2 Biomechanics of the Thoracic Spinal Column Abstract The thoracic spine is a unique load-bearing structure. It is an area of the spine that is often subject to extraphysiological forces during high energy trauma, and is often involved in spinal deformity such as scoliosis and kyphosis. The thoracic spine has unique biomechanical properties compared to other parts of the spine due to the articulations with the rib cage, the kyphotic sagittal alignment, and the transition from the stiff upper thoracic spine to the relatively mobile thoracolumbar junction. This unique anatomy comprised of articulations with the rib cage, and its overall sagittal alignment creates a distinct biomechanical environment which differs from the cervical and lumbar spine. Keywords: thoracic, ribs, kyphosis, fourth column, costovertebral Clinical Pearls • The thoracic spine has unique biomechanical properties compared to other parts of the spine due to the articulations with the rib cage through the costovertebral and costotransverse joints. • A transition zone exists between the relatively stiff upper thoracic spine and the more mobile thoracolumbar junction, creating a common area for traumatic disorders. • Stress is defined as force applied over unit area, typically expressed as Newtons/meters2. • Strain is a measure of relative deformation as a result of a force, measured as change in length over original length. • The functional spinal unit is defined as the smallest functional motion segment of the spine, and involves two adjacent vertebrae and the interposed soft tissues. • Physiological spinal range of motion demonstrates biphasic nonlinear load displacement behavior, characterized by a neutral zone and an elastic zone. The thoracic spine is a unique load-bearing structure in the human body. On a daily basis, it resists repeated physiological forces of axial loading, flexion and extension, lateral bending, and twisting while protecting the spinal cord and providing a foundation to the thorax. It is an area of the spine that is often subject to extraphysiological forces during high-energy trauma, and is often involved in spinal deformity such as scoliosis and kyphosis. The thoracic spine has unique biomechanical properties compared to other parts of the spine due to the articulations with the rib cage, the kyphotic sagittal alignment, and the transition from the stiff upper thoracic spine to the relatively mobile thoracolumbar junction. Thorough understanding of these biomechanical properties and the underlying anatomic structures is critical for spine surgeons who treat traumatic disorders, deformity, and degenerative conditions of the thoracic spine. A better understanding of the motion characteristics and forces transmitted through the thoracic spine will allow surgeons to elucidate the limitations of the system and the conditions that subsequently result in pain and tissue damage. Spinal biomechanics builds on the basic definitions and principles of orthopaedic biomechanics and applies them to the complexity of the spinal column. It provides a quantitative tool for assessment of the forces, movements, and overall function of the spine under varying loading conditions. The bulk of biomechanical information that we have on the thoracic spine has come from in vitro studies because of the difficulty in obtaining these measures in vivo. Though biomechanical models can be utilized to predict response interactions to loads on spinal tissues of interest, these responses represent a gross estimation and likely differ from their natural occurrence in live humans. Stress is defined as force applied over unit area, typically expressed as Newtons/meters2. These forces can be compressive, tensile, shear, or torsional.1 Strain is a measure of relative deformation as a result of a force, measured as change in length over original length.1 When this deformation is temporary, it is termed elastic deformation, and when it is permanent, it is termed plastic deformation. Every material has a unique stress–strain curve which describes how that material will respond to an external stress. The stress–strain curve typically starts with an elastic zone, where the stress and strain are proportional, and described by Young’s modulus (E). At the end of this elastic point is the yield point; further deformation to the material is irreversible, until the breaking point is reached, which is the point at which the material fails. In the realm of spinal biomechanics, the functional spinal unit (FSU) is defined as the smallest functional motion segment of the spine, and involves two adjacent vertebrae and the interposed soft tissues.2 Each FSU can translate and/or rotate about three orthogonal axes, creating 12 possible modes of displacement. When these individual motions are combined, they result in more complex motions of flexion/extension, lateral bending, and axial rotation. The instantaneous axis of rotation (IAR) is the imaginary line that the vertebral body will rotate about when a bending moment arm is applied to the FSU. In the thoracic spine this typically occurs in the superior end plate of the more caudal vertebral body of the two adjacent vertebrae.3 The neutral axis is the line connecting the IARs in the spinal column.2 Spinal movements are referenced on a coordinate system where rotation is described in the axial or transverse plane, lateral bending in the coronal plane, and flexion/extension in the sagittal plane. Physiological spinal range of motion (ROM) demonstrates biphasic nonlinear load displacement behavior, characterized by a neutral zone and an elastic zone. The neutral zone is the range of intervertebral motion within which physiological spinal motion is produced with minimal internal resistance. Panjabi described this as the zone of high flexibility or laxity.4 The elastic zone is defined as the range of intervertebral motion from the end of the neutral zone to the physiological limit of motion, and is a result of internal resistance from various soft issues structures and musculature.2,5 This is the zone of high stiffness.4 The neutral zone represents an important functional measurement of spine stability and can be affected by muscular weakness, trauma, degeneration, and instrumentation. Muscle strengthening, osteophyte formation, and surgical fixation/fusion work to decrease the neutral zone to within a normal physiological range.4 The thoracic spine is the largest segment of the spinal column and is composed of 12 vertebrae. Although the basic structure of the vertebral body is the same, the thoracic spine is distinguished from the cervical and lumbar spine by its articulations with the ribs through the costovertebral and costotransverse joints, which contribute to the stability of the upper thoracic spine. As a result, a transition zone exists between the relatively stiff upper thoracic spine and the more mobile thoracolumbar junction, creating a common area for traumatic disorders. The thoracic spine differs from the cervical and lumbar spine in that it articulates with the ribs through the costovertebral and costotransverse joints, and thus is ultimately stabilized by the rib cage.6 These articulations occur from the 1st to 10th thoracic vertebrae. The costovertebral articulation occurs at the level of the thoracic disc, and is comprised of two demifacets above and below the disc bilaterally. Additionally, the ribs articulate with a concave facet on the ventral surface of the transverse process termed the costotransverse joint or costal facet of the transverse process. The 11th and 12th ribs are disconnected from the sternum and are often labeled as “floating ribs.” Their respective thoracic vertebrae have unique transitional characteristics with small transverse processes that fail to buttress the last two ribs, likely contributing very little stability to that region of the spine.7,8 Cadaveric sequential sectioning studies have shown the ribs add stiffness to rotational and bending forces to the thoracic spine.9 When the rib head is resected, ROM is increased from 70 to 80% in flexion, extension, and bending.9 Watkins et al demonstrated that the rib cage increased the stability of the thoracic spine by 40% in flexion/extension, 35% in lateral bending, and 31% in axial rotation.10 Brasiliense et al, in a cadaveric study, demonstrated that the sternum and anterior rib cage contributed most to stability in flexion/extension, the posterior rib cage was most important in lateral bending, and stability in axial rotation was directly related to the proportion of rib that remained intact.8 Overall, they found that the ribs accounted for 78% of mechanical thoracic stability. Given the increased stability that the rib cage and sternum confer to the thoracic spine, it has been proposed that the rib cage and sternum be considered a “fourth column” in the classic three-column model of spinal stability.11
2.1 Overview
2.2 Biomechanics
2.2.1 Definitions
2.2.2 Neutral and Elastic Zones
2.3 Anatomy
2.3.1 Ribs and Sternum
2.3.2 Vertebral Body
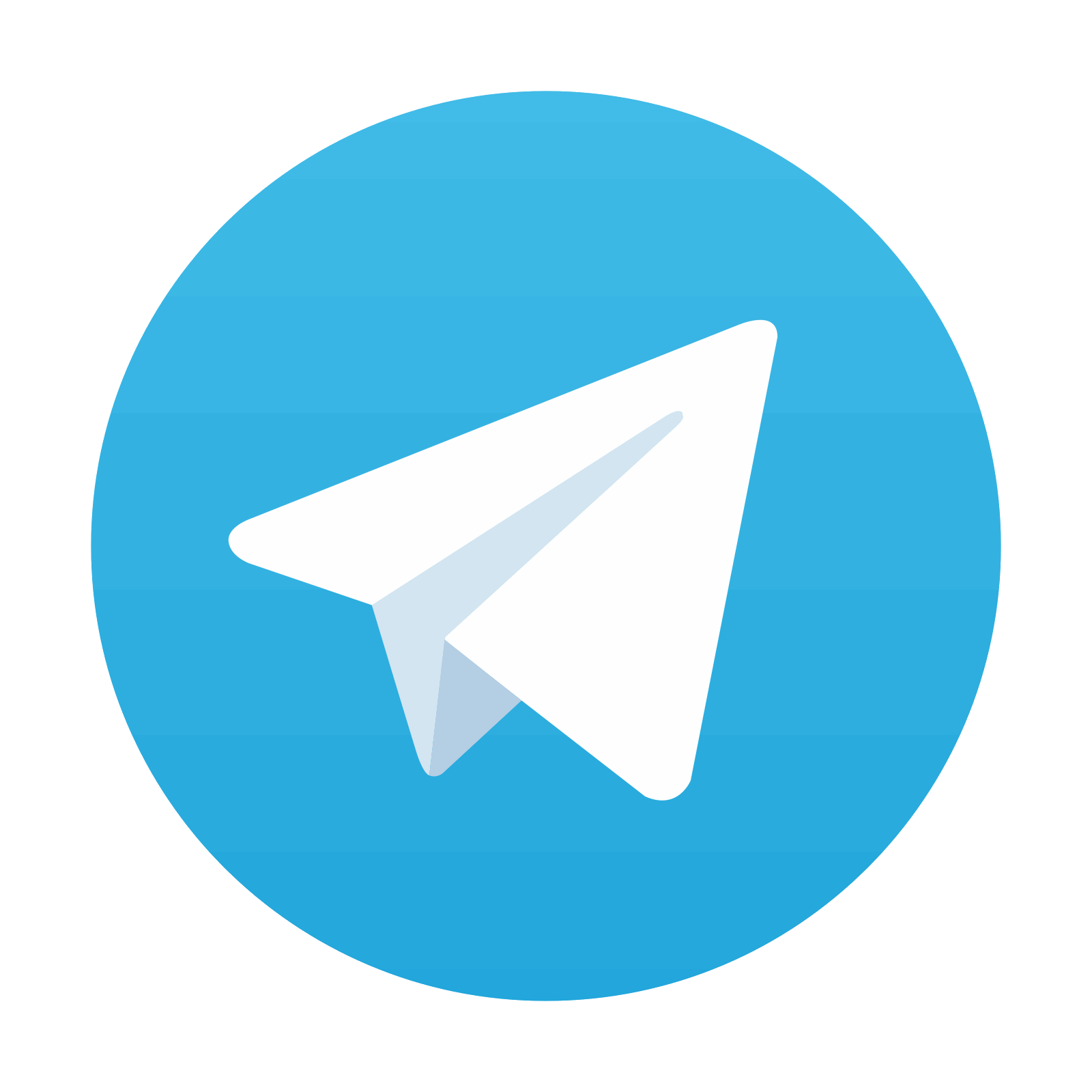
Stay updated, free articles. Join our Telegram channel

Full access? Get Clinical Tree
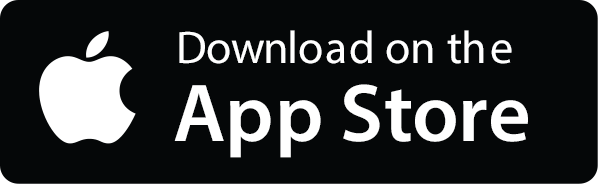
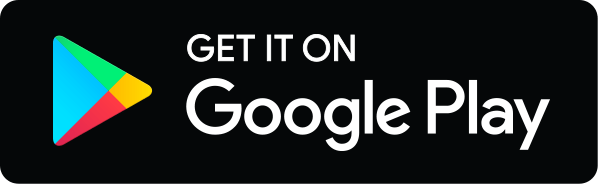