A 35-year-old man arrives by ambulance to the emergency department (ED) with chief complaints of chest pain, shortness of breath, nausea, and dizziness. Physician interviews reveal no relevant risk factors for cardiovascular disease and no family history of cardiovascular events. Because his electrocardiogram (ECG) does not show any alterations, and the chest x-ray is normal, he is promptly discharged with possible diagnoses of influenza or stress. A few days later, he returns to the ED for severe chest pain accompanied again by shortness of breath and dizziness. Both ECG and chest x-ray do not show relevant alterations, so he is again discharged. Less than 30 hours later, he is found on his kitchen floor, unresponsive. First responders declare him dead at 3:30 that morning. The following day, his autopsy reveals the cause of death: aortic dissection.
Biomarkers have risen to popularity for their ability to promote timely diagnoses, reveal prognostic information, monitor disease progression, and predict therapeutic response. In the context of emergency medicine, biomarkers can serve as rule-in or rule-out diagnostic tools to supplement the emergency physician’s knowledge and judgment. In the Patient Case above, laboratory tests may have served both secondary and tertiary prevention roles in the optimal course of treatment. In the context of the continuous management of a chronic disease, such as heart failure (HF), biomarkers can help evaluate whether therapies are effective, guide management, and predict adverse outcomes.1,2
In the ED, the most common and concerning symptoms are nontraumatic chest pain and shortness of breath, especially among older populations; they represent, respectively, 9% to 10% of ED complaints, followed by abdominal pain and general weakness. This equates to more than 1.5 million visits out of a total of more than 5.0 million visits a year in the United States alone, and this figure has steadily grown over the past decade. Often, patients experience concomitant chest pain and shortness of breath, but dizziness, nausea, and diaphoresis can commonly present as well.1
Cases with these symptoms share a wide variety of etiologies; the most concerning, ie, those that must be most promptly included or excluded, can either have cardiac or respiratory etiologies. Acute myocardial infarction (AMI), acute heart failure (AHF), aortic dissection, and pulmonary embolism (PE) are the most common diagnoses, but pneumothorax, asthma, or a panic attack can present with these symptoms. For this reason, accurate and specific diagnostic techniques are crucial for timely treatment. The patient’s history, clinical evaluation with an accurate physical examination, biomarkers, and some diagnostic tools—such as ECG, x-ray, and other imaging techniques—are fundamental in identifying the disease.2 The efficacy depends on biomarker predictive accuracy; based on the etiology of biomarker release, the marker may have high sensitivity, specificity, positive predictive value, or negative predictive value (Figure 20-1).
Figure 20-1
The 4 main accuracy values associated with a quantitative biomarker. Sensitivity and specificity evaluate the probability of correct testing status given disease state, while predictive values assess the patient’s likelihood of disease in the context of the associated positive or negative test result. While sensitivity and specificity are often the chief focus of biomarker evaluation and utility, positive—but mostly negative—predictive value presents a particular proclivity for clinical rule-out in the differential diagnosis, management, and prognosis of cardiac diseases.

Recent advances in biomarker detection have given the emergency medicine community cause to diagnose and treat persons with acute coronary syndrome (ACS) much more quickly, methodically, and quantitatively. The world of cardiac symptoms has become increasingly biochemical with the goal of improving diagnosis and prognosis, from the emergency setting to the chronic management of the patient.2 In this chapter, we outline the most common uses of cardiac biomarkers in the clinical setting and alert the reader to recent advances, particularly with regards to prognosis.
There is a strong association between the type of damage or alteration that characterizes a pathological condition and the mechanism of biomarker release. In this review, we outline the utility of several biomarkers in relation to both their biochemistry and associated dynamics in pathophysiology and their practical utility in the clinical setting.
Several biomarkers, the most important of which are cardiac peptides, show vastly increased sensitivity and specificity over canonical ECG changes and chest x-ray techniques.2-4 Hundreds of novel cardiac biomarkers, such as microRNAs (miRNAs), in conjunction with well-known biomarkers of more generalized necrosis, fibrosis, and systemic stress have also been proposed as biomarkers for both rule-in and rule-out for potential cardiac pathologies presenting with the traditional symptoms described above.
Essentially, a constellation of biomarkers must be considered in conjunction with the physician’s best judgment to differentially diagnose the etiologic pathology of these symptoms, especially as new markers are discovered for specific cellular and biochemical processes.2 Figure 20-2 shows just some of the signals that may or may not manifest clinically in cardiac pathophysiology. It behooves the physician to consider the multidimensionality of biomarker elevation; compartmentalization, pharmacological history, biochemistry, and metabolism of such markers all play a role when considering the clinical picture of the patient, particularly in differential diagnosis.
Figure 20-2
Clinical constellation of cardiac pathology etiologies and correspondent elevations in biomarkers. Elevated or suppressed biomarkers (red) must be clinically considered together with other disease inputs (green), and metabolic or pharmacologic clearance (purple) to arrive at an accurate clinical diagnosis, especially in the context of multiple comorbidities. Though certain markers will point positively to certain disease state etiologies (eg, procalcitonin and infection), negative predictive value should also be considered to rule out other etiologies, a valuable tool in the context of differential diagnosis.

To lend insight into the impetus behind the use of biomarkers in differential diagnosis of common cardiac diseases, we outline briefly some biochemical features of the most commonly used markers in clinical practice. Also we will mention some of the emerging biomarkers that are still under study but are promising as both diagnostic and emerging prognostic tools (Table 20-1).
Molecule | Characteristics | Normal Levels | Altered In |
---|---|---|---|
Myoglobin (Mb) |
| 0-85 ng/mL | AMI Timeline of detectability after the ischemic event
|
Creatine kinase-MB (CK-MB) |
| 5-25 IU/L 3%-5% (ratio CKMB/total CK) | AMI Timeline of detectability after the ischemic event
|
Lactate dehydrogenase (LDH) |
| 105-333 IU/L | AMI Timeline of detectability after the ischemic event
|
Troponin I (TnI) |
| 99th percentile | AMI Timeline of detectability after the ischemic event
|
High-sensitivity troponin I (hsTnI) |
| Assay dependent | As above |
MicroRNA (miRNA/miR) |
| Under investigation | AMI and HF |
Copeptin |
| Under investigation | AMI and HF |
Soluble ST2 (sST2) |
| Under investigation | AMI and HF |
Pro-N-terminus brain natriuretic peptide (NT-proBNP) |
| <300 pg/mL | HF (see Figure 20-3) |
Brain natriuretic peptide (BNP) |
| <100 pg/mL | HF (see Figure 20-3) |
D-dimer |
| <500 ng/mL | PE and aortic dissection |
Myoglobin (Mb) is a carrier of oxygen, ubiquitously present in both cardiac and skeletal muscle types. It serves as a reserve of aerobic fuel when hemoglobin reserves deplete or when myocytes undergo necrotic damage. Because it infiltrates the bloodstream in both skeletal and cardiac muscle damage, its specificity is low in the clinical setting for acute cardiac distress. Normal levels rest between 0 and 85 ng/mL.5
Creatine kinase-MB (CK-MB) is a heterodimer in a family of molecules that catalyzes the phosphorylation of creatine, a ubiquitous byproduct of amino acid catabolism. It is highly linked to transmitochondrial energy pathways and serves as a key regulator of ATP inside the mitochondrial lumen and in the cytosol. The MB isoform is present in myocytes’ cytosol, so it is strongly related to this type of cell damage. However, this marker has still proven less sensitive than other acute cardiac biomarkers. Normal levels rest between 5 and 25 IU/L, though it can also be reported as a ratio of isoforms. In this case, a 3% to 5% ratio of CK-MB to total CK blood content is typical in a healthy patient.6
Lactate dehydrogenase (LDH) is produced in response to increased metabolic stress in cardiomyocytes. Anaerobic metabolism produces lactate in the cytosol of diseased cardiomyocytes, necessitating the production of increased LDH. The enzyme is released from the cytosol when cardiomyocytes undergo necrosis, particularly in AMI. Normal levels of LDH fall between 105 and 133 IU/L, though this can vary slightly and should always be adjusted relative to a cohort of healthy controls for individual assay kits.7
Troponin I (TnI) is 1 of the 3 troponin isoforms, and it inhibits the ATPase activity of myosin complexes. The peptide binds to actin in thin myofilaments to hold the troponin-tropomyosin complex in place. Primarily residing in the cytosol of cardiac myocytes, TnI comprises 1 of the most localized detectable known cardiac biomarkers, yielding high sensitivity and specificity. Because assays vary, typical TnI levels are determined based on individual assay comparison to a cohort of healthy control patients. A TnI reading above the 99th percentile in a control cohort of healthy patients indicates significant cardiac myocyte necrosis.
More sensitive assays have recently emerged to detect extremely low levels of circulating TnI even in healthy patients. In these so-called hsTnI assays, levels below 0.01 ng/mL are considered normal (a typical cutoff for normal TnI assays is 0.07 ng/mL).8
ST2 is a member of the family of interleukin 1, and its ligand is interleukin 33 (IL33). It has 2 isoforms: 1 soluble (sST2), detectable in serum; and 1 membrane-bound (ST2L). Both have different roles related to cardiac pathology. In the case of cardiac injury, ST2L binds IL33, and it exerts a preservative effect on heart function. In fact, IL33 has antihypertrophic and antifibrotic effects on myocardium, in part due to reduction in proapoptotic pathways. However, because IL33 also binds to sST2, when higher serum levels of sST2 are present, there is increased competition between the 2 ST2 isoforms; thus, the cardioprotective effect is decreased by increased sST2 plasma levels.9
The prohormone pre-proBNP is secreted from the myocardium, whereupon it is processed into the active hormone BNP-32 and the inactive amino-terminal fragment NT-proBNP-76. The active part of this lysis, BNP, exerts hormonal effects on the heart and kidneys, decreasing sympathetic tone, reducing afterload by inducing the secretion of sodium and water into the urine, and inhibiting RAAS mechanisms. It is released into the bloodstream in response to increased ventricular load, and is broken down into inactive fragments by the enzyme neprilysin. Normal levels in healthy patients vary, but cutoff schemes pivoting about 100 pg/mL are usually employed (see the upcoming section, Diagnosis: “Heart Failure”).
Released in equimolar amounts to BNP, NT-proBNP is an inactive fragment byproduct of pre-proBNP lysis that is not broken down by neprilysin. Increased ventricular wall stress promotes the synthesis of BNP, releasing NT-proBNP into the bloodstream as a result. The sensitivity of this marker has been debated and is still under investigation with respect to diagnostic ability.10
D-dimer is a thrombus degradation byproduct that circulates in the blood in response to thrombotic events such as emboli. It is notably increased in events such as aortic dissection, though it is not specific to these conditions; any thrombotic event will result in increased levels of this fibrin-derived homodimer, so it can be considered highly sensitive, but not specific. A normal D-dimer value is typically under 500 ng/dL in healthy populations.11
The insufficiency in the heart’s ability to pump blood in response to the body’s needs is a serious condition with high mortality, morbidity, and frequent visits to the ED and/or multiple rehospitalizations. This condition represents a major cause of hospitalization in people >65 years old, and the incidence of new cases has been predicted at >550,000 cases/year, adding to some 5.8 million prevalent cases in the United States alone.1,12 HF usually has a typical presentation: dyspnea, low extremities edema, fatigue, orthopnea and paroxysmal nocturnal dyspnea or episodes of obstructive sleep apnea, and weight gain not related to diet. Detectable signs can be distinguished in these typical of left- or right-sided HF, but usually there is the copresence of both dysfunctions.2-4,12 When one suspects HF, BNP and NT-proBNP levels are usually examined.
In the Breathing Not Properly trials, BNP levels were shown to vary in diagnostic power with age but not sex or race. Now a standard for cardiac health care, BNP and NT-proBNP tests bring both high sensitivity and negative predictive value to the differential diagnosis of HF in the acute setting.13 One proposed scheme includes a stratification structure for likelihood of HF in the context of symptoms of acute cardiac decompensation. We particularly highlight the gray zone of 100 to 400 pg/mL (Figure 20-3). In the presence of chest pain or shortness of breath, we stress the importance of clinical judgment including patient history, risk factors, and physical examination in diagnosing HF when natriuretic peptides fall in this range.14
Figure 20-3
BNP and NT-proBNP stratification schemes in the context of heart failure differential diagnosis. While these figures are often used in conjunction with imaging data to diagnose heart failure, these ranges may change with age and weight, as discussed in the text. Despite the range of values, both BNP and NT-proBNP have high sensitivity and specificity for heart failure in the context of volume overload, and larger increases should correlate with likelihood of heart failure in the appropriate age ranges.

In contrast, the predictive accuracy of NT-proBNP has been shown to interact with age, likely due to naturally progressive decreases in left ventricular compliance and glomerular filtration rate (GFR). Figure 20-3 highlights the lower cutoff of 300 ng/mL for likelihood of HF diagnosis in the appropriate clinical presence. After age 50 years, the likelihood of HF increases with age at the rate of about 2 times the lower NT-proBNP cutoff per 25 years thereafter. NT-proBNP is not as widely utilized as BNP in the clinical setting, but studies continue to emerge with the promise of increasing its predictive power.
Particular attention has to be given to patients with elevated body mass index (BMI). In fact, an inverse relationship between BNP and NT-proBNP levels and BMI has been found. Obesity is a risk factor for systemic hypertension, hyperlipidemia, diabetes mellitus, and left ventricular hypertrophy. These conditions, in turn, are associated with an increased prevalence of chronic heart failure (CHF) and an increased mortality rate of about half of the patient population within 5 years of diagnosis. Obese patients can present with common symptoms and signs of HF, such as low extremities edema, dyspnea, and orthopnea; also, diagnostic tools such as chest x-rays and cardiac echocardiograms can be difficult to perform or to interpret due to body habitus.
Biomarkers can significantly aid in the management of HF patients in the emergency setting. As already mentioned, higher levels of natriuretic peptides in the bloodstream in patients with dyspnea are likely related to HF, but obese patients unfortunately could possibly not present with elevated natriuretic peptides. In 2004, Wang et al were the first to describe the inverse relationship between obesity and BNP levels and none of the subjects had HF. The mean plasma BNP levels were 21.4, 15.5, and 12.7 pg/mL in patients who were lean (BMI <25), overweight (BMI of 25-29.9), and obese (BMI ≥ 30), respectively. Subsequently, the Dallas Heart Study confirmed the report of Wang et al and demonstrated the inverse relationship among BMI, BNP, and NT-proBNP levels, both in stable and acute CHF patients.
According to these results, several studies had been completed in order to establish a punctual cutoff; lower thresholds were considered for diagnoses of the CHF patient population. In one study, based on the established clinical threshold of 100 pg/mL, BNP testing yielded false-negative results in 20% of obese HF patients, so, to rule out CHF, the cutoff point should be BNP ≤54 pg/mL in severely obese patients (BMI ≥35).15
It is also suggested that a higher BNP cutoff point of ≥170 pg/mL in lean patients increases specificity. In contrast to BNP cutoff points, relatively lower concentrations of NT-proBNP in overweight and obese patients with acute dyspnea retain their diagnostic and prognostic capacity. A cutoff point of 300 ng/L NT-proBNP had highly significant negative likelihood ratio of 0.02, 0.03, and 0.08 for BMI of <25.0, 25.0 to 29.9, and ≥30.0, respectively, ruling out acute HF. Also, a NT-proBNP cutoff point of >986 ng/L remained strongly prognostic across all 3 BMI groups.16
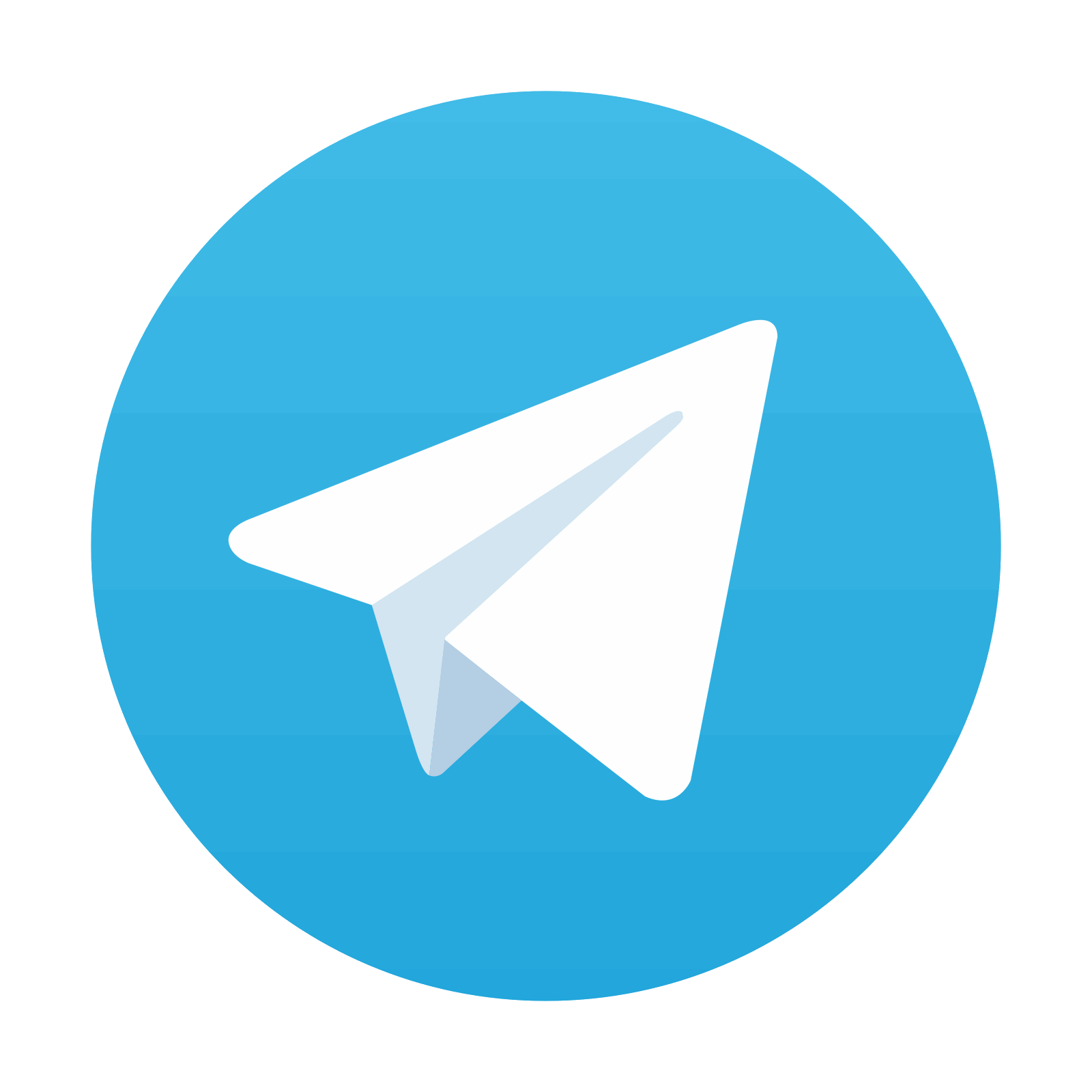
Stay updated, free articles. Join our Telegram channel

Full access? Get Clinical Tree
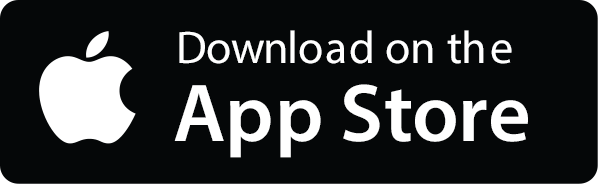
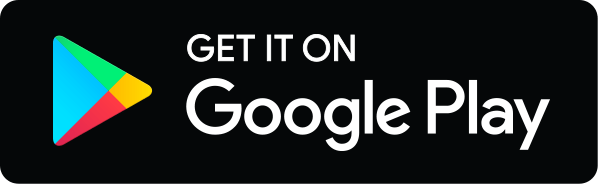