Blood component
% By volume
Principle function
Low %volume
High %volume
Plasma
50–60
Fluid base for blood, carbon dioxide and nutrient transport
Dehydration
Blood loss
Excess salt loss (e.g. after sport)
Kidney disease
Red cells
40–50
Carrying oxygen
Blood loss
Sickle cell anaemia
Enlarged spleen
Cancer
Polycythaemia
Chronic hypoxia
Blood doping
Dehydration
White cells
0.7
Immune system
Medication and radiation treatment
Immune dysfunction (e.g. AIDS)
Toxins including alcohol
Major surgery
Infection (normal)
Genetic disorders
Leukaemia
Spleen removal
Platelets
0.3
Clotting of blood
Medication and chemotherapy
HELLP syndrome
Haemolytic-uremic syndrome
Snakebite
Thrombocytosis
Macromolecules: albumin
2
Maintenance oncotic pressure
Reduced production (various diseases)
Dehydration
Other
1.5
Various

Fig. 3.1
Blood cells. Several types of blood cells are shown including red cells (erythrocytes), two different white cells (neutrophils and a monocyte) and platelets. Image kindly provided by Karen Hart, Peninsula College, Port Angeles, WA, USA. © 2006–2010 Karen Hart
3.1.1 Plasma
Plasma is a straw coloured fluid and consists of 90 % water and 1 % electrolytes, with various molecules making up the remainder. Electrolytes play an important role in ensuring the correct fluid content within cells; consequently, electrolyte concentration is subject to several control mechanisms. Low plasma volume may arise from a number of causes including dehydration, salt depletion (e.g. following sport), or blood loss. High plasma volume can occur as a result of inadequate salt excretion associated with kidney disease.
3.1.2 Macromolecules and Other Molecules
Macromolecules make up around 9 % of the plasma volume. The majority of molecules are proteins, such as globulins (part of the immune system), albumin (important for maintenance of oncotic pressure) and fibrinogen (part of the clotting system). Also present are a number of molecules in transit such as vitamins, hormones, waste products (urea, ammonia), carbon dioxide and oxygen. After a meal there is an increase in volume of fatty acids, amino acids and peptides arising from digestion in the blood. Some of these molecules such as LDL (low density lipoprotein) cholesterol play important roles in arterial disease.
Albumin is important in regulating the balance of fluids in the body. Low levels of albumin in blood are due to lack of protein in the diet or due to impaired production from the liver or to kidney disease. Low albumin levels result in an imbalance of fluids resulting in generalised swelling of tissues as a result of water retention, known as ‘oedema’. A high level of albumin may arise from excess protein in the diet, but are usually caused by dehydration where the fluid levels in the tissues are low.
3.1.3 Red Cells
The principle particle in blood is the red cell or erythrocyte. The percent by volume of red cells is called the haematocrit, the packed cell volume or the erythrocyte volume fraction. The haematocrit has a normal range of 41–52 % in men and 36–48 % in women. The red cell is unique as, unlike other cells it has no nucleus and it has a biconcave shape (Fig. 3.2). Human red cells, have a diameter of 7.5 μm, a thickness of 2.0 μm and an effective diameter (i.e. the diameter if the red cell contents were formed into a sphere) of 5.5 μm. The size and shape of red cells is remarkably similar across all mammals; the diameter of a red cell in a mouse and an elephant is about the same. Red cells are involved in the transport of oxygen from the lungs to the tissues, for which the iron in the red cell plays an important role. Low haematocrit has a number of causes, including blood loss and various diseases. High haematocrit leads to an increase in the oxygen carrying ability of the blood, which is the reason why some athletes involved in endurance events have blood transfusions before the race. However it is also risky, as the viscosity of blood is higher, leading to greater resistance to flow and increased risk of clinical events such as heart attack and stroke. Sickle cell disease is associated with abnormally shaped red cells in the form of a sickle rather than a disc. While the oxygen carrying capability is impaired, the presence of sickle cells gives immunity against malaria, a deadly disease which operates through destruction of red cells.


Fig. 3.2
Red cells. a Red cell shape and dimensions. b Red cells at low shear. (b) from; Krüger (2015); © Springer-Verlag Berlin Heidelberg 2015, with permission of Springer
3.1.4 White Cells
White cells, or leukocytes, occupy some 0.7 % of the blood volume in health. There are a number of different leukocytes as shown in Fig. 3.3, each with a different role. Neutrophils (diameter 10–12 μm) ingest and digest bacteria and fungi. Eosinophils (diameter 10–12 μm) attack larger parasites and are involved in allergic responses. Monocytes (diameter 15–30 μm) are carried by the cardiovascular system to different tissues where they transform into cells called macrophages. Lymphocytes (diameter 7–15 μm) attack invading bacteria and viruses and also help destroy cells in the body which have become diseased through virus infection or cancer. Basophils (diameter 12–15 μm) are involved in response to allergic symptoms including histamine release. An increase in the volume fraction of white cells is a normal response to infection and is not usually harmful. However abnormal increases occurring in diseases such as leukaemia may be fatal. Low white cell volumes are due to decreased production or increased destruction arising from various diseases and lead to impaired functioning of the immune system.


Fig. 3.3
White cells; illustration of various types of white cell. Image reproduced from wikipedia with permission. Original image authored by Bruce Blausen: Wikiversity Journal of Medicine. doi:10.15347/wjm/2014.010. ISSN 20018762.—Own work, CC BY 3.0, https://commons.wikimedia.org/w/index.php?curid=28223981
3.1.5 Platelets
Platelets are fragments of much larger cells called megakaryocytes and occupy 0.3 % of the blood volume in health. Platelets exist in unactivated and activated forms (Fig. 3.4). The majority of circulating platelets are unactivated and their shape is plate-like (hence the name ‘platelet’). These have a greatest diameter of 2–3 μm. Once activated, they become sticky and more spherical with projections (pseudopods). These projections are important in enabling activated platelets to clump together. Platelets are involved in blood clotting and in the repair of damaged endothelium. If the endothelium is damaged, underlying collagen fibres are exposed. Unactivated platelets coming into contact with collagen become activated and will stick to the collagen sealing off the damaged area after which repair of the area ensues. Platelets can also be activated by increases in wall shear in narrowed vessels. Low platelet volume fraction can arise from a number of diseases and lead to impaired clotting ability, leading to potentially life-threatening blood loss from minor wounds. Increased platelet volume fraction arising from disease leads to increased risk of thrombosis which in turn may lead to potentially life-threatening clinical events such as heart attack and stroke.


Fig. 3.4
Platelets; illustration of unactivated and activated platelets. Image reproduced from wikipedia with permission. Original image authored by Bruce Blausen: Wikiversity Journal of Medicine. doi:10.15347/wjm/2014.010. ISSN 20018762.—Own work, CC BY 3.0, https://commons.wikimedia.org/w/index.php?curid=28223979
3.2 Forces on Blood Particles
This section examines the forces on particles and discusses these in the context of blood viscosity and blood flow. The examples below are drawn from a variety of sources including in vitro experimental flow systems, computational modelling and in vivo measurements.
3.2.1 Forces Associated with Gravity
Gravity is a long-range force affecting all particles in a fluid in the lab and in the human body on planet earth (and any other planet or moon humans choose to live on). The forces on a particle arising from gravity are:
Gravitational force. The weight of the particle will tend to make the particle fall in a gravitational field.
Buoyant force. The hydrostatic pressure (difference in pressure in the fluid due to height difference) tends to make the particle rise in a gravitational field.
These forces are illustrated in Fig. 3.5. If the particle density is greater than the fluid density then the overall force will cause the particle to sink. Conversely if the particle density is less than the fluid density, then the particle will rise. When the particle density is the same as the density of surrounding fluid, there is no net force on the particle and the particle is said to be ‘neutrally buoyant’. The density of an average red cell, at 1125 kg m−3, is slightly higher than that of plasma at 1025 kg m−3, and so red cells have a slight tendency to sink.


Fig. 3.5
Forces on a particle initially at rest in a stationary fluid
3.2.2 Forces Associated with the Velocity and Shear Field at High Reynolds Number
Forces arising from the velocity and shear field have different effects depending on the flow conditions in the vessel. In the following sections the forces will be based on Reynolds number. It will be recalled that Reynolds number (Re) is the ratio of inertial to viscous forces. Effects due to inertia of the fluid (present at high Re) are most prevalent in larger vessels and will be considered in this section. Effects relevant at lower Re (<1) where viscous forces dominate, are most prevalent in the microcirculation and will be considered in the next section. Figure 3.6 shows the principal forces arising from motion of the fluid. The forces are as follows:


Fig. 3.6
Inertial forces arising from motion of the fluid with respect to the particle. a Drag force. b Shear induced lift. c Wall induced lift. Note that lateral motion of the particle will also give rise to a drag force
Drag force. When there is relative motion between the fluid and the particle, the particle experiences a force in the direction of the flow as a result of the inertia of the particle. If a fluid is at rest and is subject to a pressure gradient, the fluid will accelerate. The particle experiences a force in the direction of the fluid motion which accelerates the particle until there are no forces in the direction of motion. If there is lateral motion of the particle (i.e. across streamlines) then this relative motion will also lead to a drag force.
Shear-induced inertial lift. When a particle is in a shear field the difference in shear on either side of the particle causes the particle to spin. There is an associated force perpendicular to the direction of fluid motion which causes the particles to migrate to higher shear rate regions. The force originates from inertial effects in the fluid surrounding the particle. Ho and Leal (1974) provided one of the first theoretical formulations which gave good agreement with experimental data.
Wall-induced inertial lift forces. A number of different inertial forces are present on a particle near a wall or a particle touching a wall. All these forces are directed away from the wall leading to motion of the particle away from the wall. These include lift on a non-spinning particle near a wall (Cherukat and McLaughlin 1994), lift on a spinning particle near a wall (called the Magnus effect), and lift on a particle touching the wall (Leighton and Acrivos 1985).
In 1962, Segre and Silberberg published a paper on flow of neutrally buoyant solid particles in a vertical tube. The particles were uniformly distributed at the inlet to the tube but, further along, they located in a ring of particles at a distance of 0.6 of the tube radius from the centre. This was termed the ‘tubular pinch effect’ and subsequently became known as the Segre-Silberberg effect. The pinch effect was strongest for Re numbers of less than about 30. For higher Re the width of the particle-free region near the wall increased and there was spreading of the particles throughout the whole cross section of the tube. The explanation for this effect concerns inertial forces which push the particle across the streamlines (di Carlo 2009). Particles experience a shear-induced lift force which pushes the particles away from the centre. Particles near the wall experience a lift force as a result of the presence of the wall which pushes them away from the wall. The particles locate at a distance where these 2 forces balance each other (Fig. 3.7). At higher Reynolds numbers, the balance of lift forces changes and the equilibrium position moves towards the wall. Higher Reynolds number flows of 500–2000 is associated with an inner annulus and a more uniform distribution (Matas and Morris 2004). These effects are seen at low volume concentrations, less than 20 %, where particle–particle interaction can be neglected. However, any particle suspension at Re > 1 will be subject to these lift forces to some degree, which will lead first, to particle depletion at the wall and second, to inhomogeneity in particle concentration.


Fig. 3.7
Overall inertial lift force on a particle in Pouiseille flow in a cylinder for a dilute suspension of particles; at low shear the equilibrium position is at 0.68 of the diameter corresponding roughly to the Segre-Silberberg position. At higher shear the equilibrium position moves nearer to the wall. From Matas and Morris (2004); reproduced with permission
3.2.3 Forces Associated with the Velocity and Shear Field at Low Reynolds Number
At low Reynolds numbers < 1, flow is dominated by viscous effects. Forces arising from inertial effects, such as the lift forces discussed in Sect. 3.2.2, do not occur. A formal explanation for this is that at very low Re, fluid flow is governed by Stokes flow which is time reversible for spherical objects meaning that viscous forces cannot operate (see, e.g. Cantat and Misbah 1999). Inertial-based lateral motion of a particle is therefore impossible. However, viscous lateral motion is possible and mainly relevant to deformable particles. The forces leading to lateral motion are described here. For further reading see Vlahovska et al. (2009).
Drag force. Relative motion between the particle and the fluid will result in a drag force due to the viscous forces between the fluid and the particle surface.
Lift due to loss of particle symmetry. An initially spherical particle which is deformable will become elongated as a result of the difference in drag on the particle from the wall and the non-wall side. In particular, the particle will have upstream–downstream asymmetry resulting in a viscous lift force (Olla 1997; Cantat and Misbah 1999).
These forces contribute to the creation of a cell-free layer in the microcirculation where viscous flow dominates, discussed further in Chap. 8.
3.2.4 Chemical and Electrical Forces
These forces generally operate at close range and arise through interaction between particles, rather than through the dynamic behaviour of the fluid in which the particles are suspended. Figure 3.8 illustrates these two forces.


Fig. 3.8
Electrostatic and chemical forces on a particle in a fluid
Electrostatic forces. Electrically charged particles will repel similarly charged particles and attract particles of opposite charge. Some particles may have overall neutral charge but the charge can be split in the form a dipole with a positive and negative end. In this case the positive and negative ends of adjacent particles attract and the similarly charged ends repel.
Molecular binding forces. These concern the binding of one biological cell or molecule with another biological cell or molecule.
Electrostatic forces are important in that red cells, white cells and platelets all have a negative charge. This helps to keep them apart and helps prevent thrombus formation in the normal circulation. Electrostatic forces are also the origin of a lift force on deformable particles near a wall. If a particle deforms in shear flow near a wall, an electrostatic dipole will be produced which interacts with the mirror of the dipole in the wall producing a lift force (Leal 1980).
Molecular binding forces are especially relevant in blood flow and three phenomena will be considered in more detail; Rouleaux formation, leukocyte adhesion and platelet aggregation and adhesion.
Rouleaux formation
Red cells at low shear clump together face to face to form rouleaux (Fig. 3.13). A review by Wagner et al. (2013) considers two explanations for this; the bridging model and the depletion model. Rouleaux formation in vivo is thought to require the presence of albumin and fibrinogen. If plasma is replaced with an isotonic saline solution then rouleaux formation does not take place. In the bridging model, albumin and fibrinogen are adsorbed onto the surface of the red cell. There is chemical bonding between the molecules on the surfaces of adjacent red cells, which is sufficient to overcome the electrostatic repulsion resulting in adhesion of red cells and rouleaux formation. In the depletion model, a randomly low concentration of albumin and fibrinogen concentration gives rise to a depletion force (see Sect. 3.2.5), which results in red cell adhesion and rouleaux formation.
Leukocyte adhesion
Activated white cells travelling close to the vessel wall form molecular links with endothelial cells. These links are formed through bonding between ligands on the white cell and selectin molecules on the endothelium. If other forces (e.g. related to particle inertia and shear) are greater than the molecular binding force then the link is broken, the white cell rolls along the endothelium until a link is re-established. This repeatedly happens and the white cell rolls along the endothelium until either the shear forces are sufficient to detach the white cell or the molecular links are strong enough to prevent the cell from rolling further. Leukocyte adhesion is a necessary step in the migration of leukocytes through the endothelium.
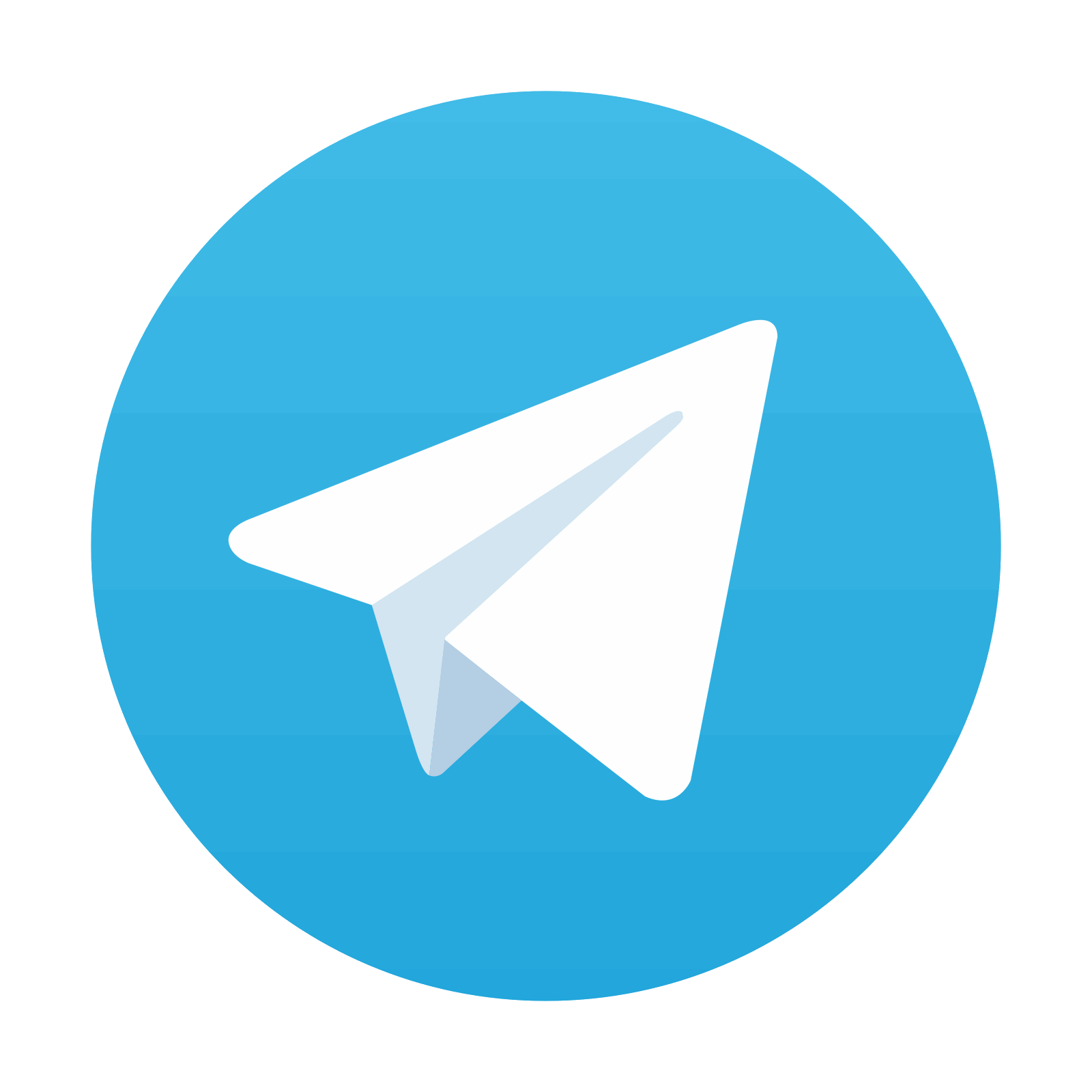
Stay updated, free articles. Join our Telegram channel

Full access? Get Clinical Tree
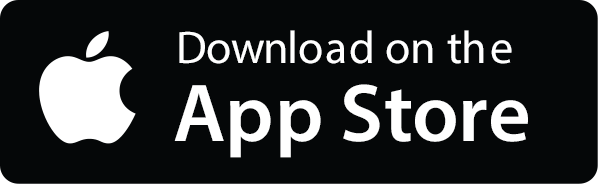
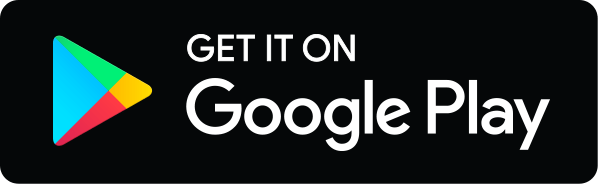