Background
The presence and progressive nature of primary myocardial involvement in Marfan syndrome are debated. The aim of this study was to evaluate the clinical relevance of left ventricular (LV) and right ventricular (RV) strain in adult patients with Marfan syndrome without significant valvular disease.
Methods
Adult patients with Marfan syndrome ( n = 50; mean age, 35.2 ± 12.9 years) were followed prospectively. Echocardiography was performed annually and consisted of comprehensive assessment of ventricular and valvular function. Using speckle-tracking imaging, the baseline strain values of the Marfan population were calculated and compared with the values of normal controls. The follow-up evaluations were used to assess changes in ventricular strain. The association between the incidence of adverse events (heart failure, [supra]ventricular arrhythmias, and proximal aorta surgery) and baseline strain values was investigated.
Results
Compared with controls, patients with Marfan syndrome had significantly lower peak longitudinal LV strain (−18.9 ± 2.3% vs −20.1 ± 1.9%, P < .01) and RV strain (±26.9 ± 5.2% vs ±29.3 ± 4.25%, P < .01). The absolute changes in LV longitudinal, radial, and circumferential strain and RV longitudinal strain during a median 4 years of follow-up were 0.1 ± 2.8%, 1.12 ± 7.6%, 0.3 ± 3.7%, and 0.9 ± 5.5%, respectively, which was not statistically significant. Cox regression demonstrated that reduced LV or RV strain was not associated with adverse outcome (supraventricular arrhythmias, n = 3; proximal aorta surgery, n = 4).
Conclusions
This study suggests that patients with Marfan syndrome show lower ventricular strain and strain rate values compared with healthy controls. However, no relevant changes in LV and RV function occurred during midterm follow-up in patients with Marfan syndrome without valvular disease at baseline. Although ventricular strain and strain rate were mildly reduced in patients with Marfan syndrome, this did not affect outcomes negatively in the present study.
Marfan syndrome is a systemic connective tissue disorder caused by mutations in the fibrillin-1 gene. Besides abnormalities in the musculoskeletal and ocular systems, the cardiovascular phenotype consists of widening of the aorta and pulmonary artery and of abnormalities in the mitral valve. In addition, studies of primary myocardial involvement have revealed that ventricular function may also be impaired in patients with Marfan syndrome. Several investigations in which conventional echocardiography was used indicated that left ventricular (LV) diameters can be increased and that LV diastolic function can be impaired. More recent analyses in which myocardial deformation was assessed using novel echocardiographic techniques demonstrated that LV and right ventricular (RV) systolic ventricular function may also be reduced in patients with Marfan syndrome compared with controls.
In light of these study data, it is advocated to regularly perform follow-up echocardiographic evaluations, to detect decreased ventricular function in a timely manner. Nonetheless, the largest longitudinal follow-up study so far, conducted in 234 patients with Marfan syndrome, did not demonstrate any significant changes in LV diameters and function during a median follow-up duration of 6 years. This questioned whether the ventricular abnormalities that were observed in the Marfan population had a progressive nature. However, it should be noted that this study relied on conventional echocardiography, whereas recent investigations have indicated that small changes in ventricular function may go undetected using these methods. In recent years, echocardiographic speckle tracking–derived strain imaging was introduced as a novel modality that can be used to detect clinically relevant but modest changes in ventricular performance. The aim of the present study was threefold: (1) to assess the differences in LV and RV performance, quantified using speckle tracking–derived strain and strain rate imaging, between patients with Marfan syndrome and healthy controls; (2) to investigate the association between biventricular strain and strain rate on one hand and the clinical outcomes of patients with Marfan syndrome on the other hand; and (3) to analyze whether any relevant changes in LV and RV performance occur in patients with Marfan syndrome, using both conventional echocardiographic measures of ventricular function as well as speckle tracking–derived strain and strain rate imaging.
Methods
Study Population
The study population consisted of all patients with Marfan syndrome who where prospectively followed at the outpatient clinic from January 1, 2000, until June 11, 2009. Marfan syndrome was diagnosed according to the Ghent criteria, and genetic testing for Marfan-associated mutations was performed in all patients. To investigate whether potential changes in ventricular function were related specifically to Marfan syndrome, only patients without significant valvular regurgitation or stenosis were selected. Patients with significant valvular heart disease, including moderate and severe regurgitation and any grade of valvular stenosis, were excluded. Valvular heart disease was evaluated with color, continuous, and pulsed-wave echocardiographic Doppler techniques, applying the reference measures indicated by current recommendations. The other criteria for exclusion from the analysis were a follow-up duration of <2 years; documented coronary artery disease or a history of coronary artery disease–related events; diabetes, defined as fasting glucose ≥ 6.1 mmol/L or receiving antidiabetic medication; and hypertension, defined as resting systolic blood pressure ≥ 140 mm Hg or diastolic blood pressure ≥ 90 mm Hg on multiple measurements. A comprehensive echocardiographic evaluation was performed annually and included assessment of valvular function and measurement of aorta and pulmonary artery diameters. In addition, function of the left and right ventricles was evaluated using conventional echocardiographic measures and novel speckle tracking–derived strain and strain rate imaging.
First, the baseline strain and strain rate values of the patients with Marfan syndrome (derived from the first echocardiogram) were compared with the strain and strain rate values of a normal control population. This population comprised consecutive patients with no histories of medical illness and was matched for age, sex, and body surface area. All patients were referred for echocardiography with atypical chest pain, palpitations, or syncope without murmur and did not show structural heart disease. Those individuals who showed LV dilatation, had known hypertension, or were referred for echocardiographic evaluation of known valvular disease, murmur, or heart failure were excluded.
In addition, to assess whether strain-derived ventricular performance was related to the occurrence of adverse events in patients with Marfan syndrome, all cardiovascular events were noted during follow-up between the echocardiograms. For that purpose, cardiovascular events were defined as symptomatic heart failure, (supra)ventricular arrhythmia, and elective or emergency proximal aorta surgery. An event was noted as symptomatic heart failure when patients had heart failure–related symptoms (e.g., dyspnea, orthopnea) that could not be attributed to any other disease or evidence of heart failure during clinical investigation (e.g., edema, cyanosis). Arrhythmias were documented when patients were symptomatic or when noted on electrocardiographic evaluation during regular follow-up. Patients were referred for proximal aorta surgery when the diameter at the sinus of Valsalva exceeded 5 cm, when the aorta growth exceeded 0.5 cm/y, or when a family history of aortic dissection at a diameter < 5 cm was present.
Finally, to analyze changes in ventricular performance over time, the baseline echocardiogram was compared with the last follow-up echocardiogram, which was obtained ≥2 years after the baseline evaluation.
Echocardiography
Echocardiographic imaging was performed in the left lateral decubitus position using a commercially available system (Vivid Seven; GE Vingmed Ultrasound AS, Horten, Norway). Images were acquired at a depth of 16 cm in the parasternal, apical, suprasternal, and subcostal views using a 3.5-MHz transducer. In all patients, standard M-mode and two-dimensional images were obtained during breath holds, and three consecutive beats were saved in regular cine loop format. In addition, color Doppler data were acquired in all views after optimizing gain and Nyquist limit. Subsequently, standard continuous and pulsed-wave Doppler examinations were performed. The images were analyzed offline by two independent observers, using dedicated software for echocardiographic analysis (EchoPAC version 108.1.5; GE Vingmed Ultrasound AS).
Vascular Diameters and Valvular Function
The diameters of the aorta were measured in the parasternal long-axis view to visualize both the aortic root as well as the proximal part of the ascending aorta. Diameters were measured leading edge to leading edge at the level of the aortic annulus, the sinus of Valsalva, the sinotubular junction, and the ascending aorta. The pulmonary artery diameter was assessed in the parasternal short-axis view at the level of the aortic valve, and the diameter was measured in the proximal pulmonary artery, distal from the pulmonary valve.
Using color, continuous, and pulsed-wave Doppler data, valvular heart disease (regurgitation and stenosis) was scored semiquantitatively according to the guidelines of the American Society of Echocardiography and was graded as absent, mild, moderate, or severe. Evaluation of valvular stenosis included a qualitative assessment of morphology and motion of the valvular leaflets and quantification of transvalvular gradients and effective orifice area on the basis of continuous and pulsed-wave Doppler recordings. Valvular regurgitation was evaluated using an integrative approach that includes measurement of vena contracta of the regurgitant jet, quantification of the regurgitant volume and effective regurgitant orifice area using the proximal isovelocity surface area method whenever possible, and evaluation of atrial and ventricular dimensions. When tricuspid regurgitation was present, pulmonary artery pressure was estimated using the modified Bernoulli equation. Significant (clinically relevant) valvular heart disease was defined as moderate or severe valvular stenosis or regurgitation.
Conventional Measurement of Ventricular Function
First, conventional quantification of LV and RV systolic function was performed. For this purpose, LV end-diastolic volume (EDV), end-systolic volume, and ejection fraction were derived using the biplane Simpson’s method. LV volumes were indexed for body surface area according to Mosteller’s formula. In addition, diastolic function of the left ventricle was assessed using pulsed-wave Doppler of the mitral valve inflow. The Doppler sample volume was placed between the tips of the mitral leaflets, and subsequently, the peak early (E) and late (A) diastolic velocities and E-wave deceleration time were measured. Furthermore, the averaged E′ of the LV septal and lateral wall was measured using color-coded Doppler tissue imaging, and the E/E′ ratio was calculated. Thereafter, RV function was evaluated. First, RV diameters were measured in the apical four-chamber view at the level of the tricuspid annulus, mid right ventricle, and from base to apex. Second, RV fractional area change was determined using the standard formula. Third, tricuspid annular plane systolic excursion was measured in the RV free wall by placing the M-mode cursor in the lateral part of the tricuspid annulus parallel to the RV free wall, and the maximum systolic displacement of the RV base was measured.
Measurement of Ventricular Strain and Strain Rate
In addition to the conventional measures of ventricular performance, peak strain and strain rate of both the left and right ventricles were assessed offline, using speckle-tracking analysis software (EchoPAC version 108.1.5). For the current study, the peak longitudinal strain and strain rate values were calculated for the left and right ventricles separately. Maximum LV longitudinal strain and strain rate were calculated globally as the average of 18 segments, which were obtained in the apical two-chamber, three-chamber, and four-chamber views. In addition, strain and strain rate were measured circumferentially and radially on short-axis images of the left ventricle at the level of the papillary muscles. Furthermore, peak RV longitudinal strain and strain rate were determined as the average of three free wall segments obtained in the apical four-chamber view.
Statistical Analysis
SPSS version 16.0 for Windows (SPSS, Inc., Chicago, IL) was used for statistical analysis. Data are presented as mean ± SD, unless otherwise stated. Intraobserver reproducibility was determined by repeating the strain and strain rate measurements by a single observer in 25 randomly selected patients. The patients were again analyzed by the second observer to provide interobserver variability. Variability was determined using Bland-Altman analysis. Unpaired Student’s t tests were used to test the statistical significance of the differences between patients with Marfan syndrome and healthy controls. Cox proportional-hazards regression analysis was applied to analyze the association between strain and strain rate, on one hand, and the incidence of adverse events during follow-up on the other hand. In these analyses, strain and strain rate were entered as continuous variables into the regression equation. To test whether changes in vascular dimensions or ventricular function were statistically significant, a paired Student’s t test was performed. To analyze further whether the extent of potential changes in ventricular function was related to age, the study group was divided according to the mean age at baseline, corresponding to 35 years. Thereafter, changes in ventricular function were analyzed separately in the group of patients aged < 35 years and the group of patients aged ≥ 35 years at baseline. The difference in changes in ventricular function between the groups was assessed using an unpaired Student’s t test. P values < .05 were considered statistically significant.
Results
After the exclusion of six patients with significant valvular disease, five patients with other medical conditions besides Marfan syndrome, and 10 patients with inadequate follow-up duration (<2 years), 50 patients were included in the analysis. Characteristics of those patients are summarized in Table 1 . Exactly 50% of the patient population was male, and all patients were adults at time of inclusion. Marfan-related genetic mutations were found in 37 patients (74%). Pathologic mutations in the fibrillin-1 gene were identified in 35 patients (70%), whereas in two patients (4%), pathologic mutations in transforming growth factor–2 receptor were found.
Variable | Value |
---|---|
Male/female | 25/25 |
Genetic mutation | 37 (74%) |
Fibrillin-1 | 35 (70%) |
Transforming growth factor–2 receptor | 2 (4%) |
Cardioprotective medications ( n = 46) | 96% |
β-blockers | 25 (50%) |
ARBs | 8 (16%) |
ACE inhibitors | 2 (4%) |
Combination | 11 (22%) |
Baseline echocardiography | |
Age (y) | 35.2 ± 12.9 |
BSA (m 2 ) | 2.0 ± 0.3 |
Follow-up echocardiography | |
Age (y) | 39.0 ± 13.1 |
BSA (m 2 ) | 2.0 ± 0.2 |
Follow-up time (y) | 3.9 ± 1.2 |
Most patients used β-blockers, angiotensin-converting enzyme inhibitors, or angiotensin receptor blockers, though combinations were also frequently applied (22%; see Table 1 ). The mean age during the baseline echocardiographic evaluation was 35.2 ± 12.9 years, and the median follow-up duration was 4 years.
The mean age of the control group was 34.8 ± 8.1 years, comparable with that of the Marfan population ( P = .75). Body surface area, which was 1.94 ± 0.18 m 2 in the control population, did not differ significantly from that of the Marfan patient population ( P = .23). In addition, the number of male subjects was not statistically different in the control population ( n = 29 [58%]) compared with the Marfan population ( P = .55).
Intraobserver and interobserver agreement for biventricular strain and strain rate assessment was good. The intraobserver variability of LV longitudinal strain and strain rate was 0.19 ± 2.11% and 0.01 ± 0.26%/sec, respectively. The intraobserver variability of RV longitudinal strain and strain rate was 0.41 ± 1.45% and 0.03 ± 0.37%/sec. The interobserver variability of LV longitudinal strain and strain rate was 0.40 ± 2.95% and 0.02 ± 0.21%/sec, whereas the interobserver variability of RV longitudinal strain and strain rate was 0.30 ± 1.82% and 0.01 ± 0.38 %/sec, respectively.
Ventricular Strain and Strain Rate: Comparison between Patients with Marfan Syndrome and Healthy Controls
Strain and strain rate values, in addition to standard echocardiographic measures (see Supplemental Table 1 ), of 50 healthy control subjects were compared with the strain and strain rate values of the 50 patients with Marfan syndrome ( Figure 1 ).

Consistent differences in longitudinal strain and strain rate values of the left and right ventricles were observed between patients with Marfan syndrome and healthy controls. Mean peak LV longitudinal strain was −20.1 ± 1.9% and strain rate was −1.07 ± 0.18%/sec in normal controls, whereas these values were −18.9 ± 2.3 % ( P < .01) and −0.99 ± 0.19%/sec ( P = .03), respectively, in the Marfan population ( Figure 1 ). Similar differences in mean peak RV longitudinal strain (−29.3 ± 4.25% vs −26.9 ± 5.2%, P = .01) and strain rate (−1.65 ± 0.30%/sec vs −1.48 ± 0.31%/sec, P < .01) were observed between normal controls and patients with Marfan syndrome (see Figure 1 ). The mean peak LV circumferential strain rate was significantly lower in patients with Marfan syndrome (−1.13 ± 0.24%/sec) compared with the control population (−1.29 ± 0.27%/sec) ( P < .01), whereas mean peak LV circumferential strain was not significantly different. Finally, mean peak LV radial strain and strain rate were not significantly different between the groups.
Adverse Cardiovascular Events and Association with Baseline Strain and Strain Rate
During a mean follow-up duration of 3.9 ± 1.2 years of the Marfan population, seven cardiovascular events occurred. No episodes of symptomatic heart failure were observed. Arrhythmias occurred in three patients (6%) and consisted of atrioventricular nodal reentrant tachycardia ( n = 2) and atrial fibrillation ( n = 1). Proximal aorta surgery was performed in four patients (8%), because of either aneurysmatic widening of the proximal aorta ( n = 2) or type A aortic dissection ( n = 2). Univariate Cox regression analysis revealed that none of the baseline strain or strain rate values was related to the incidence of adverse events (see Table 2 ).
Variable | HR | 95% CI | P |
---|---|---|---|
Strain | |||
LV longitudinal | 1.08 | 0.74–1.58 | .68 |
LV radial | 0.96 | 0.88–1.03 | .26 |
LV circumferential | 1.01 | 0.81–1.24 | .96 |
RV longitudinal | 0.94 | 0.79–1.10 | .43 |
Strain rate | |||
LV longitudinal | 12.96 | 0.25–683.01 | .21 |
LV radial | 1.05 | 0.24–4.60 | .95 |
LV circumferential | 1.13 | 0.35–36.27 | .95 |
RV longitudinal | 0.46 | 0.04–5.50 | .54 |
Changes in Vascular Diameters and Conventional Measures of Ventricular Function
Overall, a significant increase in vascular diameters was consistently observed during follow-up, as summarized in Table 3 . A small increase in diameter was observed in the aortic valve annulus, the sinus of Valsalva (including the Z -score), and sinotubular junction. In addition, moderate widening was also noted in the ascending aorta and the trunk of the pulmonary artery. In contrast with vascular diameters, LV volumes and function remained unchanged during follow-up of the study population, as summarized in Table 2 . At baseline, mean LV EDV was mildly enlarged, but after correction for body surface area, mean LV EDV was within normal limits. The mean LV EDV was 64 ± 19 mL/m 2 at baseline and 64 ± 12 mL/m 2 at follow-up, indicating that LV EDV was not subject to changes. Analogously, no relevant changes in LV end-systolic volume and LV ejection fraction were observed. The mean LV end-systolic volume was 24 mL/m 2 , and the mean LV ejection fraction was 63% at both baseline and follow-up. In addition to systolic LV functional parameters, no changes in the measures of LV diastolic function or left atrial diameter were noted during follow-up ( Table 3 ).
Variable | Baseline | Follow-up | Mean change | P |
---|---|---|---|---|
Vascular diameters (cm) | ||||
Annulus (cm) | 2.5 ± 0.3 | 2.6 ± 0.3 | 0.1 ± 0.3 | .01 |
Sinus of Valsalva | 3.8 ± 0.5 | 4.0 ± 0.5 | 0.2 ± 0.3 | <.01 |
Z -score | 2.5 (−1.1 to 9.8) | 3.2 (−1.4 to 10.2) | 0.76 (−1.2 to 4.2) | <.01 |
Sinotubular junction | 3.1 ± 0.4 | 3.3 ± 0.4 | 0.2 ± 0.3 | <.01 |
Ascending aorta | 3.1 ± 0.4 | 3.4 ± 0.5 | 0.3 ± 0.4 | <.01 |
Pulmonary artery | 2.4 ± 0.4 | 2.6 ± 0.4 | 0.2 ± 0.3 | <.01 |
LV systolic function | ||||
LV EDV (mL) | 128 ± 36 | 133 ± 29 | 5 ± 24 | .19 |
LV EDV index (mL/m 2 ) | 64 ± 19 | 64 ± 12 | 0 ± 20 | .89 |
LV ESV (mL) | 48 ± 18 | 49 ± 17 | 1 ± 14 | .49 |
LV ESV index (mL/m 2 ) | 24 ± 9 | 24 ± 7 | 0 ± 9 | .73 |
LV EF (%) | 63 ± 6 | 63 ± 8 | 0 ± 7 | .67 |
LV diastolic function | ||||
E (m/sec) | 0.73 ± 0.17 | 0.73 ± 0.14 | −0.01 ± 0.15 | .77 |
A (m/sec) | 0.54 ± 0.12 | 0.56 ± 0.16 | 0.02 ± 0.13 | .26 |
E/A ratio | 1.41 ± 0.41 | 1.37 ± 0.35 | −0.04 ± 0.43 | .43 |
E′ (m/sec) | 0.09 ± 0.02 | 0.08 ± 0.02 | 0.00 ± 0.02 | .20 |
E/E′ ratio | 8.56 ± 2.03 | 9.01 ± 2.74 | 0.45 ± 1.87 | .11 |
LA dimensions | ||||
LA diameter (cm) | 3.6 ± 0.6 | 3.6 ± 0.7 | 0.0 ± 0.6 | .40 |
LA diameter index (cm/m 2 ) | 1.6 ± 0.7 | 1.7 ± 0.7 | 0.1 ± 0.8 | .37 |
RV systolic function | ||||
RVD1 (cm) | 3.4 ± 0.6 | 3.5 ± 0.5 | 0.1 ± 0.6 | .26 |
RVD2 (cm) | 3.5 ± 0.6 | 3.6 ± 0.7 | 0.2 ± 0.6 | .24 |
RVD3 (cm) | 7.4 ± 0.9 | 7.5 ± 1.0 | 0.1 ± 1.0 | .63 |
RVFAC (%) | 39 ± 7 | 39 ± 7 | 1 ± 9 | .62 |
TAPSE (cm) | 2.1 ± 0.5 | 2.1 ± 0.5 | 0 ± 0.4 | .62 |
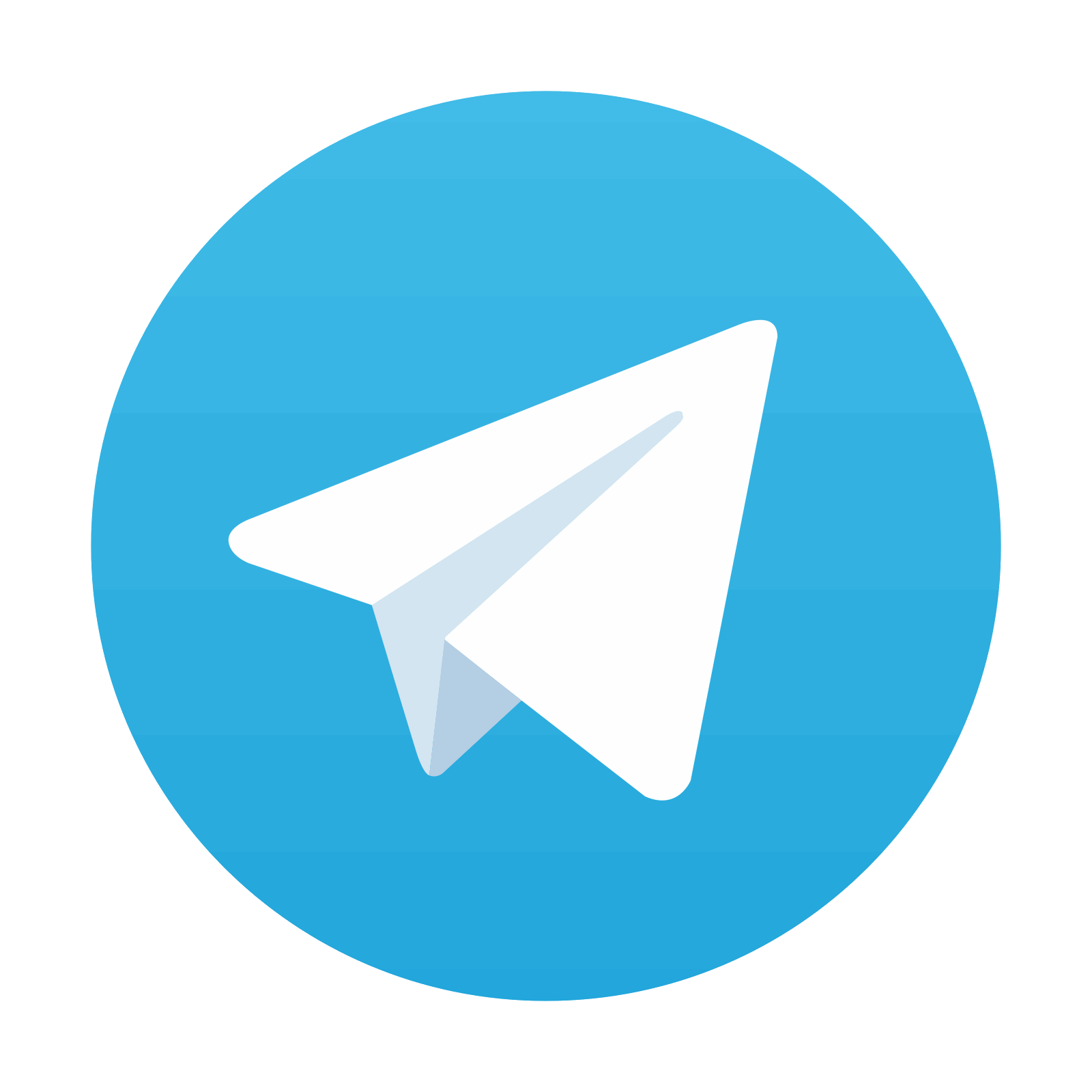
Stay updated, free articles. Join our Telegram channel

Full access? Get Clinical Tree
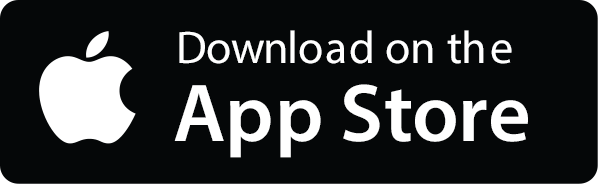
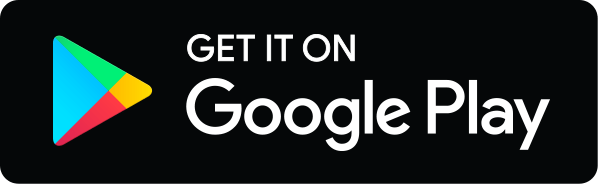
