Fig. 21.1
Principles of autofluorescence: green fluorescence is emitted by normal bronchial tissue when excited by blue light, cancer shows decreased green fluorescence due to increased epithelial thickness and vascularity
Clinical studies (Table 21.1) using these devices have generally demonstrated improved sensitivity of AF (1.3–6.4 times) over WLB for the detection of preinvasive lesions, also in patients with abnormal sputum cytology. AF appears to be better at defining tumor margins and may add useful information in preoperative surgical planning as well as in targeting the area for endoscopic treatment (Fig. 21.2).
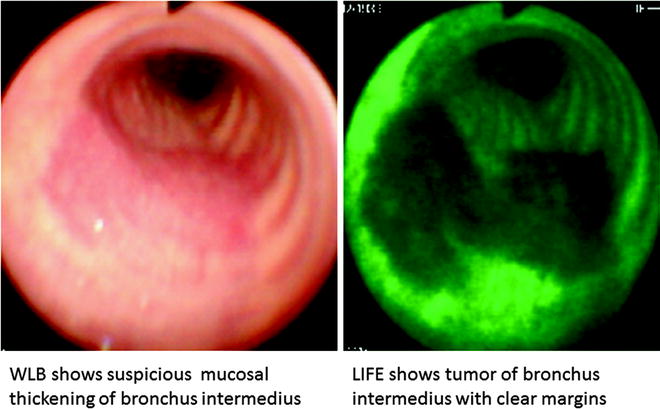
Table 21.1
Sensitivity and specificity of autofluorescence bronchoscopy for airway dysplasia
Authors | Equipment | Sensitivity sequential procedure | Specificity sequential procedure |
---|---|---|---|
Lam et al. [7] | BF, LIFE | 0.56 (WLB + LIFE) | 0.66 (WLB + LIFE) |
Hirsch et al. [8] | BF, LIFE | 0.79 (WLB + LIFE) | 0.29 (WLB + LIFE) |
Edell et al. [9] | BF, Onco-LIFE | 0.74 (WLB + Onco-LIFE) | 0.75 (WLB + Onco-LIFE) |
Haussinger et al. [10] | BF, D-Light | 0.82 (WLB + D-Light) | 0.58 (WLB + D-light) |
Chhajed et al. [11] | VE, LIFE | 0.72 VE/0.96 LIFE | 0.53 VE/0.23 LIFE |
Chiyo et al. [12] | VE, LIFE, AFI | 0.56 VE/0.97 LIFE/0.8 AFI | 0.5 VE/0.37 LIFE/0.83 AFI |
Ikeda et al. [13] | VE, SAFE | 0.65 VE/0.90 SAFE | 0.49 VE/0.47 SAFE |
Lee et al. [14] | VE, SAFE | 0.86 dual image | 0.94 dual image |
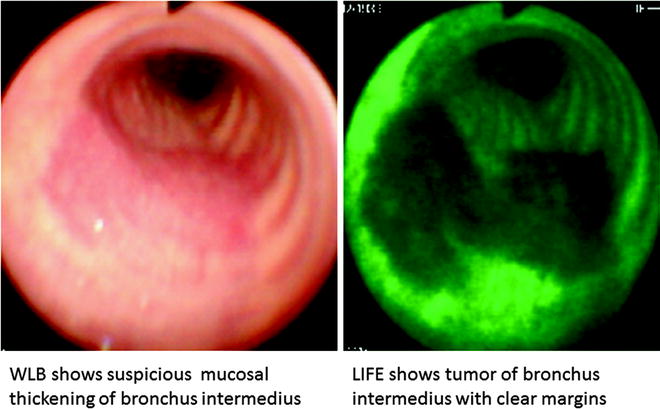
Fig. 21.2
Superficial spreading tumor with margins clearly demarcated by LIFE bronchoscopy
With the advent of the video bronchoscope that has a miniature charge couple device built in its tip which delivers clearer images, sensitivity for premalignant lesions has correspondingly improved without compromising its specificity. Chhajed et al. showed that the addition of video bronchoscopy to LIFE could better select sites for biopsy. However, the procedure required a change of scopes and represented a source of discomfort to the patient and inconvenience to the bronchoscopist. AFI is a video bronchoscope that displays a composite image by integrating three signals: autofluorescence caused by excitation light (395–445 nm), green and red light signals by respective green (550 nm), and red (610 nm) wavelengths. Since hemoglobin absorbs green and minimal red light, in areas with high hemoglobin due to increased vascularity, a feature of dysplasia rather than bronchitis, Chiyo and coworkers showed that AFI was superior to LIFE in discriminating preinvasive and malignant lesions from bronchitis or hyperplasia where LIFE would otherwise indicate as abnormal. Although switching from WLB to AFI involves hitting the button on the bronchoscope, simultaneous comparison of the video-endoscopic and AF images cannot be achieved (Fig. 21.2).
Dual real-time display of video-endoscopic and AF images is recently made possible with SAFE 3000 (Pentax, Tokyo, Japan). SAFE 3000 is a video bronchoscope that uses xenon lamp for WLB and allows real-time color image transmission using the miniature charge couple device that is built into its tip. The AF mode utilizes a diode laser that delivers excitation light to the target from the tip of the scope, and fluorescence from the target is captured by filtering out the wavelength of excitation light with the objective lens. Since both light sources are available, dual real-time display of video and AF bronchoscopic images of the target is achieved. Our study showed that dual real-time display of video-bronchoscopic and AFB images was not only sensitive for detecting preneoplastic lesions (0.86), it was highly specific (0.94) as it provided both functional and anatomic information of the lesion simultaneously (Fig. 21.3). By doing so, it allowed targeted biopsy that led to shorter procedural time, better patient comfort, and safety. Good correlation between visual classification (normal, abnormal, suspicious) and pathology was achieved particularly in identifying previous biopsy site, bronchitis, and airway fibrosis following endobronchial therapy (Fig. 21.4).
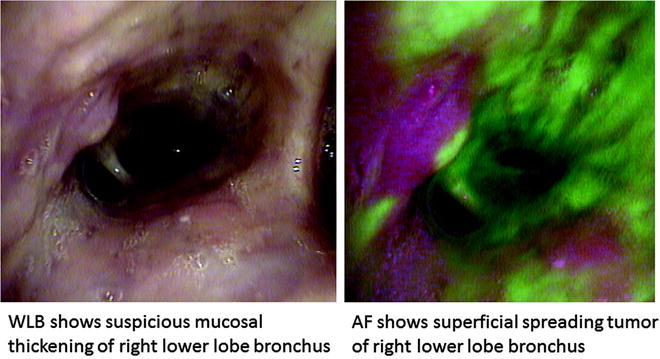
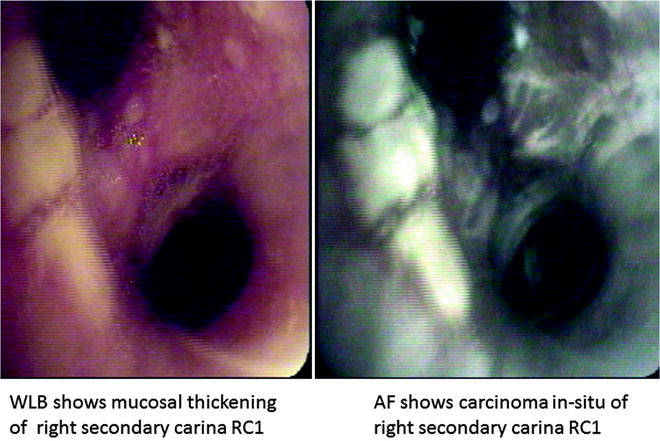
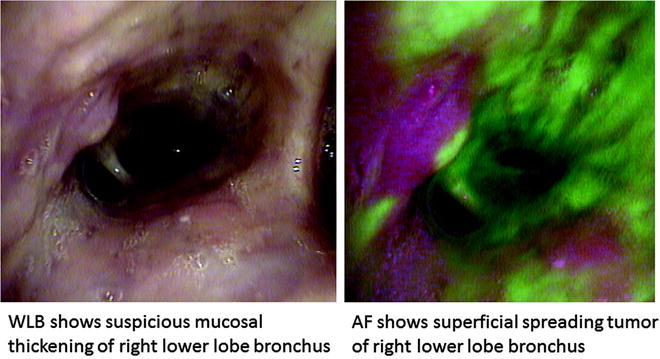
Fig. 21.3
Superficial spreading tumor with margins clearly demarcated by AFI
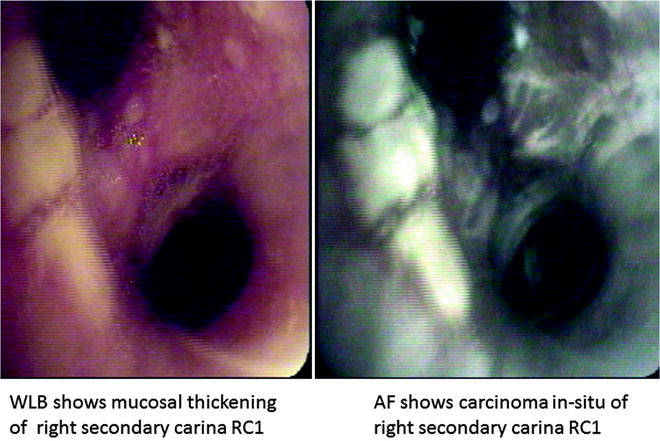
Fig. 21.4
Dual images of carcinoma in situ with SAFE 3000
The newly developed Onco-LIFE device uses both reflectance and fluorescence light for imaging. Blue light (395–445 nm) and small amount of red light (675–720 nm) from a filtered mercury arc lamp are used for illumination. A red-reflectance image together with the green-autofluorescence image is captured by nonimage-intensified charge-coupled device which further enhances the contrast between preinvasive/malignant from normal tissues. By using reflected infrared red light as a reference, it has an advantage over reflected blue or green light as it is less absorbed by hemoglobin and may be less influenced by changes in vascularity associated with airway inflammation, thereby reducing its false-positivity. Red light is also uniformly scattered within the tissue and serves as a reference signal from which differences in light intensities due to changes in angle and distance of the bronchoscope from the bronchial surface are corrected (Fig. 21.5). In addition, Onco-LIFE allows quantitative analysis of the fluorescence image by providing a numeric representation (R/G ratio) of the combined colors in the central portion of the displayed image. R/G ratio of the 16 × 16 pixel square target defined within the displayed brackets is calculated by dividing the average red reflectance with green fluorescence signals captured by the camera (Fig. 21.6). We performed a study correlating pathology of 3,362 biopsies with their corresponding R/G ratios. R/G ratio 0.54 and greater conferred 85% sensitivity and 80% specificity for the detection of high-grade and moderate dysplasia. When visual score (normal, abnormal, suspicious) was combined with R/G ratio, specificity in diagnosing moderate dysplasia or worse was further improved to 88% suggesting that color fluorescence ratio could objectively guide the bronchoscopist in selecting sites for biopsy with good pathological correlation.
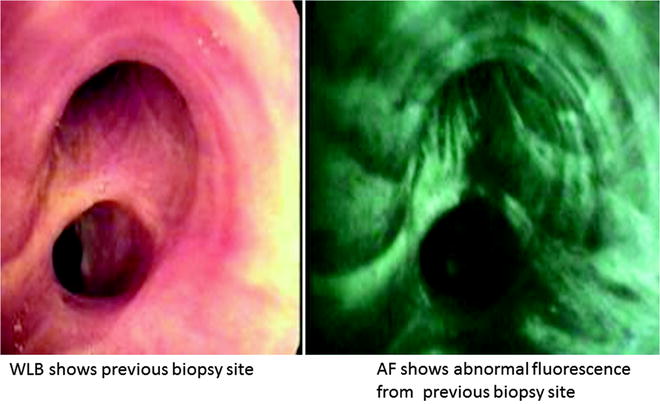
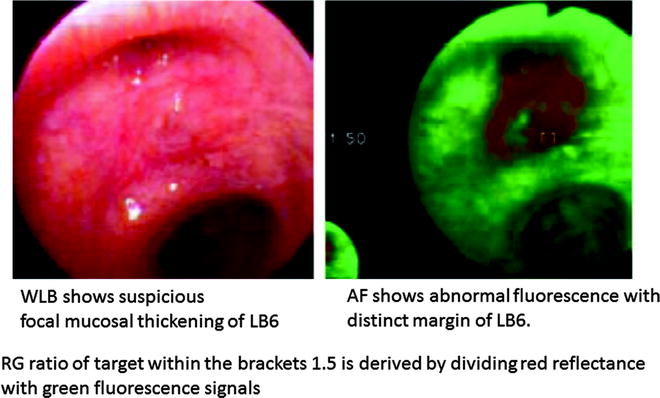
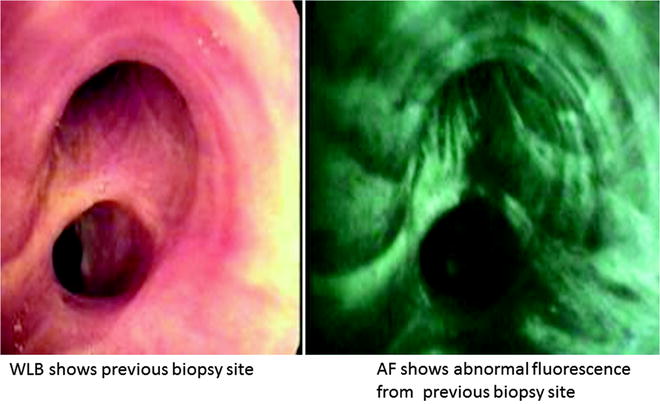
Fig. 21.5
Dual images of previous biopsy site with SAFE 3000
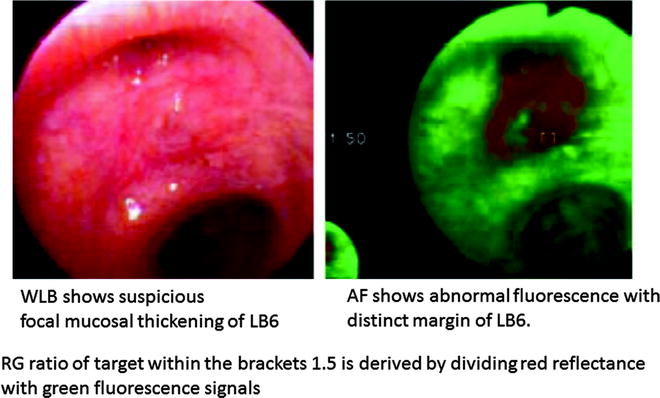
Fig. 21.6
Color fluorescence ratio R/G of carcinoma in situ
Narrow Band Imaging
The conventional RGB sequential video bronchoscope system has a xenon lamp and rotation disk with three RGB optical filters. The rotation disk and monochrome CCD are synchronized, and three band images are generated sequentially. Color images are created by the video processor. Narrow band imaging (NBI) is a novel system that is developed to enhance microvessel structure using a new narrow banding filter on an RGB sequential video bronchoscope system instead of the conventional RGB broadband filter (Fig. 21.7). Wavelengths used by the NBI filter are B1: 400–430 nm, B2: 420–470 nm, and G: 560–590 nm which are in contrast to the conventional RGB broadband filter, namely, B: 400–500 nm, G: 500–600 nm, and R: 600–700 nm. Tissue optical absorption and scattering properties are wavelength dependent, and blue light has a shorter wavelength that reaches into shallow surfaces. The main chromophore in bronchial tissues is hemoglobin which has a maximum absorptive wavelength near 415 nm within the wavelength range for NBI-B1. Therefore, it is presumed that NBI-B1 filter would detect blood vessel structures more accurately than other filters.
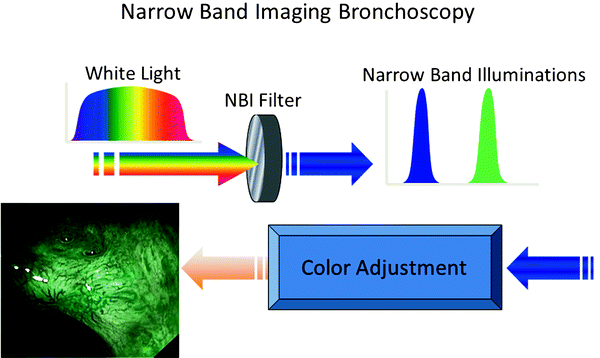
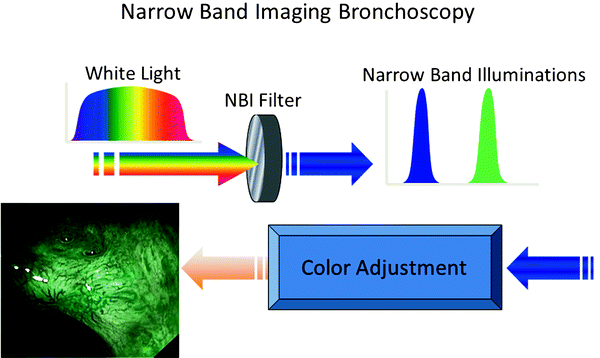
Fig. 21.7
Narrow band imaging enhances microvessel structure by means of narrow banding filter instead of conventional RGB broadband filter
When conventional RGB broadband light is delivered onto tissue surface, light is scattered and absorbed by tissue with little light reflected to form an image; however, if narrow band light is delivered onto same surface, it causes less scattering, thereby enhancing its reflected image. The first publication on NBI combined with high-magnification bronchovideoscopy showed significant association between dotted vessels detected by NBI-B1 and pathological diagnosis of angiogenic squamous dysplasia (ASD) (Fig. 21.8). ASD is a morphological entity of squamous dysplasia of the central airways where collections of capillary blood vessels are projected into dysplastic bronchial epithelium, suggesting that angiogenesis is an early event of lung carcinogenesis. Several studies that investigate into the multistep model of carcinogenesis have indicated that angiogenesis switch occurs in preinvasive lesion prior to invasive tumor development. Although Hirsch and coworkers have demonstrated that fluorescence bronchoscopy was able to detect 75% of ASD cases, AF could not distinguish bronchial squamous dysplasias with ASD from those without. NBI appears to be useful for detecting capillary blood vessels in ASD lesions at sites of abnormal fluorescence. Studies are underway to determine if patients harboring ASD have greater risk for progression to lung cancer. In a pilot study of 22 patients where WLB was performed followed by NBI, NBI identified dysplasia or malignancy not apparent on WLB in 23% of subjects. This was confirmed in a larger trial where 62 patients underwent WLB then randomized to AFI or NBI. The objective was to determine if there was any value combining NBI with AFI and WLB. Respective sensitivities and specificities of AFI and NBI were 3.7/3.0 and 0.5/1.0 relative to WLB. Addition of NBI to AFI did not increase the diagnostic yield significantly, and sequence with which AFI and NBI was performed did not impact findings. The authors proposed NBI as an alternative to AFI for early lung cancer detection since WLB could be converted to NBI by merely pressing a switch button on available bronchoscopy systems (EVIS EXERA II, BF-Q180/BF-IT180, Olympus Medical Systems Corp., Japan) unlike AFI that required a change of bronchoscope (Evis Lucera video bronchoscope BF-F260, Olympus Medical Systems Corp., Japan). NBI was also found to be useful in early detection of head and neck cancers as well as impacting therapeutic decision in the assessment of tumor extension for centrally located lung cancer (Fig. 21.9).