Conventional Doppler echocardiography, including pulsed-wave, continuous-wave, and color-flow Doppler techniques, is an integral component of an echocardiographic study for the diagnosis and assessment of cardiovascular disease. It is used routinely to provide hemodynamic information for flow measurement, diastolic function, estimation of right-sided pressures, pressure gradients and valve areas, valve regurgitation, shunt lesions, and prosthetic valves, to name a few, that would otherwise require invasive investigation.
Compared with other modalities, such as cardiac magnetic resonance and invasive measurements by cardiac catheterization, Doppler echocardiography has a few limitations. One of the “soft spots” is the lack of accuracy of quantitative flow measurement in assessing stroke volume (SV), cardiac output, shunt lesions, and regurgitant volumes and fractions, which limits not only the routine evaluation of various structural and flow abnormalities but also the assessment of medical, percutaneous, and surgical interventions and longitudinal follow-up.
Limitations of Flow Measurement by Doppler Echocardiography
Flow is derived as the product of cross-sectional area (CSA) and the average velocity of the blood flow through a blood vessel or valve orifice. Therefore, SV is usually calculated from the velocity-time integral (VTI) using pulsed-wave Doppler, typically through the aortic valve, mitral valve, or pulmonary valve, multiplied by the CSA by two-dimensional (2D) echocardiography. At least two assumptions must be made to derive flow by 2D and Doppler echocardiography: (1) The velocity profiles across the valve are flat and uniform; therefore, the sample volume placed at the center of the valve represents all velocities across the flow stream. (2) The geometry and area of the valve are constant during the cardiac cycle, and the CSA can be measured accurately by 2D echocardiography. In fact, neither of these two assumptions is true. For example, Haugen et al. showed that the mean of the ratio of the maximum VTI at the center of the valve to the mean VTI was 1.3 (range, 1.1–1.6) across the mitral valve using a reconstructive three-dimensional (3D) color Doppler technique. At the time of peak flow, the mean of the ratio of the maximum to the mean velocity was 1.5 (range, 1.2–2.6). When one uses a single sample volume for Doppler measurements of the maximum VTI, errors ranging from 10% to 60% may be introduced when calculating SVs. For the aortic valve, the errors ranged from 20% to 50%.
Accurate measurement of the CSA of the valve is also challenging. The diameter ( D ) of the valve annulus is routinely measured to calculate the CSA as D 2 × π/4. This calculation has been shown to be an oversimplification. For example, Levine et al. demonstrated that the mitral valve is far from regular and circular. As shown in the previous equation, any inaccuracy in the measurement of the valve diameter is multiplied in the calculation of SV. Furthermore, mitral valve diameter changes during the course of the cardiac cycle, and it has a complex, biphasic flow profile.
Three-Dimensional Doppler Echocardiography
As discussed above, 3D Doppler echocardiography is the next logical step toward the objective of improving the accuracy of flow measurement. The 3D Doppler echocardiography technique for quantifying volumetric flow is based on the Gaussian theorem: for any arbitrarily shaped control surface system, the flow rate passing through it equals the sum of all velocity components that are normal to the surface. The technique was first tested in 2D color Doppler flow mapping and reconstructive 3D color Doppler echocardiography.
My colleagues and I first became interested in volumetric flow measurement when real-time 3D color Doppler echocardiography (RT3DCDE) became available. We worked in collaboration with Dr. Milan Sonka’s laboratory in the Department of Electrical and Computer Engineering at the University of Iowa, supported by a research grant from the University of Iowa and the American Heart Association. We used a hemispheric surface model based on the Gaussian theorem and developed an algorithm to unwrap velocity aliasing by baseline shift. We first demonstrated by RT3DCDE that volumetric flow across the mitral valve orifice correlated and agreed well with SV determined by phase velocity cine magnetic resonance imaging at the ascending aorta and by left ventricular volumetric measurement by magnetic resonance imaging. In addition, compared with SV determined by real-time 3D echocardiography, the correlation with volume flow was excellent for the mitral valve ( r = 0.91), the aortic valve ( r = 0.89), and the pulmonary valve ( r = 0.89) but poor for the tricuspid valve ( r = 0.20) using RT3DCDE. Agreement was good for the aortic valve (bias, 0.9 ± 5.0 mL), the pulmonary valve (bias, −0.4 ± 5.7 mL), and the mitral valve (bias, 4.1 ± 4.7 mL) and markedly underestimated for the tricuspid valve (bias, −24.4 ± 14.6 mL). In vitro and in vivo animal and human studies have also validated this approach. Therefore, there is a growing body of evidence supporting the utility of RT3DCDE for measuring SV and other indices, but there is a lack of development of more automated methods and software to incorporate this approach into clinical practice.
In this issue of the Journal of the American Society of Echocardiography , Thavendiranathan et al. describe a “3D real-time volume color-flow Doppler” approach, developed by a major vendor, with several new features: (1) automated detection of the 3D left ventricular endocardial surface, the mitral annulus, and the left ventricular outflow tract; (2) automated adjustment of each frame, used to derive a flow curve when the hemispheric surface is applied to the valve orifices; (3) feasibility of a one-beat 3D data set; (4) temporal resolution of 33 volumes/sec and the acquired color Doppler voxel dimension at the sampling plane of 4.5 × 5.0 × 0.6 mm in the azimuthal, elevational, and range directions, respectively; and (5) a field of view of 90° × 90°, so that mitral and aortic flow can be incorporated into the same data set.
Using this approach, the investigators found that automated flow measurement was feasible in all study patients. Compared with flow measured by cardiac magnetic resonance, for both mitral and aortic valves, the 3D Doppler method derived mitral and aortic flows not statistically different from those measured using CMR. In contrast, 2D transthoracic echocardiographic SV underestimated the reference standard for the mitral and the aortic valves. The interobserver and intraobserver variability for SV measurements was significantly worse for the 2D compared to the 3D transthoracic echocardiographic method. Therefore, using 3D Doppler echocardiography represents a significant step toward accurate and reproducible automated flow measurement.
Three-Dimensional Doppler Echocardiography
As discussed above, 3D Doppler echocardiography is the next logical step toward the objective of improving the accuracy of flow measurement. The 3D Doppler echocardiography technique for quantifying volumetric flow is based on the Gaussian theorem: for any arbitrarily shaped control surface system, the flow rate passing through it equals the sum of all velocity components that are normal to the surface. The technique was first tested in 2D color Doppler flow mapping and reconstructive 3D color Doppler echocardiography.
My colleagues and I first became interested in volumetric flow measurement when real-time 3D color Doppler echocardiography (RT3DCDE) became available. We worked in collaboration with Dr. Milan Sonka’s laboratory in the Department of Electrical and Computer Engineering at the University of Iowa, supported by a research grant from the University of Iowa and the American Heart Association. We used a hemispheric surface model based on the Gaussian theorem and developed an algorithm to unwrap velocity aliasing by baseline shift. We first demonstrated by RT3DCDE that volumetric flow across the mitral valve orifice correlated and agreed well with SV determined by phase velocity cine magnetic resonance imaging at the ascending aorta and by left ventricular volumetric measurement by magnetic resonance imaging. In addition, compared with SV determined by real-time 3D echocardiography, the correlation with volume flow was excellent for the mitral valve ( r = 0.91), the aortic valve ( r = 0.89), and the pulmonary valve ( r = 0.89) but poor for the tricuspid valve ( r = 0.20) using RT3DCDE. Agreement was good for the aortic valve (bias, 0.9 ± 5.0 mL), the pulmonary valve (bias, −0.4 ± 5.7 mL), and the mitral valve (bias, 4.1 ± 4.7 mL) and markedly underestimated for the tricuspid valve (bias, −24.4 ± 14.6 mL). In vitro and in vivo animal and human studies have also validated this approach. Therefore, there is a growing body of evidence supporting the utility of RT3DCDE for measuring SV and other indices, but there is a lack of development of more automated methods and software to incorporate this approach into clinical practice.
In this issue of the Journal of the American Society of Echocardiography , Thavendiranathan et al. describe a “3D real-time volume color-flow Doppler” approach, developed by a major vendor, with several new features: (1) automated detection of the 3D left ventricular endocardial surface, the mitral annulus, and the left ventricular outflow tract; (2) automated adjustment of each frame, used to derive a flow curve when the hemispheric surface is applied to the valve orifices; (3) feasibility of a one-beat 3D data set; (4) temporal resolution of 33 volumes/sec and the acquired color Doppler voxel dimension at the sampling plane of 4.5 × 5.0 × 0.6 mm in the azimuthal, elevational, and range directions, respectively; and (5) a field of view of 90° × 90°, so that mitral and aortic flow can be incorporated into the same data set.
Using this approach, the investigators found that automated flow measurement was feasible in all study patients. Compared with flow measured by cardiac magnetic resonance, for both mitral and aortic valves, the 3D Doppler method derived mitral and aortic flows not statistically different from those measured using CMR. In contrast, 2D transthoracic echocardiographic SV underestimated the reference standard for the mitral and the aortic valves. The interobserver and intraobserver variability for SV measurements was significantly worse for the 2D compared to the 3D transthoracic echocardiographic method. Therefore, using 3D Doppler echocardiography represents a significant step toward accurate and reproducible automated flow measurement.
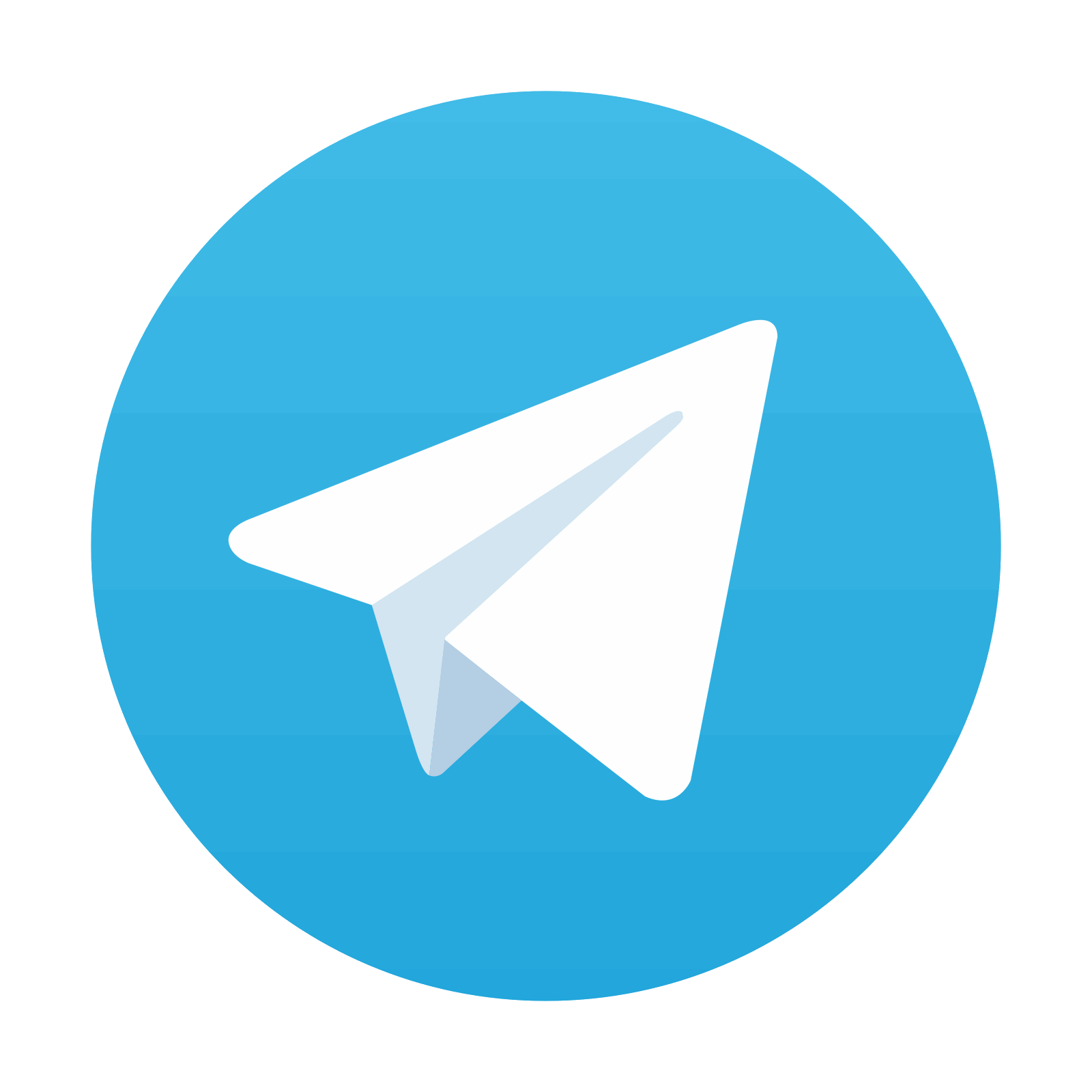
Stay updated, free articles. Join our Telegram channel

Full access? Get Clinical Tree
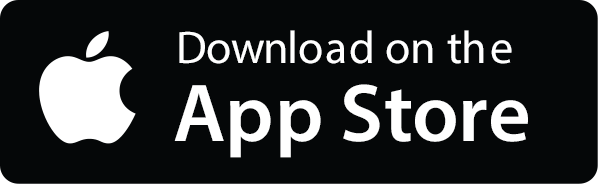
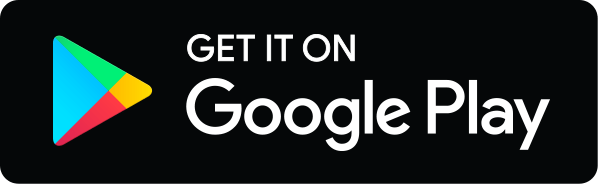