Background
Ischemic mitral regurgitation (IMR) results from mitral leaflet tethering from left ventricular remodeling. Heterogeneity in local or global left ventricular remodeling can result in differential tethering patterns and affect mitral valve function and the degree of mitral regurgitation. The aims of this study were to compare mitral valve geometry in asymmetric and symmetric tethering patterns using three-dimensional transesophageal echocardiography and to examine the impact of tethering pattern on IMR severity.
Methods
Sixty-two patients with moderate or greater IMR underwent three-dimensional transesophageal echocardiography for the assessment of mitral valve geometry. Symmetric and asymmetric tethering patterns were determined by mitral regurgitation jet direction and coaptation of the mitral leaflets. The ratio of posterior to anterior leaflet tethering angle was a measure of tethering pattern (the higher the ratio, the more asymmetric the pattern). Overall tethering degree was assessed by tenting volume (TV).
Results
Compared with the symmetric group, the asymmetric group had less annular dilatation, greater annular heights (10.3 ± 1.9 vs 8.5 ± 1.9 mm, P < .01), greater ratios of posterior to anterior leaflet tethering angle (3.19 ± 0.88 vs 1.95 ± 0.46, P < .01), and smaller TVs with more posterior displacement of the coaptation line. Vena contracta normalized to TV was greater in the asymmetric group (0.38 ± 0.24 vs 0.19 ± 0.13 cm/mL, P < .01). Multivariate analysis showed that both ratio of posterior to anterior leaflet tethering angle (β = 0.46, P < .001) and TV (β = 0.41, P = .001) were predictors of IMR severity.
Conclusions
Differences in mitral valve geometry are observed between asymmetric and symmetric tethering patterns in IMR. IMR degree is affected by both the pattern of tethering and the total degree of tethering. For the same degree of tethering, an asymmetric pattern is associated with increased MR severity. The pattern of mitral leaflet tethering may be considered in therapeutic decision making.
Ischemic mitral regurgitation (IMR) is a common and serious sequela of myocardial infarction and is associated with a poor prognosis. IMR primarily results from leaflet tethering due to papillary muscle displacement from ischemic left ventricular (LV) wall remodeling, with a contribution from mitral annular deformation. Heterogeneity in local or global LV remodeling could result in differential leaflet tethering patterns. A symmetric tethering pattern is characterized by apical tethering of both leaflets to a similar degree, resulting in symmetric coaptation and a centrally directed mitral regurgitation (MR) jet. An asymmetric tethering pattern has predominant posterior tethering of both leaflets, resulting in asymmetric coaptation and a posteriorly directed MR jet. Tethering pattern may affect mitral valve function and MR degree because of differences in coaptation. We hypothesized that there are mitral valve geometric differences between symmetric and asymmetric tethering patterns, which influence the degree of MR. Specifically, an asymmetric pattern predisposes to having a greater degree of MR because the asymmetric coaptation is unfavorable for an effective coaptational surface compared with a symmetric pattern. Although two-dimensional (2D) echocardiography has previously described mitral valve tethering patterns, three-dimensional (3D) echocardiography can provide greater quantitative assessment of the differences in mitral valve geometry between symmetric and asymmetric tethering patterns. Therefore, the aims of the study were to use 3D transesophageal echocardiography (TEE) to (1) examine the quantitative differences in mitral valve geometry between asymmetric and symmetric tethering patterns in patients with IMR and (2) examine the impact of these quantitative differences and tethering pattern on IMR severity.
Methods
Patient Population
From February 2009 to November 2011, a total of 62 patients with IMR referred for cardiac surgery for coronary artery disease were retrospectively studied. Inclusion criteria were limited to patients with moderate or greater IMR due to restricted leaflet motion (Carpentier functional classification type IIIb). Ischemic etiology was confirmed by coronary angiography. Exclusion criteria included structural abnormalities of the mitral apparatus (such as rheumatic, degenerative, or congenital mitral valve disease; mitral valve mass; vegetation; or fenestration), irregular rhythm, and inadequate image quality for 3D analysis. Two thirds of patients were from the IMR clinical trials of the Cardiothoracic Surgical Trials Network, and the remaining patients were from Massachusetts General Hospital. All patients underwent transthoracic echocardiography and TEE as part of the study protocol for the clinical trials or routine clinical care. The echocardiographic protocol and data were approved by the committee of the Massachusetts General Hospital Institutional Review Board.
Echocardiographic Examination
2D Transthoracic Echocardiographic Assessment
Two-dimensional transthoracic echocardiography was performed by experienced sonographers according to a standardized echocardiographic protocol using an iE33 ultrasound system (Philips Medical Systems, Andover, MA) with an S5-1 probe.
Doppler color flow mapping was used to evaluate the direction of the MR jets. The primary direction of the MR jets was classified as central or eccentric. Asymmetric or symmetric tethering patterns were determined by asymmetric or symmetric coaptation of the mitral leaflets and the direction of the MR jet. Specifically, asymmetric tethering pattern was defined by (1) asymmetric coaptation (predominant posterior tethering of both leaflets, with the distal anterior leaflet, less restricted, sliding up toward the midlevel of the posterior leaflet, creating a “pseudoprolapse” or “hockey stick configuration”) and (2) an eccentric, posteriorly directed MR jet consistent with the asymmetric coaptation. Symmetric tethering pattern was defined by (1) symmetric coaptation (symmetric and predominant apical tethering of both leaflets into the left ventricle) and (2) a central MR jet consistent with the symmetric coaptation. Tethering patterns were assessed on apical four-chamber and long-axis views as well as the parasternal long-axis view at midsystole ( Figure 1 ).

Color flow Doppler images of the MR jet were acquired at a Nyquist limit of 50 to 60 cm/sec and a color gain that just eliminated random color speckle from nonmoving regions. Quantification of MR was performed as recommended by the American Society of Echocardiography using an integrative method, including measurement of vena contracta (VC) width, effective regurgitant orifice area (EROA), and the ratio of mitral regurgitant jet area to left atrial area. VC width was measured as the narrowest width of the proximal jet measured from a magnified parasternal long-axis view. EROA was calculated using the proximal isovelocity surface area (PISA) method from apical views, using the formula EROA = 2π × R PISA 2 × V aliasing / V max , where R PISA is the maximal PISA radius (centimeters), V aliasing is the aliasing velocity of the proximal flow convergence (centimeters per second), and V max is the maximal velocity of the continuous-wave Doppler MR signal (centimeters per second). Jet area/left atrial area ratio was calculated as the ratio of MR jet area to left atrial area, averaged from apical four-chamber and two-chamber views. Severe MR was defined by the presence of at least two of three color Doppler methods categorizing MR severity as severe by American Society of Echocardiography–recommended thresholds.
LV end-diastolic volume (EDV) and end-systolic volume (ESV) were measured using the biplane Simpson’s rule. Ejection fraction (EF) was calculated as (EDV − ESV)/EDV. Regional wall motion was scored using a 16-segment model on a five-point grading scale (1 = normal, 2 = hypokinetic, 3 = akinetic, 4 = dyskinetic, 5 =aneurysmal). A wall motion score index was calculated as the average value of the analyzed segments.
3D Transesophageal Echocardiographic Acquisition and Analysis
TEE was performed by using a fully sampled matrix transducer (X7-2t). The transducer was positioned at the midesophageal four-chamber view. To avoid stitching artifacts, special care was taken to stabilize the probe during data acquisition. The acquisition was electrocardiographically gated, with sector settings optimized for resolution. Either full volume or zoom-mode acquisition of the entire mitral valve and aortic valve was used. Three-dimensional data sets were analyzed using the commercial software MVQ (Mitral Valve Quantification, QLAB version 8.0; Philips Medical Systems) according to the software manufacturer’s recommended method. The aortic annulus, mitral annulus, mitral leaflets, and papillary muscles were manually traced at midsystole. Briefly, four key reference points indicating the orientation of the mitral annulus (anterior, posterior, anterolateral, and posteromedial points) were marked on two cut planes representing the three-chamber view and an orthogonal plane. The mitral annulus was then outlined by marking a total of 16 annular points at the leaflet insertion on eight equiangular image planes intersecting at the center of the mitral annulus. To delineate the leaflet geometry and coaptation points, mitral leaflets were traced in a set of 17 equidistant parallel long-axis planes. The anterior and posterior papillary muscle tips were identified by comparing the adjacent long-axis planes. Finally, QLAB was used to reconstruct the mitral valve apparatus ( Figure 2 ), generating parameters representing 3D geometry, including mitral annular intercommissural (CC) diameter, anteroposterior diameter, height, circumference, 2D projection area, aortic-mitral angle, anterior leaflet tethering angle, posterior leaflet tethering angle, tenting volume, tenting height, and leaflet closure areas. Leaflet closure areas were the areas of leaflets involved in coaptation, defined as the closed leaflet surface between the left ventricle and the left atrium in midsystole. Leaflet closure area was the summation of anterior mitral leaflet closure area and posterior mitral leaflet closure area. The following calculations were also made: (1) the ratio of mitral annular height to CC diameter, representing the degree of saddle shape ; (2) the ratio of posterior to anterior leaflet tethering angle, representing a measure of the tethering pattern (the higher the ratio, the more asymmetric the pattern); (3) the ratio of anterior to posterior leaflet closure area, representing the position of the coaptation line; and (4) the ratio of VC width or EROA to tenting volume or tenting height, representing MR severity relative to tenting volume or tenting height.

To assess the reproducibility of the measurements of mitral valve geometry by 3D TEE, 15 patients were randomly selected. To evaluate interobserver variability, two independent observers measured the mitral valve geometry using the same 3D data sets. To test intraobserver variability, measurements were repeated 3 months later by the same observer.
Statistical Analysis
Measurements are expressed as mean ± SD for continuous variables and as percentages for categorical variables. Differences between groups were analyzed using unpaired Student’s t tests for continuous variables and Mann-Whitney-Wilcoxon rank-sum tests for noncontinuous variables. Chi-square tests were used to compare frequencies between groups. The linear association between continuous variables was made using Pearson’s correlation coefficient. Univariate regression analysis was used to evaluate the association between the mitral geometric measurements and IMR severity assessed by VC width. Multivariate linear regression with a stepwise approach was further performed among variables with P values < .10 by univariate regression analysis to identify the independent determinants associated with IMR severity. Interobserver and intraobserver reproducibility was tested using intraclass correlation coefficients. P values < .05 were considered statistically significant. SPSS software was used for statistical analysis (SPSS, Inc, Chicago, IL).
Results
Assessment of MR and LV by 2D Transthoracic Echocardiography
Patients were divided into asymmetric ( n = 22) and symmetric ( n = 40) groups as defined under “Methods.” The clinical and echocardiographic characteristics of the asymmetric and symmetric groups are demonstrated in Table 1 .There were no significant differences in age, gender, and body surface area between the two groups. The symmetric group had significantly lower EFs (37 ± 11% vs 44 ± 9%, P < .05) with higher wall motion score indices (2.01 ± 0.39 vs 1.76 ± 0.29, P < .05). Wall motion scores in the anteroseptum, anterior wall, and apex were significantly higher in the symmetric group, while wall motion scores in the posterior and inferior walls were higher in the asymmetric group. Despite higher EFs and less extensive wall motion abnormalities, the asymmetric group had a greater percentage of severe MR assessed by integrative method (68% vs 40%, P = .03). However, the differences in VC width (0.72 ± 0.18 vs 0.63 ± 0.20 cm) and EROA (0.36 ± 0.16 vs 0.30 ± 0.15 cm 2 ) between the two groups were not significantly different ( P = .11 for both). EDV and ESV were smaller in the asymmetric group, but these differences were not statistically significant.
Variable | Asymmetric ( n = 22) | Symmetric ( n = 40) |
---|---|---|
Age (y) | 66 ± 10 | 68 ± 11 |
Men | 65% | 68% |
Body surface area (m 2 ) | 1.9 ± 0.2 | 2.0 ± 0.3 |
Left atrial diameter (cm) | 4.8 ± 0.7 | 5.0 ± 1.0 |
Left ventricle | ||
EDV (mL) | 184.7 ± 63.3 | 209.5 ± 78.4 |
ESV (mL) | 106.6 ± 45.9 | 135.1 ± 65.7 |
EF (%) | 44 ± 9 | 37 ± 11 ∗ |
Sphericity | 0.56 ± 0.09 | 0.58 ± 0.09 |
Wall motion score index | 1.76 ± 0.29 | 2.01 ± 0.39 ∗ |
MR severity | ||
Severe MR | 68% | 40% ∗ |
VC width (cm) | 0.72 ± 0.18 | 0.63 ± 0.20 |
EROA (cm 2 ) | 0.36 ± 0.16 | 0.30 ± 0.15 |
Ratio of jet area to left atrial area | 0.37 ± 0.10 | 0.35 ± 0.11 |
Assessment of Mitral Valve Geometry by 3D TEE
Significant differences in mitral valve geometry were found between the asymmetric and symmetric tethering patterns ( Table 2 , Figures 3 and 4 ). Compared with the symmetric group, the asymmetric group had significantly greater mitral annular heights and smaller CC diameters. Ratios of height to CC diameter were therefore higher in the asymmetric group. The asymmetric group had significantly greater posterior leaflet angles, smaller anterior leaflet angles, and smaller posterior leaflet closure areas. Ratios of posterior to anterior leaflet tethering angle in the asymmetric group were significantly higher (3.19 ± 0.88 vs 1.95 ± 0.46, P < .01; Figure 4 ). Additionally, ratios of anterior to posterior leaflet closure area were greater in the asymmetric group (2.04 ± 0.37 vs 1.4 ± 0.24, P < .01; Figure 4 ), suggesting a posterior movement of the line of coaptation. Total tenting volumes were greater in the symmetric group (2.6 ± 1.6 vs 4.4 ± 2.9 mL, P < .01; Figure 4 ). Absolute tenting volume as a measure of overall tethering degree was weakly correlated with MR severity (VC width: r = 0.26, P = .04; EROA: r = 0.32, P = .01). The correlation between tenting volume and VC width was better in the symmetric group ( r = 0.39, P = .01) than in the asymmetric group ( r = 0.27, P = .23).
Variable | Asymmetric ( n = 22) | Symmetric ( n = 40) |
---|---|---|
Mitral annulus | ||
CC diameter (mm) | 37.7 ± 3.2 | 40.7 ± 4.5 † |
AP diameter (mm) | 35.7 ± 4.5 | 37.0 ± 4.9 |
Height (mm) | 10.3 ± 1.9 | 8.5 ± 1.9 † |
Ratio of height to CC diameter | 0.27 ± 0.05 | 0.21 ± 0.05 † |
Circumference (mm) | 127 ± 12 | 133 ± 15 |
Area (cm 2 ) | 10.8 ± 2.0 | 12.3 ± 2.9 ∗ |
Mitral leaflets | ||
AML angle (°) | 19 ± 5 | 24 ± 5 † |
PML angle (°) | 58 ± 12 | 45 ± 9 † |
AML closure area (cm 2 ) | 8.9 ± 1.9 | 9.0 ± 2.5 |
PML closure area (cm 2 ) | 4.5 ± 1.2 | 6.5 ± 1.8 † |
Leaflet closure area (cm 2 ) | 13.5 ± 2.8 | 15.5 ± 4.1 ∗ |
AML tenting volume (mL) | 1.8 ± 1.3 | 3.0 ± 2.1 ∗ |
PML tenting volume(mL) | 0.8 ± 0.4 | 1.6 ± 0.8 † |
Tenting volume (mL) | 2.6 ± 1.6 | 4.4 ± 2.9 † |
Tenting height (mm) | 7.7 ± 2.6 | 9.3 ± 3.3 ∗ |
Aortic-mitral angle (°) | 113 ± 10 | 116 ± 9.0 |
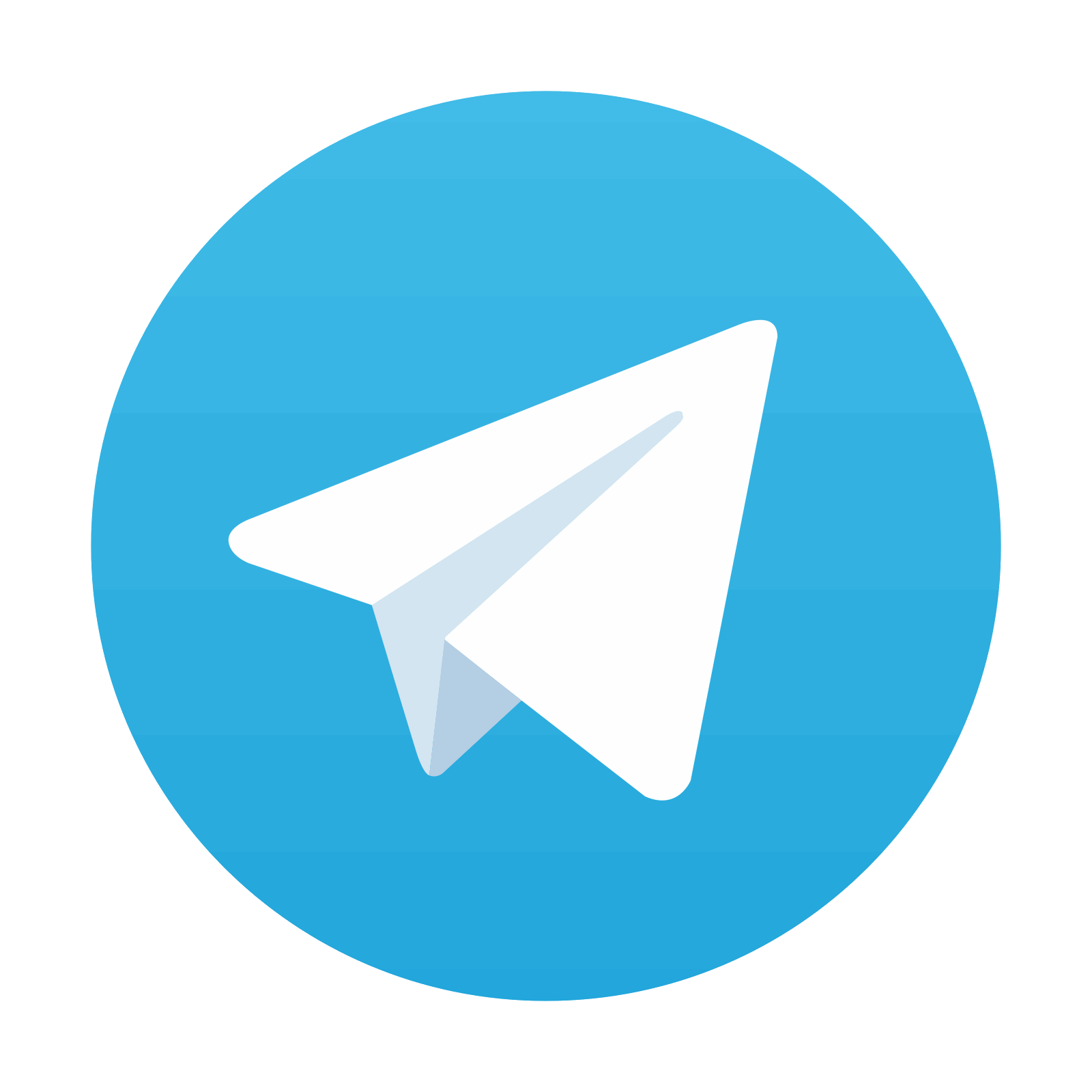
Stay updated, free articles. Join our Telegram channel

Full access? Get Clinical Tree
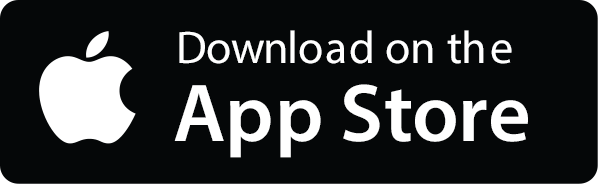
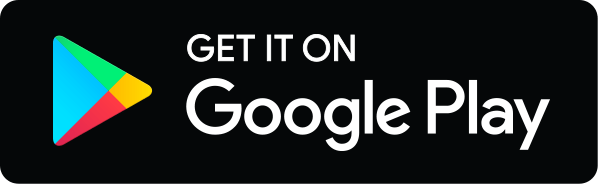
