/25 |
IL1α/3/8/10–11/16 | ||
Receptors of IL1–6/8–10/12–13/15–16/18/29/31/33 | ||
gmCSF, CSF2R | ||
Receptors of Ifnα/γ | ||
Mastocyte | IL4/5/6 | |
Macrophage | IL12 | IL6/10 |
Monocyte | IL12 | IL6/10 |
Neutrophil | IL12 | IL4/6/9 |
IL1/8/15/16/18/20/32 | ||
Receptors of IL1–10/12–13/15–18/29/31 | ||
GH, GHR, CSF2R, CSF3R | ||
Ifnα/β | ||
Receptors of Ifnα/γ/ω |
Basophils represent the main antigen-binding cell population in the bone marrow, spleen, and blood after protein immunization. Their capacity to bind large amounts of antigens on their surface depends on their expression of immunoglobulin-E receptors and antigen-specific IgE produced by B cells after immunization [174]. Activated basophils express plasmalemmal receptors such as CD40L. Activated basophils also enhance humoral memory B-cell responses by producing interleukin-4 and -6 in response to specific antigens.
Basophils induce a TH2 phenotype in CD4 + T lymphocytes, especially during parasite infection and allergic inflammation. Basophils activate the production by CD4 + T cell of interleukins IL4, IL5, IL10, IL13, and transcription factor GATA3, but downregulate interferon-γ and IL2. Basophils synthesize both IL4 and thymic stromal lymphopoietin to elicit TH2 immunity. In addition, TH2 differentiation promoted by basophils involves intercellular contact with CD4 + T lymphocytes in draining lymph nodes [173].
Human basophils, after IgE crosslinkage, secrete IL25 (or IL17e). IL25 stimulates the production of TH2 cytokines IL4, IL5, and IL13 and recruitment of eosinophils. IL25 can also activate TH2 memory T cells that strongly express IL25 receptor.
Basophils are rarely detected in normal tissues, but reside in sites of allergic inflammation, especially in respiratory ducts of asthmatic patients. Basophil products, such as interleukin-4 and -13, leukotriene-C4, and histamine, cause symptoms of acute and chronicallergy.
3.7.2 Eosinophils
Eosinophil (E
; size ∼ 15 μm) has large granules and a nucleus which often has two lobes. The granules contain digestive enzymes that are effective against parasitic larvae. These cells also phagocytize antigen–antibody complexes. These cells account for less than 5% of all leukocytes.

In addition to the release of toxic granule content and interleukin-4 (that drives lymphocytes toward a type-2 helper T phenotype and activation of Toll-like receptor-2 on dendritic cells, eosinophils defend the body against pathogens by rapidly ejecting mitochondrial DNA [177]. Expelled mitochondrial DNAs act as an extracellular immune effector, especially in the gastrointestinal tract, that generate a sticky matrix to trap bacteria and activate their death by cationic antimicrobial peptides such as cathelicidins and nuclear proteins such as histones. Ejection of mitochondrial DNAs depends on reactive oxygen species and interleukin-5 produced by TH2 cells.
3.7.3 Neutrophils
Neutrophil (N
; size 8–15 μm) has small plasma granules and frequently multilobed nucleus. Normally, neutrophils account for 50 to 70% of all leukocytes. Scouting neutrophils look after possible invading agents.

Neutrophils possess an arsenal of hydrolytic and oxidative substances as well as pore-forming molecules such as pannexins that can cause tissue destruction that can contribute to further ischemic injury as well as autoimmunity.
3.7.3.1 Immunostimulatory and Immunosuppressive Neutrophils
Tumors avoid the activity of the immune system, as they set up an immunosuppressive environment. Neutrophils that are major effector cells during inflammation can also control excessive inflammation, as they secrete anti-inflammatory cytokines.
The neutrophil phenotype is regulated by the acute-phase response proteinserum amyloid-A1. Increased concentration of serum amyloid-A1 observed in tumors as well as blood renders neutrophils immunosuppressive [178]. Serum amyloid-A1 indeed provokes differentiation of neutrophils that release anti-inflammatory interleukin-10. However, it also fosters the interaction ofinvariant natural killer T cells with immunosuppressive neutrophils to limit their suppressive activity, as the production of IL10 and IL12 is reduced and enhanced, respectively. Therefore, neutrophils adopt a pro-inflammatory phenotype. Invariant NKT cells thus counteract immunosuppression and oppose cancer development [178].
3.7.3.2 Neutrophils as Scavengers in Cell Necrosis
Neutrophils are rapidly recruited to the site of inflammation, hence responding not only to microbial infection, but also sterile injury and organ necrosis to contribute to wound healing. Cell necrosis releases multiple pro-inflammatory damage-associated molecular patterns (DAMP), or host-derived indicators of cellular damage, such as proteins, nucleic acids, extracellular matrix components, and lipid mediators. These DAMPs recruit neutrophils [179].
Adenosine triphosphate generated from necrotic cells at the injury site activates the NLRP371inflammasome to assist neutrophils in extravasation across the vascular endothelium using integrins [179] (Vol. 3 – Chap. 11. Receptors of the Immune System).72The NLRP3 inflammasome promotes maturation and secretion of interleukin-1β. It can integrate signals from interleukin-1β, reactive oxygen species, and thioredoxin-interacting protein, thereby acting as a sensor for metabolic stress, such as in insulin resistance and type-2diabetes mellitus [180]. Maturation of IL1β into an active form is actually controlled by caspase-1-activating platforms, the so-called inflammasomes. In addition, formyl peptides released from necrotic cells guide neutrophils to the injury site [179].
3.7.3.3 Neutrophils as Microphages for Pathogens
Neutrophils constitute a first line of defense against invading pathogens. These polymorphonuclear microphages are able to phagocytize foreign cells, toxins, and viruses. Neutrophils use neutrophil extracellular traps that consist of chromatin coated with peptidases, such as myeloperoxidase, elastase, and cathepsin-G, to trap and kill bacteria [97].
Neutrophils express numerous receptors for bacterial phagocytosis, such as αMβ2-integrin that binds complement component C3b on the surface of opsonized bacteria and IgG Fc receptors (FcγR) that bind IgG-coated particles and immune complexes [97]. These molecules facilitate the uptake of opsonized bacteria into phagosomes. Phagosomes then fuse with lysosomes and neutrophil granules that contain numerous proteolytic and antibacterial enzymes and peptides. NADPH oxidase of phagolysosome membranes generatesreactive oxygen species to destroy bacteria.
Human neutrophil peptides are released from granules of activated leukocytes to kill pathogens. Human neutrophil peptides can also modulate inflammation and vascular tone. In endothelial cells stimulated by human neutrophil peptides,COx2 expression (but not COx1) increases in time- and dose-dependent manner [181]. However, human neutrophil peptides do not significantly enhance COx2 activity, hence PGI2 production, but provoke the release ofendothelin-1.
3.7.3.4 Neutrophil Migration
Neutrophils are the first recruited cells and responders to sites of inflammation and infection by primary chemoattractants. They then attract other cells, as they release secondary (relay) chemoattractants such asleukotriene-B4 that enables neutrophil activation and migration [182].73
Neutrophil migration relies on 2 main pathways: (1) phosphatidylinositol 3-kinase that is activated by interactions between chemokines and G-protein-coupled receptors and (2) mitogen-activated protein kinase P38MAPK pathway that dominates in chemotaxis induced by bacterial or complement chemoattractants.Lipid and protein phosphatase and tensin homolog (PTen) that counteracts PI3K acts for priorization of bacterial chemoattractants for neutrophil migration toward bacterial products viaphospholipase-A2 and P38MAPK [183].
In their search for bacteria, neutrophils respond to many chemotactic substances produced by macrophages, mastocytes, or serosal cells. Near the infection site, neutrophils select chemoattractants, favoring bacterial chemoattractants (e.g., Nformyl-methionyl-leucyl-phenalanine andcomplement component C5a) rather than chemokines and leukotrienes.
3.7.3.5 Neutrophil Extravasation
Pentraxin-3 (a.k.a. TNFα-induced protein TNFαIP5) has both inflammatory and anti-inflammatory effects.74 On the one hand, cytokines and liganded Toll-like receptors stimulate Ptx3 synthesis in endothelial cells, macrophages, and dendritic cells, as well as Ptx3 storage in neutrophils [184]. Neutrophils secrete Ptx3 that promotes innate immunity, as it binds to pathogens and activatescomplement [185]. C-terminus of Ptx3 links to the complement protein C1q, among other substances such as P-selectin.
Inflammation relies on the recruitment of circulating cells. Rolling permits selectin ligands and chemokine receptors on neutrophils to prime deceleration, arrest, then extravasation. Pentraxin-3 can limit neutrophil recruitment, as it impedes rolling on P-selectin in inflamed venules. It indeed sequesters P-selectin away from its endothelial ligands. In summary, neutrophils rolling on P-selectin in venules at sites of infection or injury receive signals that release paracrine regulator Ptx3 from specific granules. Released Ptx3 binds to locally expressed P-selectin in a paracrine manner.
Therefore, pentraxin-3 is another inhibitor of leukocyte adhesion to vascular endothelia. The integrin ligand EGF-like repeats and discoidin-1 (I)-like domain-containing protein EDIL3 is a matrix glycoprotein secreted by endothelial cells.75 EDIL3 ligand inhibits adhesion of leukocytes and endothelial progenitor cells, as it competes for integrin binding with ICAM1, a major β2-integrin ligand [186, 187].76 Consequently, different proteins preclude inflammation, as they impede either selectin- or integrin-dependent leukocyte adhesion.
3.7.3.6 Neutrophils in Lungs
Alveolar type-2 cells77 releasegranulocyte colony-stimulating factor (CSF3),78 interleukin-8, andleukotriene-B4 to attract neutrophils [188]. These chemoattractants are synthesized not only in alveolar and airway epithelial cells, but also in endothelial cells and fibroblasts. Interleukin-1β, tumor-necrosis factor-α, bacterial lipopolysaccharide, andbradykinin stimulate the release of these chemoattractants, particularly CSF3.
In addition,alveolar type-2 epithelial cells secreteinterleukin-6,chemokines CCL279 and CCL5,80granulocyte–macrophage colony-stimulating factor (CSF2),transforming growth factor-β, constitutively and in response toTNFα and IL1β [188].
In humans, major chemoattractants for neutrophils during lung inflammation are Glu-Leu-Arg (or ELR) motif-containing (ELR + ) CXC chemokines that include CXCL1, CXCL2 and CXCL3, and CXCL8 (IL8). The receptors for these chemoattractants are CXCR1 and CXCR2 in humans.Matrix constituent-derived peptides can also behave as chemoattractants. Certaincollagen fragments can provoke accumulation of pulmonary neutrophils. The tripeptide Nacetyl Pro–Gly–Pro (or PGP) that arises from the breakdown of matrix constituents after exposure to lipopolysaccharides in mouse lungs and can be detected in patients with chronic obstructive pulmonary disease fosters chemotaxis of neutrophils and production of superoxide via CXCR1 and CXCR2 receptors [189].81
3.8 Mastocytes
Mastocytes, or mast cells,82 are leukocytes that contain storage granules for inflammatory mediators, such as histamine, serotonin, proteolytic enzymes cleaving complement components, heparin, chondroitin sulfate, and chemotactic factors, in particular for eosinophils and neutrophils. Mastocytes contribute to innate and adaptive immunity, angiogenesis, wound healing, tissue remodeling, and immunoregulation [190].
Mastocytes are tissue-resident sentinels. Differences in concentrations of stem cell factor and other cytokines and chemokines (interleukins IL3, IL2, IL4, IL9, and IL18 as well as chemokines CCL2 and CCL5), or nerve growth factor in respective tissues, and possibly between different regions within the same tissue, can determine the number, distribution, and subtype of mastocytes at these loci. Mastocytes recruit many immunocyte types (granulocytes, monocytes, macrophages, dendritic cells, B-, T-, and NK cells; Table 3.11).
Table 3.11
Immunomodulatory mastocytes (Source: [191]; IL: interleukin; PG: prostaglandin: TNF: tumor-necrosis factor; TSLP: thymic stromal lymphopoietin). Mastocytes initiate and increase magnitude and/or duration of immune response (positive activity). They promote the initiation and augment the magnitude of inflammation and tissue remodeling, as well as tissue reaction during allergic and autoimmune disorders. Mastocytes also have anti-inflammatory and immunosuppressive (negative) activity. Mastocytes can first enhance and later limit certain innate and adaptive immune responses.
Positive functions | Negative effects |
---|---|
Maturation, differentiation, and | Suppression of cytokine production |
recruitment of immunocytes | by T cells, monocytes, and |
(via chemokines, histamine, | keratinocytes |
leukotriene-B4, TNF, and peptidases) | (via IL10) |
Antigen presentation to T cells | Enhancement of dendritic |
Production of IgE by B cells | cell-mediated decrease of |
(via IL4, IL13, and TNFSF5) | T-cell proliferation and cytokine |
production (via IL10) | |
Helper-2 T-cell responses (via PGd2 | |
on maturation of dendritic cells) | |
Expression of TSLP by epithelial cells | |
(via TNF, IL4, and IL13) | |
Production of chemo- and cytokines by | |
airway smooth myocytes | |
(via TNF, IL4 and IL13) |
3.8.1 Mastocyte Origin and Maturation
Mastocytes derive from hematopoietic stem cells and undergo differentiation and maturation after migration of mastocyte precursors from blood to tissues, especially those exposed to the environment (skin, conjunctiva membrane of eyes, airways, and gastrointestinal and genital tract), or serosal cavities (pericardial, peritoneal, and pleural cavities).83
Mastocytes derive from multipotent hematopoietic progenitor cells in the bone marrow and Thy1low,84 SCFRhigh,85 CD13 + ,86 CD33 + ,87 CD34 + ,88 CD38 + ,89 bone marrow-derived circulating progenitors and FcεRI − precursors in blood. These precursors generate mastocytes only in destination tissue (in which they become resident).
Stem cell factor is required to ensure mastocyte survival in the body’s tissues.90 However, the mature mastocyte type (mucosal and connective tissue mastocytes, among other types) varies according to growth factor stimuli (interleukins IL3, IL4, and IL9; transforming growth factor-β1; etc.) [191].
Mastocytes are long-lived cells that proliferate upon suitable stimulation. Mastocyte recruitment and local maturation of mastocyte progenitors contribute to the expansion of mastocyte population. Division and tissue redistribution of mastocytes, as well as mastocyte type modulation particularly occur during helper-2 T-cell responses.
Mastocytes can also derive from thymocytes at double-negative stages DN1 and DN2 (but not at DN3 stage) when transcription factorGATA3 is upregulated, although Gata3 is one of the earliest activated genes when theNotch pathway triggers T-lineage differentiation from hematopoietic precursors [192]. DN2 thymocytes can also bear respecification to the mastocyte lineage in the presence of interleukin-3 and stem cell factor and in the simulataneous absence of Notch signaling.
3.8.2 Mastocyte Functions
Mastocytes contribute to both allergy initiation and early phase by releasing pro-inflammatory mediators (histamine, tryptase, prostaglandins, and leukotrienes) and late phase by liberating cytokines and chemokines that recruit inflammatory cells (eosinophils, basophils, neutrophils, CD4 + T cells, and macrophages).
Mastocytes also favors angiogenesis, as they produce growth factors, such as vascular endothelial growth factor, fibroblast growth factor-2, platelet-derived growth factor, transforming growth factor-β, tumor-necrosis factor-α, granulocyte–macrophage colony-stimulating factor, and interleukin-3 and -8.
Mastocytes possess features of acquired immunity cells: they phagocytize, and process and present antigens to T lymphocytes and modulate T- and B-cell responses such as lymphocyte growth, recruitment, and production of immunoglobulins (Table 3.12). Mastocytes express major histocompatibility complex class-1 molecules, and are able to produce MHC class-2 molecules under some circumstances. Mastocytes express receptor FcεR1 for immunoglobulin-E produced by B cells. Mastocytes operate in acquired immunity against parasites and other pathogens, such as bacteria and viruses in the gut and skin. Certain mastocytes are activated by IgG via Fcγ receptors (Table 3.13).
Table 3.12
Mastocyte functions.
Activity | Targets |
---|---|
Procedure | |
Recruitment and activation of | Monocytes and macrophages |
Release of lipid mediators – icosanoids | |
(prostaglandins, prostacyclins, thromboxanes, | |
and leukotrienes) | |
Dendritic cells | |
T lymphocytes | |
Basophils, eosinophils, and neutrophils | |
Phagocytosis | |
Alterations of | Epithelial tight junctions |
Degradation of | Toxins (by chymase and/or other peptidases) |
Venoms (by carboxypeptidase-A) | |
Bactericidal activity | Release cathelicidins and chymases |
Defense against | Some parasites |
(innate and IgE-mediated acquired responses) | |
Viruses (via CD8 + T cells) | |
Fungi |
Molecule | Receptor |
---|---|
Lipopolysaccharide | Toll-like recptor TLR4 |
Peptidoglycan | Toll-like recptor TLR2 |
Complement | CR1, CR2, CR3, C3aR, C5aR |
Endothelin-1 | ETA |
IgE | Fc ![]() |
IgG1 | FcγR3 |
Parasites | Fc ![]() |
Bacteria and their products | CD48; Toll-like recptor TLR2, -4, -6, and -9 |
Double-stranded viral RNA | Toll-like recptor TLR3 |
Single-stranded viral RNA | Toll-like recptor TLR7 |
Venoms | G proteins |
3.8.3 Mastocyte Production and Types
Mastocytes produce many mediators that positively or negatively regulate the fate and function of immunocytes [191]. Mastocytes promote recruitment of immunocytes via secretion of tumor-necrosis factor and histamine.
Histamine elicits helper-1 T-cell activation viaH1 receptor, but suppresses bothTH1– and TH2-cell activation via H2 receptor.
Mastocytes release cytokines that influence polarization and function of T-cell subsets.Interleukins IL4, IL5, IL6, and IL13 andTNFSF5 can affect B-cell development and function (e.g., IgE production). Mastocyte-derived IL10 increases the ability of dendritic cells to prevent T-cell proliferation and cytokine production and directly inhibits the production of prostanoids by neutrophils and inflammatory cytokines by macrophages.
Long-lived mastocytes experience multiple cycles of de- and regranulation. Two types of mastocytes include T and CT phenotypes according to mastocyte content: eithertryptase alone (Tmastocyte) orchymase and tryptase (CTmastocyte), respectively. Both subtypes coexist. Multiple molecules are released into the extracellular medium (Tables 3.14 to 3.16).
Table 3.14
Molecules of mastocytes (Source: [194]). (Part 1) Granule-stored preformed mediators and de novo synthesized mediators. Mastocytes interact with blood vessels and nerves. The set of expressed cytokines varies according to the maturity state of mastocyte and tissue of residence. Histamine, eicosanoids, and other effectors are released upon crosslinking of surface-bound immunoglobulin-E by allergens or other mediators, such as TH2-cell cytokines (IL3, IL5, and IL13), fibroblast growth factor-2, transforming growth factor-β, and proteoglycans. Other stimuli that provokes the release of mastocyte mediators comprise C3a to C5a, UV light, substance-P, bacterial products, α-melanocyte-stimulating hormone released by keratinocytes, IL8, etc. Stem cell factor in the skin, produced by keratinocytes, endothelial cells, fibroblasts, melanocytes, and mastocytes, stimulates melanocytes and mastocytes (autocrine regulation) via SCFR receptor. Lipid mediators (cyclooxygenase [prostaglandins] and lipoxygenase [leukotrienes] metabolites of arachidonic acid) improve innate responses by recruiting pro-inflammatory cells. Mastocytes are sources of coagulation-regulating factors. Mastocytes store several peptidases in their secretory granules (tryptase, chymase, carboxypeptidase-A, andcathepsin-G). Chymase can activate collagenase and stromelysin, destruct vitronectin and fibronectin, and elicit fibroblast proliferation.
Agent | Effects |
---|---|
Granule-stored preformed mediators | |
Histamine | Allergy, inflammation |
Vasodilation | |
Increase in blood flow and vessel wall permeability | |
Upregulation of endothelium adhesion molecules | |
Upregulation of keratinocyte expression of integrins | |
Fibroblast proliferation | |
Serotonin | Vasoconstriction during injury |
Endothelial nitric oxide synthase activation | |
Proteoglycans | Clotting control |
(mainly heparin) | |
Peptidases | Proteolysis |
Activation of matrix metallopeptidases | |
(MMP2 and MMP9 by chymase) | |
De novo synthesized mediators | |
Prostaglandin-D2 | Nervous regulation |
Leukotriene-B4 | T-cell recruitment |
Leukotriene-C4 | Allergen-mediated release (no bacterial priming) |
Leukotriene-B4/C4/D4 | Keratinocyte growth |
Platelet-activating factor | Moncyte/macrophage recruitment and activation |
Table 3.16
Molecules of mastocytes (Source: [194]). (Part 3) Cytokines and chemokines (MCP: monocyte chemoattractant protein, MCP1 to MCP3 being CCL2, CCL8, and CCL7 chemokines, respectively; MIP: macrophage inflammatory protein, MIP1α to MIP1δ being CCL3, CCL4, CCL9, and CCL15, respectively).
TNFα | Expression of endothelial leukocyte adhesion molecule-1 |
Promotion of IL8 synthesis | |
Neutrophil recruitment | |
T-cell recruitment | |
Lymph node hypertrophy (adaptive immune response) | |
Langerhans cell migration | |
Interleukin-1, IL3–IL6, IL8, IL10, IL11, IL13, and IL16 | |
IL3 | Keratinocyte growth and differentiation |
IL4 | Langerhans cell migration and maturation |
IL8 | Granulocyte recruitment and activation |
Melanocyte growth | |
IL10 | Tolerance induction |
Chemokines | |
CCL2 (MCP1) | Chemotaxis |
CCL3 (MIPα) | Chemotaxis |
Miscellaneous | |
Neuropeptides | Communication with the nervous system |
Endothelin-1 | Vasoconstriction |
3.8.3.1 Mastocytes of the Genital Tract
In the genital tract, mastocytes are activated by estrogens and lutenizing hormone, as well as corticotropin-releasing hormone, endothelin-1, and substance-P [195]. Mastocytes are the main source ofinducible nitric oxide synthase in the uterus.
Table 3.15
Molecules of mastocytes (Source: [194]). (Part 2) Growth factors (HBEGF: heparin-binding EGF-like growth factor).
Stem cell factor | Melanocyte growth |
Nerve growth factor | Modulation of mastocyte activity, |
hair growth, | |
neuron growth and differentiation | |
Fibroblast growth factor-2/5/7/10 | Tissue remodeling |
Transforming growth factor-β | Wound healing |
Vascular endothelium growth factor | Blood vessel permeability, angiogenesis |
Platelet-derived growth factor | Fibroblast growth, angiogenesis |
Epidermal growth factor | Keratinocyte growth and differentiation |
HBEGF | Keratinocyte growth and differentiation |
Granulocyte–macrophage | Langerhans cell migration and maturation, |
colony-stimulating factor (CSF2) | angiogenesis |
3.8.3.2 Skin Mastocytes
Mastocytes in the skin exhibit a distribution gradient. The smaller the distance to epidermis, the denser the population. Skin mastocytes at the vanguard of the body’s defense display multiple receptors and secrete numerous products (Table 3.17).
Table 3.17
Skin mastocyte receptors and products (Source: [196]; FcεR: IgE Fc receptor; gmCSF: granulocyte–monocyte colony-stimulating factor; IL: interleukin; LT: leukotriene; PG: prostaglandin; TNF: tumor-necrosis factor).
Products | Receptors |
---|---|
Histamine | FcεR1/2 |
Heparin | H2, H4 |
Tryptase, chymase | PAR2 |
Carboxypeptidase-A | C5a R |
Cathepsin-G | |
IL1β, IL5, IL6, IL8, IL13 | |
TNFα | |
gmCSF | |
Proopiomelanocortin | |
Substance-P | |
PGd2, LTc4 | |
Endothelin-1 | |
Neurotrophins |
Under normal conditions, skin mastocytes are preferentially located close to nerves, especially sensory nerve endings,91 blood vessels, and hair follicles. Hair follicle cycling during periods of rest and growth, which is associated with proteolysis, angiogenesis, and nerve rearrangement, is impaired in the absence of mastocytes. Mastocytes actually participate in tissue repair and remodeling. Mastocytes are associated with sensory nerves. Mastocyte clusters can be innervated directly (Table 3.18).
Table 3.18
Interactions between mastocytes and the nervous system (Sources: [197]). Mastocytes are associated with nerves in the skin and blood vessels in the brain, especially in meninges. Mastocytes abound around the pituitary gland, where they can control the hypothalamo–pituitary–adrenal axis. Mastocyte regulators include not only pathogens (mainly parasites and bacteria), adhesion molecules, extracellular matrix proteins, nitric oxide, and cytokines, but also neurotransmitters and neurotrophins. Substance-P released from nerve endings promotes mastocyte-dependent granulocyte infiltration. Hypothalamic mastocytes localize close to nerve endings that contain corticotropin-releasing hormone. This hormone is released not only by hypothalamus, but also dorsal root ganglia. It not only activates skin mastocytes but also acts on endothelial cells. Mastocyte proliferation can be triggered by nerve growth factor released from leukocytes and nerve endings. Neuropeptides released from nerves can induce liberation of chymase and production of E-selectin in adjacent microvascular endothelia similarly to substance-P release from axons with subsequent priming of cytokine-mediated interaction between mast and endothelial cells. However, calcitonin gene-related peptide reduces antigen-presenting capability of mastocytes by causing degranulation and release of IL10 and TNFα. Endothelin-1 is a major activator that causes mastocyte degranulation.
Mastocyte | Nerve |
---|---|
Histamine | Substance-P |
Serotonin | Endothelin-1 |
Peptidases | Urocortin |
TNFα | Neurotensin |
IL1/6 | Nerve growth factor |
Corticotropin-releasing factor |
3.8.3.3 Arterial Wall Mastocytes
In normal arterial intima, only a few mastocytes are present (average density ∼ 1 cell/mm2, i.e., much smaller density than that in skin ∼ 50 cell/mm2) [198]. However, in atheroma, mastocytes, together with macrophages and T lymphocytes invade the intima.
3.8.3.4 Cardiac Mastocytes
Maturation of resident cardiac mastocytes is associated with formation of chymase and histamine as well as the loss of division capacity. Four stages of mastocyte maturation can be defined [200]: stage 1 in which immature cells have the smallest size, 2 in which still immature cells are able to divide and possess granules, 3 in which non-dividing cells are endowed with abundant granules, and 4 characterized by fully differentiated, mature cells.
Cardiac mastocytes that synthesize, store, and release activerenin trigger a (non-kidney-derived) cardiac renin–angiotensin system. Local generation of cardiac angiotensin-2 from mastocyte-derived renin causesnoradrenaline secretion from isolated sympathetic nerve terminals via AT1 receptors [199].
Cardiac mastocytes synthesize various growth factors, cytokines, chemokines, nitric oxide and other vasoactive molecules, peptidases, fatty acid metabolites, and other mediators involved in tissue remodeling. Mastocytes store and release several agents that can activatematrix metallopeptidases that degrade the collagen matrix [200].
Mastocyte peptidases, such aschymase andtryptase, can activate zinc-dependent interstitial collagenase-1 (MMP1), gelatinases (MMP2), and stromelysin (MMP3).Matrix metallopeptidases are produced and released as inactive zymogens; their propeptide domain is removed to obtain an active enzyme. Chymase activates proMMP1; tryptase cleaves proMMP3. Peptidase MMP3 then activates other proMMPs, in particular, activating MMP1 enzyme. In addition, mastocytes can synthesize and secrete MMP1 peptidase. Moreover, chymase and tryptase degrade fibronectin and vitronectin. Chymase is also capable of cleaving inactiveangiotensin-1 to active angiotensin-2. In addition, mastocytes produce TNFα that activate MMP9 synthesized from themselves and adjacent macrophages.
Identified secretagogues encompass endothelin-1, reactive oxygen species, interleukin-33, complement factor-5A, and several neuropeptides [200].Endothelin-1 operates via its ETA receptor and activates cardiac mastocytes.Reactive oxygen species (oxidative stress) can induce mastocyte degranulation.Interleukin-33, a member of the IL1 family of cytokines, regulates mastocyte function.
The complement molecule, C5a, a proteic fragment released from complement component C5, is a chemoattractant for mastocytes, an anaphylatoxin that causes histamine release from mastocytes, and a potent inflammatory mediator. It binds to a Gi-coupled receptor (C5aR or CD88) of target cells such as granulocytes.
Among neuropeptides,substance-P, which is released predominately from afferent fibers of the sympathetic nervous system, provokes degranulation of cardiac mastocytes.Neurotensin, which is produced in nerve fibers of the intracardiac ganglia and coronary vasculature, as well as those that innervate cardiomyocytes and nodal cells, also causes a calcium-dependent degranulation of cardiac mastocytes, in addition to its positive inotropic effects mediated by noradrenaline release.
Cardiac mastocyte density rises in the left ventricle maladaptive hypertrophy (e.g., upon mitral regurgitation [mitral insufficiency or incompetence]) that provokes congestiveheart failure. The myocardial MMP2 activity elevates with subsequent collagen degradation [200].
Cardiac mastocytes are also involved infibrosis developed in maladaptivehypertrophy caused by arterial hypertension. Mastocyte density is correlated to stem cell factor and SCFR receptor levels. Mastocytes can prevent macrophage recruitment [200]. Mastocytes regulate fibrosis that results from pressure overload via inflammatory cell recruitment and direct action on fibroblasts.
Mastocytes participate with monocytes, macrophages, and lymphocytes in inflammation duringatherosclerosis. They can provoke plaque destabilization with increased risk of rupture, in addition to their role in the development of aortic abdominalaneurysms.
Cardiac mastocyte density also augments during myocardial infarction, in particular during the healing phase [200]. Recruited macrophages and neutrophils gather near degranulated mastocytes. Mastocytes releaserenin and chymase that form myocardial angiotensin-2. The latter elevates noradrenaline levels, hence risk of arrhythmias. Renin and noradrenaline release indeed prime ventricular fibrillation during ischemia–reperfusion events. Mastocytes also contribute to the regulation of myofibroblast activity.
3.8.3.5 Airway Wall Mastocytes
Human lung mastocytes that reside predominantly in the submucosal connective tissue of normal human bronchi synthesizerenin that triggersangiotensin formation after degranulation in airways [201]. Local generation of angiotensin-2 from mastocyte renin primescontraction of bronchial smooth myocytes via AT1 receptors.92 The airway renin–angiotensin system thus complements the traditional, circulating renin–angiotensin axis, in which renin is released into the blood circulation from the kidneys in response to decreased renal perfusion pressure, reduced delivery of NaCl at the macula densa, and/or increased renal sympathetic nerve activity. The pulmonary endothelium is the primary source of angiotensin-converting enzyme in the body.
In allergic asthma, mastocytes release renin, histamine, β-hexosaminidase, tryptase, leukotrienes LTc4 and LTd4, adenosine, cytokines, and kinins that elicit acute and chronic airway constriction and inflammation.
3.9 Lymphocytes
Lymphocytes (L
; size 8–15 μm) have a very large nucleus. Lymphocyte is much smaller than the 3 types of granulocytes. They account for 25–35% of leukocytes. They regulate adaptive immunity. The major production sites of T and B cells are the thymus and bone marrow, respectively. Both B and T lymphocytes reside in lymph nodes and in the spleen, where they mature into fully functional cells.

Cell history can lead to tissue-specific cells according to the residential type of lymphoid organs, but this property can be reverted. Effector cell behavior is influenced by cytokines involved in helper TH1 and TH2 responses. T-cell exit from lymphoid and peripheral tissues requiressphingosine 1-phosphate and chemokine CC-motifreceptor CCR7, respectively.
B lymphocytes as well as some types of T lymphocytes, such as CD4 + , CD8 + , α/β, γ/δ, and NK (natural killer) T cells, constitute distinct lineages that do not interconvert. On the other hand, other T-cell lineages can remodel in other T-cell types, such as helper and inducible TReg lymphocytes.
Both CD4 and CD8 that bind to major histocompatibility complex molecules lodge on immature, double-positive thymocytes. Double-positive thymocytes with a T-cell receptor that interacts weakly with self-peptides bound to MHC molecules undergo a positive selection to become single-positive CD4 + or CD8 + T lymphocytes. Double-positive thymocytes with a TCR that links strongly to self-peptide bound to MHC molecules undergo a negative selection and apoptosis, thereby preventing the development of self-reactive T lymphocytes. Positive selection relies on a moderate, sustained Ca2 + import and ERK activation [202]. On the other hand, high-affinity TCR ligands — negative selectors — cause high magnitude, short duration Ca2 + import and ERK activation. Increase in cytosolic Ca2 + concentration induced by TCRs that activates T cells results from the stimulation of the PLCγ1–IP3 axis.93 In response to weak TCR ligands,NaV1.5 bound to the regulatory NaVβ4 subunit favors a sustained calcium entry [202].94
3.9.1 Selection between CD4 and CD8 Coreceptors
Coreceptors CD4 and CD8 intervene in the recognition of major histocompatibility complex class-2 and -1 ligands, respectively, as well as the recruitment of theleukocyte-specific non-receptor Tyr kinase LCK to the TCR complex. Coreceptors CD4 and CD8 have different capacity to recruit LCK to the TCR, thus directing lineage decision [203]. In addition, differentiation into the CD8 + lineage requires the TCR-induced increased density of the70-kDa TCR ζ-chain-associated protein (ZAP70). In the thymus, CD4 + and CD8 + cells arise from distinct subsets of double-positive thymocytes that possess different kinetics and sensitivities to stimulation of T-cell receptors. ZAP70 kinase determines the cell sensitivity to TCR stimulation, thereby enabling the selection of CD4 + and CD8 + fate [204].
3.9.2 Sensing of Endo- and Exogenous Molecules
Immunocyte receptors are able to sense microbial components (danger signals) as well as endogenous molecules generated during injury (damage signals) such as ribonucleoprotein complexes released from dead cells. Self nucleic acids must not be mistaken for microbial products to avoid autoimmunity. Endogenous agonists ofToll-like receptors TLR2 and TLR4 probably trigger signals distinct from those of microbial ligands, hence may not activate adaptive immune responses due to the engagement of different coreceptors. On the other hand,tumor cells produce small-molecular-weight fragments of hyaluronic acid, biglycan, and versican that can stimulate TLR2 and/or TLR4 receptors [205].
T cells can recognize 3 types of substances [206]: (1) ligands of MHC-related and -unrelated γδ T-cell receptors, such as MHC class-1-like CD1c plasmalemmal glycoprotein and phosphoantigens; (2) various types of cell-surface molecules, such as retinoic acid early transcript RAE1 and MHC class-1 polypeptide-related sequence MICA that engage natural killer receptors (NKR; e.g., NK group-2 member-D [NKG2d] that is also called killer cell lectin-like receptor KLRk1); and (3) danger- (DAMP) and pathogen (PAMP)-associated molecular patterns sensed by pattern recognition receptors, such as Toll-like receptors anddectin-1.95
Pathogen-specific innate immune recognition activates antigen-specific adaptive immune responses. Pattern recognition receptors (Vol. 3 – Chap. 11. Receptors of the Immune System) of the innate immune system detect relatively invariant molecular patterns found in most microorganisms of a given class, the so-called pathogen-associated molecular patterns.
Microbial pattern recognition receptors can be categorized into [205]: (1) secreted (collectins,ficolins, andpentraxins); (2) transmembrane (Toll-like receptors andC-type lectins); and (3) cytosolic types (retinoic acid-inducible gene RIG-1-like [RLR] and nucleotide-binding domain and leucine-rich repeat-containing [NLR] receptors).
Cytosolic pattern recognition receptors — RLRs — detect viral material such as RNA, recruit adaptor Mitochondria antiviral signaling protein (MAVS), and activate nuclear factor-κB and interferon regulatory factor IRF3 [205].
Intracellular sensors — NLRs — recognize degradation products of peptidoglycans and microbial products, among others.
Secretedpattern-recognition receptors bind to microbe surfaces, activate classical and lectin pathways of thecomplement system, and opsonize pathogens forphagocytosis by macrophages and neutrophils.
Transmembrane pattern recognition receptors reside either in the plasma membrane or onendosomes andlysosomes. Plasmalemmal TLRs recognize conserved microbial patterns accessible on the cell surface, such as lipopolysaccharides of Gram − bacteria (TLR4), lipoteichoic acids of Gram + bacteria, bacterial lipoproteins (TLR1–TLR2 and TLR2–TLR6), and flagellin (TLR5) [205]. Endosomal TLRs detect microbial nucleic acids, such as double-stranded RNA (TLR3), single-stranded RNA (TLR7), and dsDNA (TLR9). C-type lectin domain-containing proteins CLec7a and CLec6a (dectin-1 and -2) sense β-glucans and mannan on fungal walls, respectively [205].
Most, if not all, pattern recognition receptors activate the transcription factors nuclear factor-κB,interferon regulatory factors, ornuclear factor of activated T cells to induce both T- (e.g., TH1, TH17, and CD8 + T-cell responses) and B-cell responses (IgM, IgG, and IgA immunoglobulin–antibody responses) [205]. Secreted and some endocytic pattern recognition receptors, such as scavenger and mannose receptors, cannot induce adaptive immunity by themselves.
Three signals are required for cross-priming that permits dendritic cells to present exogenous antigens to responding antigen-specific CD8 + T cells.96 Signal 1 (MHC–AG–TCR) constitutes the complex made of peptidic antigen and major histocompatibility complex class-1 molecule that targets the relevant TCR. Signal 2 (costimulator) refers to costimulatory molecules such as B7-family members that ligate T-cell-specific surface glycoprotein CD28. Signal 3 (inflammatory cytokine) corresponds to triggering soluble factors, such as interleukin-12 and interferon-α and-β. However, this process needs help that is provided by CD4 + T cells, plasmacytoid dendritic cells, and natural killer T cells that stimulate chemokine release from cognate dendritic cells (signal 0: chemokine gradient), i.e., upstream from signal 1, to attract CD8 + T cells [207].
3.9.3 Resistance to Oxidizing Environment
Lymphocytes are exposed to oxidizing environments during inflammation. Helper T lymphocytes become progressively less sensitive to an oxidizing environment after differentiation into effector T cells, which affects their ability to proliferate, differentiate, and secrete cytokines.
In human helper T lymphocytes, calcium influx through Orai family member-based channels is involved in the cell activation, proliferation, and differentiation.Orai calcium release-activated calcium channel modulator-1, but not Orai3, is inhibited by oxidation byreactive oxygen species [208]. The decreased redox sensitivity of effector TH cells is correlated with increased amounts of Orai3 and several cytosolic anti-oxidants.
3.9.4 PIP 3 and IP 4 Signals in Lymphocytes
Membrane lipid phosphatidylinositol (3,4,5)-trisphosphate (PIP3) regulates membrane receptor functioning, in particular immunoreceptor signaling. Solubleinositol (1,3,4,5)-tetrakisphosphate (IP4) participates in the development and activity of leukocytes, such as T and B cells and neutrophils, as it controlsphosphoinositide 3-kinases,phosphatase and tensin homolog (PTen),SH2-domain-containing inositol 5-phosphatases SHIP1 and SHIP2 that target PIP3 in lymphocytes [209]. Agent IP4 regulates the membrane recruitment of proteins, as it promotes or impedes their interactions with PIP3 Lipid.
In mouse thymocytes, membrane recruitment ofinducible T-cell kinase (ITK) by IP4 achieves apositive feedback loop onphospholipase-Cγ1 downstream from T-cell receptors to produce sufficient amount of the second messenger diacylglycerol to trigger positive selection and generation of a mature T-cell directory [209]. In humans,inositol (1,4,5)-trisphosphate 3-kinase ITPKc limits T-cell activation level. Its defect contributes to Kawasaki disease in children. Inositol trisphosphate 3-kinase ITPKb is required for B-cell development and functional competence, as it restrains store-operated Ca2 + entry as well as PIP3-mediated membrane recruitment ofprotein kinase-B in neutrophils [209].
3.9.5 Immunological Memory
Lymphocytes provide antigen-specific acquired immunity, hence immunological memory. Immature T- and B-cell activity is restricted mainly to lymphoid tissues. Activated T cells migrate more efficiently than antigen-unexperienced T cells. Phenotype and certain features allow to distinguish long-lived memory B and T cells from short-lived effectors. Antigen-experienced T cells include: (1) central memory cells that circulate through lymphoid tissues and (2) effector memory cells that do not move.
Immunological memory confers long-lasting protection. Naive cells of the immune system that have not been previously exposed to a given antigen actually learn from the first experience of encountering a pathogen to adapt and react quickly to future invasion. Cells of the adaptive immune system undergo a secondary expansion and trigger a robust protective response when they re-encounter the same pathogen. The adaptive immune system is thus characterized by an antigen-specific memory response.
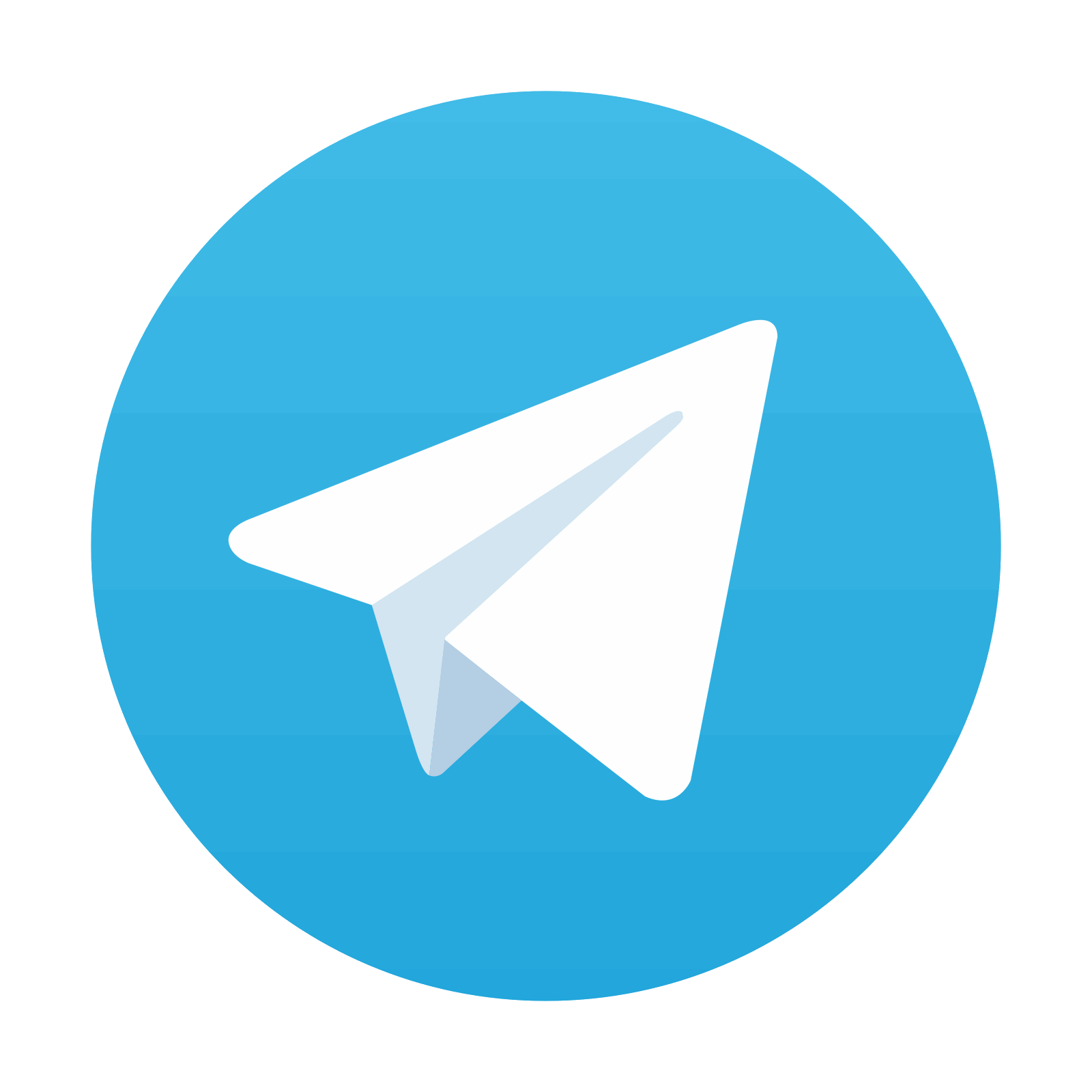
Stay updated, free articles. Join our Telegram channel

Full access? Get Clinical Tree
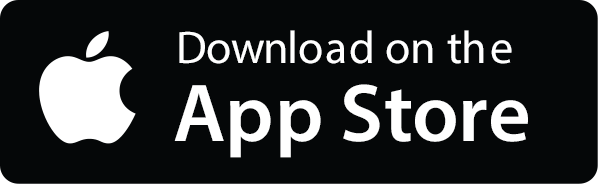
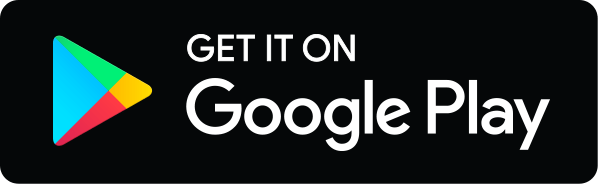