Atrial Fibrillation
Introduction
Atrial fibrillation is the most common arrhythmia and causes rapid, chaotic, and uncoordinated contraction of the atria. It can be classified by presentation as 1) paroxysmal (≥2 episodes lasting <7 days), 2) persistent (sustained episode lasting >7 days or requiring pharmacologic or electrical cardioversion), and 3) permanent (unsuccessful or unattempted cardioversion).1 Longstanding persistent atrial fibrillation is sustained atrial fibrillation lasting >1 year.
The purpose of this chapter is to:
Discuss the pathophysiology of atrial fibrillation.
Discuss the different catheter-based approaches for atrial fibrillation ablation.
Discuss ablation of post-atrial fibrillation ablation left atrial (LA) flutter/tachycardia.
PATHOPHYSIOLOGY
The onset and perpetuation of atrial fibrillation require both triggers and susceptible atrial substrate. The mechanisms of atrial fibrillation are categorized into 1) focal triggers, 2) arrhythmogenic substrate, and 3) modulating factors.
FOCAL TRIGGERS
Spontaneous and rapid firing from the muscular sleeves of the pulmonary veins (PVs) have been identified as the dominant source of triggers for atrial fibrillation (Fig. 15-1).2,3 These muscular sleeves that extend for 1-3 cm into the PVs have a complex architecture whose longitudinal and spiral fiber orientation supports anisotropic conduction and localized reentry. The mechanisms underlying these focal discharges include reentry, automaticity, and triggered activity.4,5 Non-PV triggers of atrial fibrillation (posterior LA; crista terminalis; interatrial septum; other thoracic veins such as superior vena cava [SVC], coronary sinus [CS], vein of Marshall) and supraventricular tachycardias (e.g., atrio-ventricular nodal reentrant tachycardia [AVNRT], orthodromic reciprocating tachycardia [ORT]) have also been reported (Fig. 15-2).6,7,8,9 Sustained, rapid electrical discharges from arrhythmogenic PV foci not only trigger atrial fibrillation but also drive it (focal trigger, rotor, and venous wave hypotheses).10 These focal discharges result in self-sustaining, high-frequency reentrant rotors, which when encountering tissue incapable of 1:1 conduction undergo spatial fragmentation and fibrillatory conduction.11
ARRHYTHMOGENIC SUBSTRATE
Profibrillatory conditions include inhomogeneity of atrial refractoriness (dispersion of refractoriness) and slow conduction occurring with atrial fibrosis, thereby promoting reentry within the atrium.12 A certain number of wavelets is needed to sustain fibrillation, and therefore, a critical mass of atrial tissue is required (multiple wavelet hypothesis).13 Each reentrant wavelet propagates randomly, collides with oncoming wavelets, or extinguishes spontaneously. Atrial fibrillation, itself, causes electrical and anatomical remodeling (tachycardia-mediated atrial myopathy) that begets more atrial fibrillation.
MODULATING FACTORS
The autonomic nervous system plays a critical role in the development of atrial fibrillation with increases in both sympathetic and parasympathetic tone preceding its onset.14,15 Sympathetic stimulation increases automaticity and triggered activity. Stimulation of vagal ganglionic plexi triggers PV firing, shortens atrial refractory periods, and increases dispersion of atrial refractoriness. Four ganglionic plexi are preferentially located in the epicardial fat pads at the junction of the left superior pulmonary vein (LSPV) and atrial roof, postero-inferior junctions of the right and left inferior pulmonary vein (LIPVs), and the anterior border of the right superior PV (RSPV).
ATRIAL FIBRILLATION ABLATION
Prior to catheter ablation, surgical treatment of atrial fibrillation included the Cox-Maze operation, which was based on
the multiple wavelet hypothesis and decreased surface area by compartmentalizing the atrium through a series of strategically placed surgical incisions.16,17,18 Attempt to replicate the surgery using a transvenous ablation catheter was only modestly successful, time-consuming, and associated with a high complication rate.19 Attention turned to the PVs when it was demonstrated that ablation of focal PV triggers could eliminate atrial fibrillation.2,3,20 Longitudinal- and spiral-oriented muscle sleeves spreading from the funnel-shaped antrum into the PVs facilitate anisotropic conduction and reentry. The occurrence of PV stenosis as a complication of this approach shifted the ablation target to the PV ostium (or antrum) with the idea that atrial fibrillation could not occur despite rapid PV firing if the PVs were electrically disconnected from the atrium.21 Catheterbased strategies for atrial fibrillation include 1) PV isolation (PVI) and ablation of non-PV triggers, 2) substrate modification, and 3) vagal denervation. Pre-procedural (CT/MRI) and intraprocedural (intracardiac echocardiography [ICE], pulmonary venography) imaging facilitate an understanding of PV anatomy during ablation (Figs. 15-3 and 15-4).
the multiple wavelet hypothesis and decreased surface area by compartmentalizing the atrium through a series of strategically placed surgical incisions.16,17,18 Attempt to replicate the surgery using a transvenous ablation catheter was only modestly successful, time-consuming, and associated with a high complication rate.19 Attention turned to the PVs when it was demonstrated that ablation of focal PV triggers could eliminate atrial fibrillation.2,3,20 Longitudinal- and spiral-oriented muscle sleeves spreading from the funnel-shaped antrum into the PVs facilitate anisotropic conduction and reentry. The occurrence of PV stenosis as a complication of this approach shifted the ablation target to the PV ostium (or antrum) with the idea that atrial fibrillation could not occur despite rapid PV firing if the PVs were electrically disconnected from the atrium.21 Catheterbased strategies for atrial fibrillation include 1) PV isolation (PVI) and ablation of non-PV triggers, 2) substrate modification, and 3) vagal denervation. Pre-procedural (CT/MRI) and intraprocedural (intracardiac echocardiography [ICE], pulmonary venography) imaging facilitate an understanding of PV anatomy during ablation (Figs. 15-3 and 15-4).
![]() FIGURE 15-1 Spontaneous LSPV firing initiating atrial fibrillation. Early atrial activity (asterisk) recorded from the ablation catheter in the LSPV initiates atrial fibrillation. |
FOCAL TRIGGERS
The cornerstone of atrial fibrillation ablation is PVI—either by radiofrequency (RF) or cryoablation.
Radiofrequency Ablation
Electrophysiologically guided PVI involves recording PV potentials from a circular mapping catheter (e.g., Lasso) situated at the ostium of the targeted vein and therefore generally requires two transeptal punctures unless a double or retained guide wire approach is used. To help avoid entrapment of the catheter in the mitral valve apparatus, the circular mapping catheter should be torqued clockwise (posteriorly) when exiting the transeptal sheath and positioned in the PV away from the more anterior mitral valve. The circular mapping catheter records fused electrograms consisting of a blunt LA (far-field) and high-frequency PV (near-field) potential. During left PV ablation, these electrograms overlap during sinus rhythm because of synchronous activation and best separated by distal CS pacing (Fig. 15-5). Two approaches to PVI include segmental ostial and wide area circumferential ablation (WACA)—the latter having a higher success rate.22,23,24,25,26,27 Segmental ostial ablation successively targets the earliest breakthrough site of PV potentials around the circular mapping catheter until PV muscle conduction is eliminated or dissociated from the LA (Fig. 15-6).22 WACA targets the antrum of the PVs (≥5 mm outside the PV ostium), debulking the atrium and causing PVI with contiguous focal lesions around the PVs individually, ipsilaterally, or entirely en bloc (Fig. 15-7).23 Voltage abatement (≤0.05 mV) within encircled areas validate line continuity. Clues that the ablation catheter
is in the PV where RF delivery should be avoided include 1) fluoroscopic demonstration of the catheter tip beyond the cardiac silhouette, 2) ICE imaging of catheter tip beyond the PV ostium, 3) loss of electrical signals, and 4) increase in catheter impedance (>140-150 ohms). The endpoint of PVI is entrance block (elimination or dissociation of PV potentials from the LA) and exit block (PV stimulation causes PV sleeve capture without conduction to the atrium) (Figs. 15-8, 15-9, 15-10, 15-11 and 15-12).
is in the PV where RF delivery should be avoided include 1) fluoroscopic demonstration of the catheter tip beyond the cardiac silhouette, 2) ICE imaging of catheter tip beyond the PV ostium, 3) loss of electrical signals, and 4) increase in catheter impedance (>140-150 ohms). The endpoint of PVI is entrance block (elimination or dissociation of PV potentials from the LA) and exit block (PV stimulation causes PV sleeve capture without conduction to the atrium) (Figs. 15-8, 15-9, 15-10, 15-11 and 15-12).
![]() FIGURE 15-2 Degeneration of AVNRT (top) and ORT (bottom) to atrial fibrillation. ORT uses a left free wall accessory pathway (AP). |
![]() FIGURE 15-4 PV anatomy ICE. A PentaRay catheter is positioned in each of the four PVs. The red tags denote ablation lesions around the PV antra. |
Complications
Extensive ablation directed toward the thin LA wall can cause collateral damage to adjacent structures (esophagus, phrenic nerve). Techniques that might help avoid esophageal injury during ablation on the posterior wall include 1) limiting RF energy (e.g., 25 Watts or high power/short duration [50 Watts/2-5 sec]), 2) decreasing tissue contact pressure (avoiding perpendicular orientation of the catheter tip and posterior LA), 3) moving the tip of the ablation catheter during RF delivery (avoid time-dependent tissue heating), and 4) real-time esophageal monitoring during ablation (luminal esophageal temperature [LET] probe, ICE visualization, barium paste in the esophagus) (Fig. 15-13).4,28 Techniques that might help avoid phrenic nerve injury, particularly with ablation near the RSPV, include 1) high-output pacing from the ablation site to ensure absence of phrenic nerve stimulation prior to RF delivery and 2) fluoroscopic monitoring or phrenic nerve pacing from the right subclavian vein to ensure continued right diaphragmatic function during ablation.4
![]() FIGURE 15-10 Entrance block. RF delivery to the RSPV (fourth Lasso dipole) causes abrupt loss (asterisk) of PV potentials. White arrows denote circular mapping catheter (Lasso) in RSPV. |
Pulmonary Vein Potential Pitfalls
After PVI, adenosine or isoproterenol can unmask dormant PV conduction and/or non-PV triggers and, therefore, need for further ablation.29,30,31,32 Far-field electrograms from neighboring electrically active structures (LA appendage [LAA] for LSPV and SVC for RSPV) can be misinterpreted as PV potentials resulting in unnecessary RF applications, particularly with widely spaced electrode pairs having a larger “antenna” (Figs. 15-14 and 15-15). Far-field electrograms can be differentiated from true PV potentials by 1) morphology, 2) distribution, and 3) pacing techniques. PV potentials demonstrate near-field characteristics (high frequency, sharp, narrow width) and are circumferentially distributed around the PV, while far-field electrograms (low frequency, dull, wider width) are segmentally distributed to sites overlying the electrically active neighboring structure. Pacing the neighboring structure (e.g., LAA) and capturing the electrogram (potential anticipation) confirms a far-field signal (Fig. 15-14).33,34 (Conversely, when demonstrating exit block by pacing within the PV, it is also important not to far-field capture the electrically active neighboring structure and therefore atrium, which could be falsely interpreted as persistent conduction.)
Cryoablation
Rather than point-by-point focal RF ablation, cryoablation is an alternative PVI technique using a balloon catheter to occlude the PV and liquid nitrogen to freeze its antrum.35,36,37 A circular mapping catheter inserted through the lumen of the cryoballoon allows monitoring of PV potentials while providing distal support, and therefore, only a single transeptal puncture is required. The transeptal puncture site should be low and anterior on the fossa ovalis allowing for a large turning radius of the cryocatheter to reach the posterior and superior PVs (particularly, the more difficult right inferior pulmonary vein [RIPV]). Keeping the cryoballoon coaxial to the vein with maintenance of forward pressure, advancing the sheath to the back hemisphere of the balloon for proximal support, and using the circular mapping catheter as a distal luminal supporting wire (“rail”) in different vein branches (inferior for inferior veins/superior for superior veins) facilitate balloon occlusion of the targeted vein. Because the balloon heads superiorly toward the PV antrum, inferior leaks during initial attempts at vein occlusion are common (worst site of balloon-to-PV contact) and can be sealed by a 1) “pull down” technique (pulling the entire assembly down to prevent the balloon from “riding” upward) or 2) curving the cryosheath and pushing the assembly inferiorly downward toward the antrum (“hockey stick” sign—especially for RIPV). A large common or oval ostium can make complete occlusion difficult. Signs of PV occlusion are 1) difficult contrast injection with retention in the vein (“hang up”), 2) absence of leaks on color Doppler sweep, and 3) pulmonary venous pressure waveforms (transition from LA [venous A and V wave] to pulmonary
artery pressure [only higher pressure V wave]). Because of the different viscosities between contrast and saline, it is important to adequately flush any dye out of the lumen of the cryoballoon in order to accurately record pulmonary venous pressures (Figs. 15-16, 15-17, 15-18, 15-19 and 15-20).38 Optimal cryoablation endpoints include 1) nadir temperatures (<−50°C), 2) time to isolation (TTI) <60 sec, 3) freezing time (−30°C by 30 sec, −40°C by 60 sec) (rapid freezing with shorter freezing times correlates with effective cryoablation), and 4) rewarming time (interval thaw time to 0°C by ≥10 sec) (longer rewarming times indicate more therapeutic ice crystallization with tissue-ice bonding at 0°C).39,40,41,42 In contrast to the abrupt loss of PV potentials that signify entrance block during RF ablation, cryoablation causes LA-PV delay prior to disappearance of PV potentials (Fig. 15-21).43 (A distally positioned circular mapping catheter can be pulled back to the nose of the cryoballoon within 10 sec of the cryofreeze to record PV potential before the central lumen freezes.) Pacing from the circular mapping catheter can also show PV-LA delay prior to exit block. The dosing and duration of cryofreeze applications depends on how successful the cryoablation endpoints were achieved (e.g., 180 sec [TTI <90 sec] or 150 sec [TTI <30 sec]). After the freeze, the cryoballoon should not be manipulated until the temperature reaches 35°C during the thaw in order to avoid tissue tearing from cryoadherence.
artery pressure [only higher pressure V wave]). Because of the different viscosities between contrast and saline, it is important to adequately flush any dye out of the lumen of the cryoballoon in order to accurately record pulmonary venous pressures (Figs. 15-16, 15-17, 15-18, 15-19 and 15-20).38 Optimal cryoablation endpoints include 1) nadir temperatures (<−50°C), 2) time to isolation (TTI) <60 sec, 3) freezing time (−30°C by 30 sec, −40°C by 60 sec) (rapid freezing with shorter freezing times correlates with effective cryoablation), and 4) rewarming time (interval thaw time to 0°C by ≥10 sec) (longer rewarming times indicate more therapeutic ice crystallization with tissue-ice bonding at 0°C).39,40,41,42 In contrast to the abrupt loss of PV potentials that signify entrance block during RF ablation, cryoablation causes LA-PV delay prior to disappearance of PV potentials (Fig. 15-21).43 (A distally positioned circular mapping catheter can be pulled back to the nose of the cryoballoon within 10 sec of the cryofreeze to record PV potential before the central lumen freezes.) Pacing from the circular mapping catheter can also show PV-LA delay prior to exit block. The dosing and duration of cryofreeze applications depends on how successful the cryoablation endpoints were achieved (e.g., 180 sec [TTI <90 sec] or 150 sec [TTI <30 sec]). After the freeze, the cryoballoon should not be manipulated until the temperature reaches 35°C during the thaw in order to avoid tissue tearing from cryoadherence.
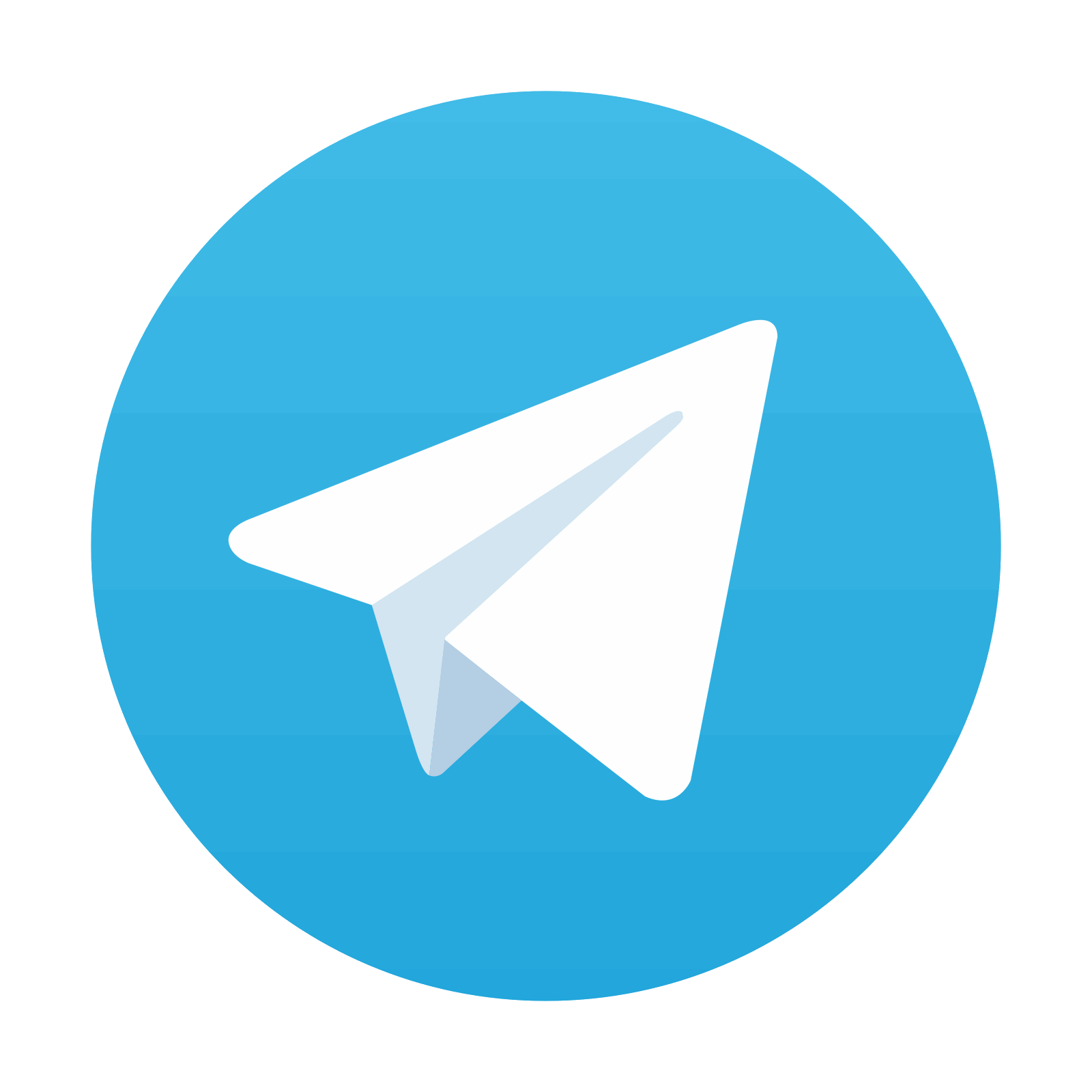
Stay updated, free articles. Join our Telegram channel

Full access? Get Clinical Tree
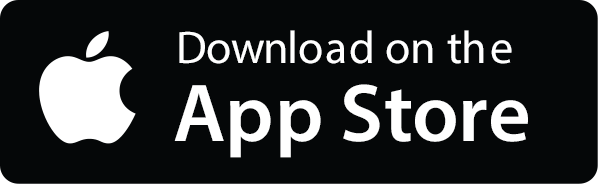
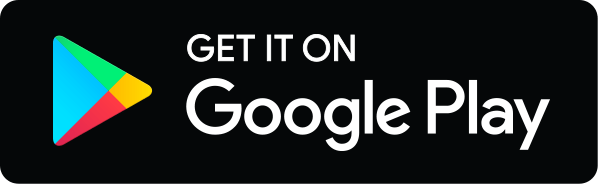
