Atherectomy and Aspiration Devices
Abhiram Prasad
Significant advances over recent years in the equipment and techniques used in coronary interventions have greatly improved the safety and efficacy of percutaneous revascularization. The improvement in procedural success has widened the spectrum of disease severity now considered appropriate for percutaneous coronary intervention (PCI). Although stents have become the most commonly used device, severe coronary calcification and thrombus in native coronary artery lesions, ostial and bifurcation lesions, and interventions in degenerated saphenous vein grafts (SVGs) are angiographic characteristics that still predict adverse outcomes and restenosis (1). The limitation of angioplasty and stents to treat complex lesions has resulted in the development of numerous alternative devices and techniques, such as rotational atherectomy (RA), directional coronary atherectomy (DCA), rheolytic thrombectomy (AngioJet), and the X-Sizer thromboatherectomy catheter; these new approaches either remove plaque (atherectomy) or thrombus (thrombectomy) and have proved to be of variable efficacy and ease of use.
ROTATIONAL ATHERECTOMY
RA (Rotablator; Boston Scientific, Natick, Massachusetts) is a low-power, high-speed rotary device. This principle of low power and high speed allows the preferential ablation of inelastic atheroma while sparing relatively normal vessel wall, which is elastic and hence deflected away from the advancing burr (2). The rotation of the burr also aids in reducing friction, which facilitates the advancement and withdrawal of the burr. The leading edge of the elliptical, nickel-plated brass burr is coated with diamond chips that protrude 5 μm above the nickel plating, resulting in an abrasive surface. A compressed air or nitrogen-driven flexible drive shaft is connected to the burr. The shaft is covered by a 4.3 Fr sheath that serves to isolate the spinning shaft from the guide and artery and delivers a flush containing vasodilator drugs. The rotational speed is regulated by the gas pressure, and the device is activated via a foot pedal, which also initiates the locking mechanism that prevents the guidewire from spinning while the burr is rotating. The burrs are available in 1.25, 1.5, 1.75, 2.0, 2.15, 2.25, 2.38, and 2.5 mm diameters for use in the coronary circulation. Guides measuring 7 Fr, 9 Fr, and 10 Fr can accommodate burrs up to 1.75 mm, 2.38 mm, and 2.5 mm, respectively. The drive shafts are attached to the advancer, which allows the operator to advance and withdraw the burr.
Clinical Data
The feasibility and efficacy of RA has been documented in several registries (3, 4, 5) and subsequent randomized trials. The Excimer Rotational Balloon Angioplasty Comparison (ERBAC) trial compared RA (maximum burr-to-artery ratio 0.66) to percutaneous transluminal coronary angioplasty (PTCA) or excimer laser. At follow-up, restenosis and target vessel revascularization (TVR) were more common in the RA group compared with PTCA (6). Acute procedural success, restenosis, and target vessel failure rates were not different in the randomized, multicenter Dilation versus Ablation Revascularization Trial (DART) comparing RA with PTCA in coronary arteries less than 3 mm in diameter (7). The findings from these trials suggested that RA did not improve procedural success or prevent restenosis compared to PTCA.
The Study to Determine Rotablator and Transluminal Angioplasty Strategy (STRATAS) and the Coronary Angioplasty and Rotational Atherectomy Trial (CARAT)
investigated the role of aggressive debulking (8,9). STRATAS compared an aggressive RA strategy (burr-to-artery ratio ≥0.7) in conjunction with low-pressure PTCA (1 atm) with a routine RA strategy (burr-to-artery ratio <0.7) followed by conventional PTCA. The hypothesis of this study was that aggressive debulking with minimal vessel stretch may result in lower restenosis. Angiographic success, major complication rates, and 6-month event rates were similar for both strategies. Similarly, CARAT, a multicenter randomized study, compared aggressive debulking (burr-to-artery ratio ≥0.7) to a strategy of lesion modification by routine RA (burr-to-artery ratio <0.7). No differences were observed in acute or long-term outcomes. However, aggressive debulking was accompanied by a greater likelihood of serious angiographic complications (12.7% versus 5.1%). Thus, these trials did not support the use of aggressive debulking with RA.
investigated the role of aggressive debulking (8,9). STRATAS compared an aggressive RA strategy (burr-to-artery ratio ≥0.7) in conjunction with low-pressure PTCA (1 atm) with a routine RA strategy (burr-to-artery ratio <0.7) followed by conventional PTCA. The hypothesis of this study was that aggressive debulking with minimal vessel stretch may result in lower restenosis. Angiographic success, major complication rates, and 6-month event rates were similar for both strategies. Similarly, CARAT, a multicenter randomized study, compared aggressive debulking (burr-to-artery ratio ≥0.7) to a strategy of lesion modification by routine RA (burr-to-artery ratio <0.7). No differences were observed in acute or long-term outcomes. However, aggressive debulking was accompanied by a greater likelihood of serious angiographic complications (12.7% versus 5.1%). Thus, these trials did not support the use of aggressive debulking with RA.
In the stent era, the concept of lesion modification was evaluated in the Stenting Post Rotational Atherectomy trial (SPORT) (10) to assess whether reduction in plaque mass and increasing vascular compliance lead to improved stent deployment. Patients were randomized to PTCA plus stent or RA plus stent. The latter approach was associated with higher procedural success, postprocedural minimal luminal diameter (MLD), and acute gain; however, no differences were observed in in-hospital major adverse coronary event (MACE) rates, 6-month TVR rates (11.5% versus 14.4%), or angiographic restenosis (27.6% versus 30.4%). Although the findings of the study did not support the routine use of RA prior to stent implantation, the data confirmed that RA is capable of plaque modification.
The efficacy of RA for the treatment of in-stent restenosis has been investigated in the Randomized Trial of Rotational Atherectomy versus Balloon Angioplasty for Diffuse In-stent Restenosis (ROSTER) and Angioplasty versus Rotational Atherectomy for the Treatment of Diffuse In-stent Restenosis (ARTIST) trials (11,12). In the ROSTER trial, RA in conjunction with PTCA at low pressures resulted in a lower primary endpoint of TLR (32% versus 45%, p = 0.04) compared to conventional PTCA alone. In contrast, a similar strategy was inferior to conventional PTCA in the ARTIST trial. The primary endpoint of MLD at 6 months was greater in the RA group (1.20 ± 0.56 versus 0.99 ± 0.62, p = 0.008). One explanation for the discordant results between the two trials is that, in the ROSTER trial, patients with underexpanded stents, who would not benefit from RA, were excluded from the study by pretreatment intravascular ultrasound. Nevertheless, the conflicting results of the two trials have diminished the enthusiasm for using RA for the treatment of in-stent restenosis.
Patient Selection
RA produces microparticles, usually less than 10 μm, that may result in microvascular obstruction and ischemic complications. The device also is associated with the phenomenon of microcavitation that can transiently obstruct the microcirculation. Both microembolization and microcavitation may contribute to the slow- or no-reflow phenomenon often seen. Therefore, RA should be used with great caution in patients with reduced left ventricular systolic function, multivessel disease, long lesions with a large plaque burden, and in patients with unstable angina who are least likely to tolerate ischemia insult (13). The female gender also has been associated with an increased risk of ischemic complications (3).
Lesion Selection
RA is most suitable for very rigid, calcified, fibrotic lesions that are resistant to dilatation using PTCA alone. It may facilitate PTCA and stenting by modifying the characteristics of the plaque by removing superficial calcification. Thus, RA is used most often in heavily calcified lesions (3,4,14). The use of the device also has been proposed for the treatment of bifurcation lesions (to reduce plaque shift), fibrotic ostial lesions, and in-stent restenosis (15,16). However, its applications for these indications have decreased over recent years in the absence of any definitive evidence that the technique is superior to PTCA and stenting. RA is relatively contraindicated in SVG lesions, lesions with visible thrombus or dissection, vessels with poor distal runoff, and eccentric lesions that are located on sharp bends or highly angulated (≥60 degrees) lesions because of the increased risk of perforation and dissection (3).
Procedural Technique
Guide selection is determined by the size of the largest burr anticipated to be used. Damage to the guiding catheter may be encountered if an oversized burr is used relative to the guiding catheter. The shape of the guiding catheter should be based on the size of the aortic root and coronary anatomy to allow coaxial intubation of the coronary artery and to avoid sharp angulation that may hamper the advancement of the burr through the guide. The RotaWire Floppy is the most frequently used guidewire; it has a 0.09- to 0.05-inch tapered core and a 0.014-inch radiopaque floppy tip. This wire has replaced the previously used A-wire and C-wire, which were stiffer and provided more support but frequently caused wire bias, pseudostenosis, and hence predisposed the patient to complications. The platinum tip of the wire must be distal to the lesion, because the burr is unable to track over this segment of the wire. The tip of the wire should not be in a minor branch, because inadvertent spinning of the wire with the burr would lead to significant vessel injury.
The final burr size should be selected to achieve a burr-to-artery ratio of approximately 0.6 to 0.7. A smaller burr should be used initially, followed by a stepwise increase in the burr size. Once the burr is loaded onto the RotaWire, and the wire clip is applied (to prevent the wire from
spinning), the burr platform speed should be established. A speed of 160,000 to 180,000 rpm is used for burrs less than 2 mm, whereas 150,000 to 160,000 rpm is preferable for larger burrs. A lower speed of 7,000 rpm, known as the Dynaglide setting, is used to minimize friction during burr exchange. The burr should be activated in the coronary artery proximal to the lesion to achieve platform speed before making contact with the lesion. A substantial drop in rpm (greater than 5,000) should be avoided, and each run should not last more than 30 to 40 seconds, with brief intermittent contact with the lesion. These techniques minimize complications and late restenosis.
spinning), the burr platform speed should be established. A speed of 160,000 to 180,000 rpm is used for burrs less than 2 mm, whereas 150,000 to 160,000 rpm is preferable for larger burrs. A lower speed of 7,000 rpm, known as the Dynaglide setting, is used to minimize friction during burr exchange. The burr should be activated in the coronary artery proximal to the lesion to achieve platform speed before making contact with the lesion. A substantial drop in rpm (greater than 5,000) should be avoided, and each run should not last more than 30 to 40 seconds, with brief intermittent contact with the lesion. These techniques minimize complications and late restenosis.
Adjunctive Pharmacotherapy
A mixture of vasodilators that contain nitroglycerin, verapamil, and adenosine is given through the Rotablator flush to minimize the occurrence of epicardial and microvascular spasm and the no-reflow phenomenon. Systemic intravenous nitroglycerin also may be administered to reduce epicardial spasm. Intravenous aminophylline (200 to 300 mg) may be used to prevent bradyarrhythmias and may circumvent the requirement for temporary pacemaker placement.
Complications
Obstruction of the microcirculation caused by excessive and large microparticles produced by ablation leads to slow- or no-reflow phenomenon. This complication may be minimized by starting with relatively smaller burrs, using lower speeds, and avoiding excessive deceleration (>5,000 rpm). Slow or no reflow can be treated with intracoronary verapamil, adenosine, or nitroprusside. The differential diagnosis of impaired flow after ablation includes epicardial spasm and coronary dissection. Dissection is important to recognize, because its presence precludes further RA and requires treatment with adjunctive angioplasty and stenting. Coronary perforation may occur in tortuous vessels and in eccentric and long lesions. Guidewire bias may direct the burr preferentially into one direction, which also predisposes to perforation. Burr stalls frequently result in dissection and perforation. Treatment of perforation depends on its size. Small perforations may seal following the reversal of anticoagulation and occlusive balloon dilatation over the site. Large perforations may require the placement of covered stents, such as the JoMed stent (JoMed, Helsingborg, Sweden).
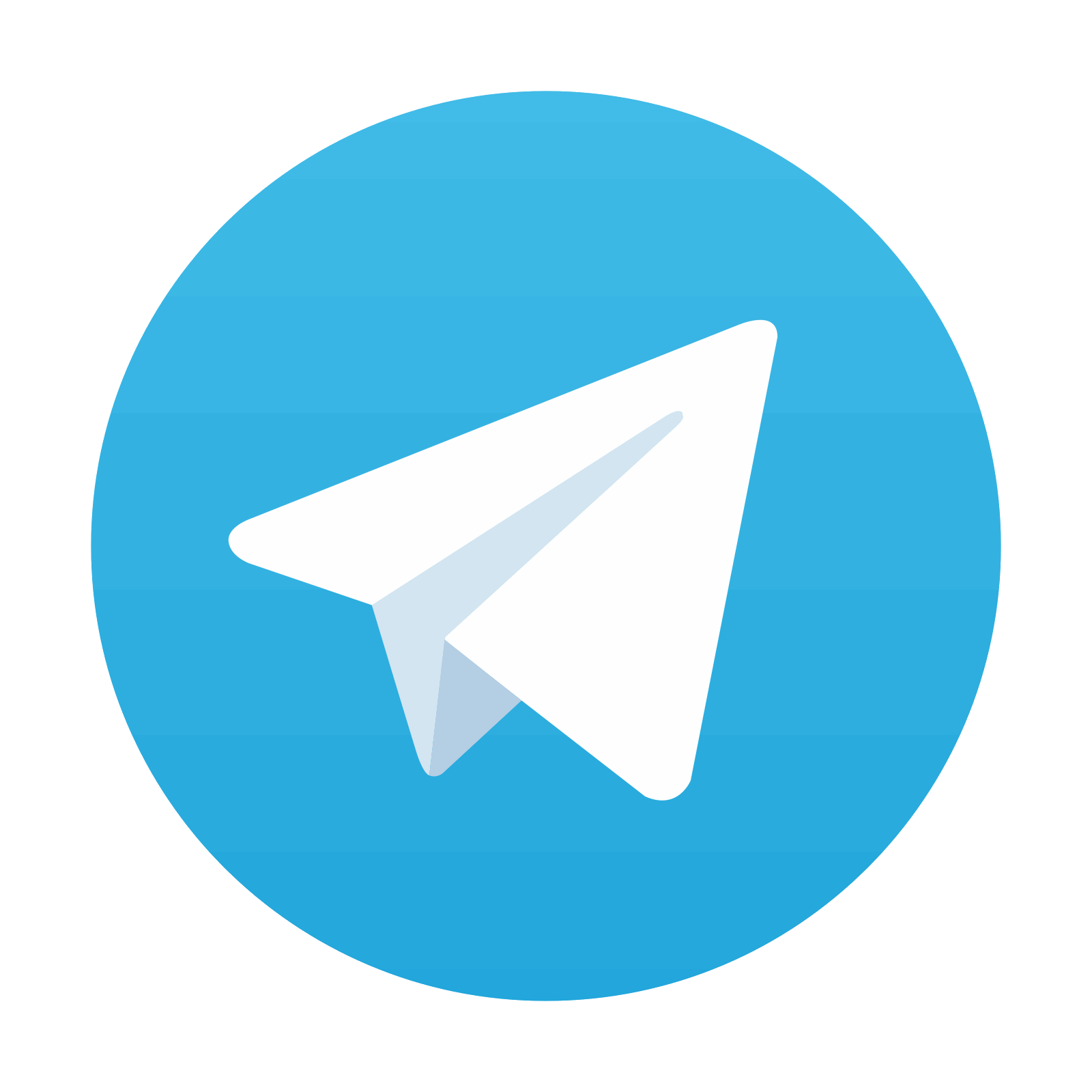
Stay updated, free articles. Join our Telegram channel

Full access? Get Clinical Tree
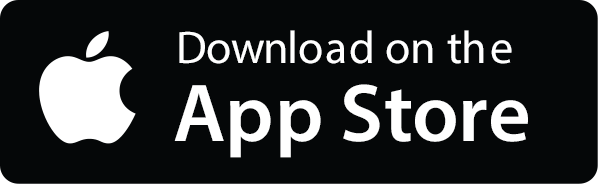
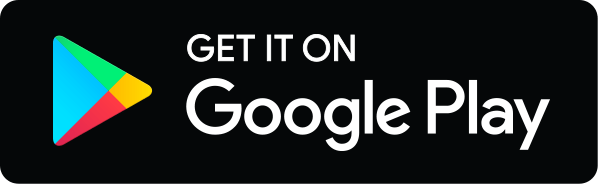