Overlap between difficult-to-control and severe asthma
14.2.1 Asthma Masqueraders
Asthma masqueraders and comorbidities: differential diagnosis of asthma
Upper respiratory tract |
allergic rhinitis/chronic rhinitis |
sinusitis |
adenoid/tonsillar hypertrophy |
nasal foreign body |
Middle respiratory tract |
Laryngo−/tracheo−/bronchomalacia |
pertussis |
structural lesions, including stenosis and vascular ring |
vocal cord dysfunction |
tracheoesophageal fistula |
foreign body aspiration |
Lower respiratory tract |
bronchopulmonary dysplasia |
GERD |
bronchiectasis cystic fibrosis (CF)-related bronchiectasis non-CF bronchiectasis |
14.2.2 Comorbid Conditions
In addition to rhinosinusitis and GERD, common comorbid conditions that worsen asthma symptoms in both children and adults include obesity and sleep-disordered breathing (Table 14.1). These comorbidities, briefly mentioned for consideration in children with severe asthma, are extensively covered in Chap. 4.
Rhinitis
The physiologic processes of the nasal and lung airways are similar and linked in asthma [19, 20]. Accordingly, allergic rhinitis is directly associated with severe asthma, is an independent predictor of poor asthma control, and impacts pulmonary function recovery following an exacerbation [21–24]. In the multicenter NIH-/NIAID-sponsored study, Asthma Phenotypes in the Inner City (APIC), rhinitis was assessed and managed as per the Allergic Rhinitis and Its Impact on Asthma (ARIA) guidelines [25], and rhinitis severity and phenotypes were characterized based on physical exam, allergy testing and longitudinal assessment of symptoms, and rhinitis controller medication usage over 1 year [26]. In this study, a strong relationship was observed between rhinitis severity and asthma severity in inner-city children with asthma (standardized direct effect, 0.52; p < 0.001) [24], and rhinitis severity at baseline was a strong predictor of difficult-to-control asthma [23]. Further, of the two severe childhood asthma phenotypes identified and distinguished by being “highly atopic” versus “low-atopic/nonatopic” in APIC, rhinitis was severe in the highly atopic phenotype [27].
Other studies have also strongly related allergic rhinitis to asthma severity and poor outcomes. Allergic rhinitis and eczema often predate and predict the onset of childhood asthma, disease progression recognized as the “Atopic March” [28]. In the European Union-sponsored Mechanisms of the Development of ALLergy (MeDALL) study , asthma with eczema, rhinitis, and multiple allergen sensitizations formed a multi-morbid allergy phenotype predictive of clinical severity and persistence of disease [29]. Improved allergic rhinitis control is associated with good asthma control [30]. Therefore, diagnosis and management of allergic rhinitis in children with high asthma morbidity is a key component of improved asthma control.
Obesity
Childhood obesity is an independent risk factor for asthma [31, 32]. Obese children have higher disease severity, poor asthma control, and suboptimal response to medications [33–35]. Although mechanistic links between pediatric asthma and obesity are still being investigated, there is evidence to suggest that truncal adiposity and its associated complications, systemic inflammation, and metabolic abnormalities are associated with pulmonary function deficits [36–38]. These obesity-related complications are more prevalent in nonatopic children [39]. Systemic inflammation in obese asthmatic children is also nonatopic, skewed to a T-helper 1 phenotype relative to normal-weight children, with paucity of airway luminal inflammation [37, 40, 41]. These immune differences between obese and normal-weight asthmatic children likely underlie their reduced response to inhaled corticosteroids [42]. In light of these observations, obese asthmatic children who are not responsive to its management may benefit from an evaluation with an asthma specialist in conjunction with an endocrinology evaluation and management of obesity-mediated metabolic abnormalities with weight reduction strategies to decrease asthma burden [43].
Gastroesophageal reflux (GERD)
Although there is some controversy regarding the role of GERD in asthma [44–47], GERD is frequently observed among children with asthma and is associated with poor control [44], and GERD management is associated with improved asthma control [45, 48]. An American Lung Association-sponsored randomized controlled trial with lansoprazole (PPI) in children with poorly controlled asthma did not demonstrate significant improvement in symptoms or lung function [49]. In contrast, a bronchoscopy-based study of adults with severe asthma found that evidence of GERD represented one of the three main features of severe asthma, and definitively treating GERD in these patients improved their asthma [50]. Thus, we recommend, for children with severe asthma, an initial evaluation for symptoms to be supported by investigations such as pH probe or impedance study and consideration of a therapeutic trial with antacids (proton pump inhibitors) for 6–8 weeks and possibly bronchoscopy with lavage and upper airway characterization for indicators of GERD [51].
Sleep-disordered breathing (SDB)
SDB, of which obstructive sleep apnea (OSA) is the most common form, can contribute to poor asthma control [52, 53]. Although the mechanisms underlying this association are not known, the role of inflammation and that of altered airway architecture are currently being investigated. Given that obesity is comorbid with asthma, GERD, and SDB, mechanistic links between obesity, asthma, and SDB need further investigation. Similar to obesity, at this time, clinicians should proactively query for SDB in children with asthma [54] and address it as a comorbid condition to facilitate improved asthma control.
14.2.3 Poor Adherence
When asthma diagnosis is correct and not severe due to comorbidities, the main determinant of difficult-to-control asthma can be poor adherence to asthma management and especially asthma controller therapy. Poor adherence can be due to a variety of factors including forgetting, competing demands [55], perceived medication adverse reactions [56], attitudes and beliefs about medications and asthma itself, mental health conditions in child and/or parent/caregiver, and incorrect medication use, reasons that overlap with those in adults (discussed in Chap. 3) [57, 58]. Conventional approaches to quantify adherence such as personal recall and pharmacy fill/refill data have inherent limitations [59]. Asthma controller medication dose counters can accurately account for controller medication use. In a seminal study of daily controller inhaled corticosteroid (ICS) monitoring of children with persistent asthma for 13 weeks, self-reported ICS usage by daily diary cards was median 95% of the prescribed; median actual ICS usage by dose counter was 58% of the prescribed, and in children experiencing an asthma exacerbation, median ICS adherence was 14% [60]. Today, asthma medication adherence monitoring has advanced to real-time cloud-based electronic monitoring of MDI/DPI usage of both reliever (e.g., albuterol MDI) [61, 62] and controller asthma medications [63]. Early studies combining electronic real-time reliever tracking with an asthma management enhancer (e.g., physician/nurse notification, digital app self-management) indicate their potential to improve outcomes [61, 62]. Until electronic monitoring becomes mainstream, practitioners may consider evaluating medication adherence from pharmacy refill data, which may be more accurate than personal report. In addition to adherence, clinicians should ensure correct technique for medication use by educating and re-evaluating correct technique and use of valved holding chambers and breath-actuated devices.
14.3 Severe Asthma in Children Is a Heterogeneous Condition
Severe asthma persists despite correct diagnosis, management of comorbidities, and good medication adherence. Investigations into phenotypes and determinants have found that severe childhood asthma is heterogeneous [64], much like that observed in adults (Chap. 7) with some distinctions from severe asthma in adults. For instance, analysis of the NHLBI-sponsored SARP III revealed that children with severe asthma had a higher proportion of males and African Americans but were not distinguished by obesity, airflow obstruction, atopic sensitization, or markers of inflammation —measures that distinguished adults with severe versus non-severe asthma [65]. This dissimilarity in distinguishing features in severe asthma in children when compared with adults indicates a need to characterize asthma phenotypes specifically in children to enable severe disease management via phenotype-directed care [66]. The APIC study has made inroads into defining some phenotypes of severe asthma in children.
14.3.1 Asthma Phenotypes in the Inner City (APIC) Study
The seminal APIC study [23] investigated asthma phenotypes and severity determinants of difficult-to-control asthma in 717 inner-city children aged 6–17 (65% black and 28% Hispanic) living in nine US urban communities and in the context of National Asthma Education and Prevention Program (NAEPP) guidelines-based care. As detailed above, study participants received evaluation and management visits every 2 months over an entire year during which standardized algorithms were used to optimize asthma management based upon NAEPP guidelines and rhinitis management based upon ARIA guidelines. The contribution of nonadherence to disease severity was mitigated by providing controller and reliever medications during the study. Three different analytic approaches of the APIC data to date have characterized asthma severity and identified its main determinants and phenotypic features among urban children, those who have the highest disease burden.
- 1.
Severe asthma phenotypes in inner-city children: Using “clustering” as an unbiased analytic approach, Zoratti et al. categorized APIC children into five different asthma phenotypes based on clinical characteristics and pulmonary function testing (PFTs) [27]. Asthma severity, the presence and degree of atopy, nasal symptoms, and pulmonary function distinguished the five phenotypes (Fig. 14.2). Two groups, each representing 15% of the cohort, were relatively severe. The first severe group had the greatest levels of asthma severity and exacerbations and was highly atopic (median 14 of 22 positive skin tests and median sIgE of 616kU/L) with severe rhinitis and abnormal PFTs. The second severe group was highly symptomatic for asthma despite high doses of controller medications, but had low to no atopy.
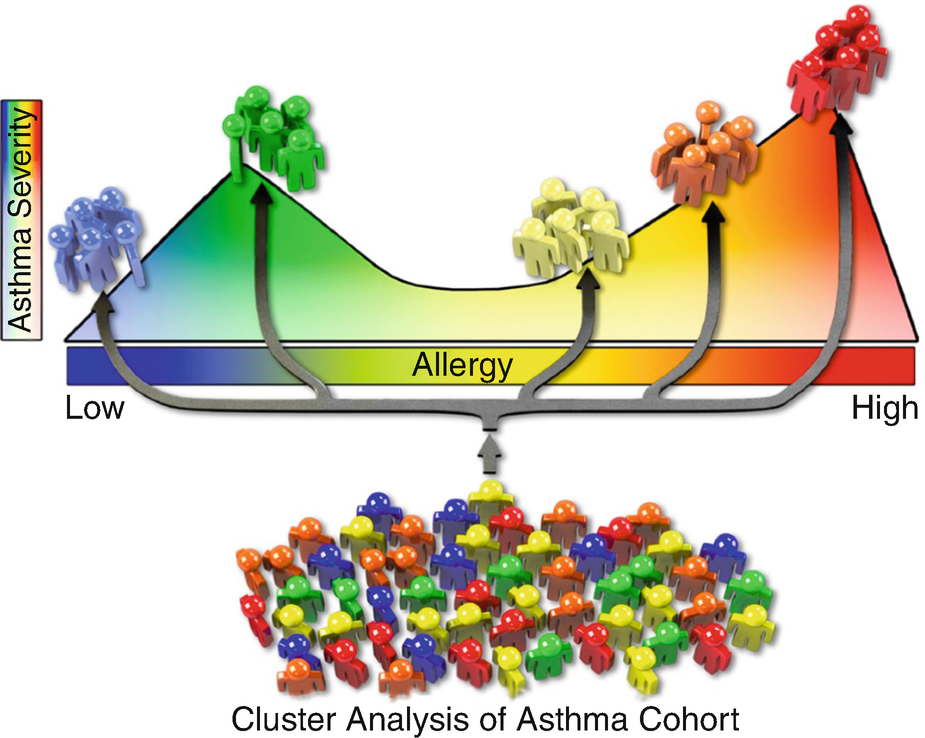
Asthma phenotypes in APIC children
The origins of these severe asthma phenotypes may begin in early childhood. In a cluster analysis of a high-risk urban cohort of infants at high risk for asthma, the highly atopic/ exacerbation-prone with lung function deficits and low-atopic/nonatopic, highly symptomatic phenotypes could be distinguished from transient wheezers and disease remitters at age 4 [67]. Further, these phenotypes were predictive of asthma severity, exacerbations, lung function deficits, and further intensification of inhalant allergen sensitization, total IgE, and FeNO at ages 6–7. Therefore, while the intensity of atopy is an important distinguishing feature of severe childhood asthma with origins in early life, these studies identified an additional phenotype of severe childhood asthma that was nearly dissociated from atopy.
- 2.
Characteristics and endotype of difficult-to-control asthma: Defined by the need for daily controller therapy of at least 500 mcg inhaled fluticasone daily with or without a long-acting beta-agonist (“difficult-to-control”) versus 100 mcg or less of fluticasone (“easy-to-control”) through 1 year of asthma management, Pongracic et al. [23] found that 41% of APIC children had difficult-to-control and 37% had easy-to-control asthma. Although adherence to controllers did not differ between groups, difficult-to-control asthma was characterized by high exacerbation rates, especially in the spring and fall; greater daytime and nocturnal symptoms, especially in the fall and winter; and impaired lung function over the year despite ongoing high-dose controller therapy. At baseline evaluation, spirometric (FEV1) bronchodilator responsiveness, Asthma Control Test score, rhinitis severity, and atopy were all predictive features of subsequent difficult-to-control asthma. Hence, for clinical practice, we suggest routine inclusion of these measures for early identification of children with difficult-to-control asthma. Brown et al. evaluated the endotype of difficult-to-control asthma in the African American subset of APIC children (n = 235) via peripheral blood cytokine and inflammatory cell profiles [68]. Relative to easy-to-control asthmatics, those who were difficult-to-control had higher peripheral blood eosinophil and neutrophil counts and higher IL-5-, IFN-γ-, and TH17-associated cytokines (CXCL-1, IL-17A, and IL-8), supporting a role of systemic immune dysregulation in severe/difficult-to-control asthma [68].
- 3.
Key clinical factors contributing to asthma severity: To address the need for an integrated understanding of key factors underlying asthma severity, Liu et al. used published medical evidence to test a conceptual diagram of how eight key factors are linked to asthma severity in APIC children: atopic sensitization, atopic inflammation, pulmonary physiology, stress, obesity, vitamin D, environmental tobacco smoke (ETS) exposure, and rhinitis severity [24]. Asthma severity was characterized by a 1-year composite assessment of day and night symptoms, exacerbations, and controller usage. The researchers found their conceptual diagram explained 53.4% of the asthma severity observed in these children (Fig. 14.3). Moreover, the atopic pathway and the ETS exposure pathway were two main and distinct contributors to asthma severity. These pathways affected asthma mostly through their effects on lung function and the nose, such that pulmonary physiology and rhinitis severity had the largest effects on asthma severity, followed by ETS exposure and allergic inflammation. This diagrammatic understanding of the key factors underlying asthma severity and their relative contributions can be used to prioritize interventions to reduce disease severity and personalize asthma management.
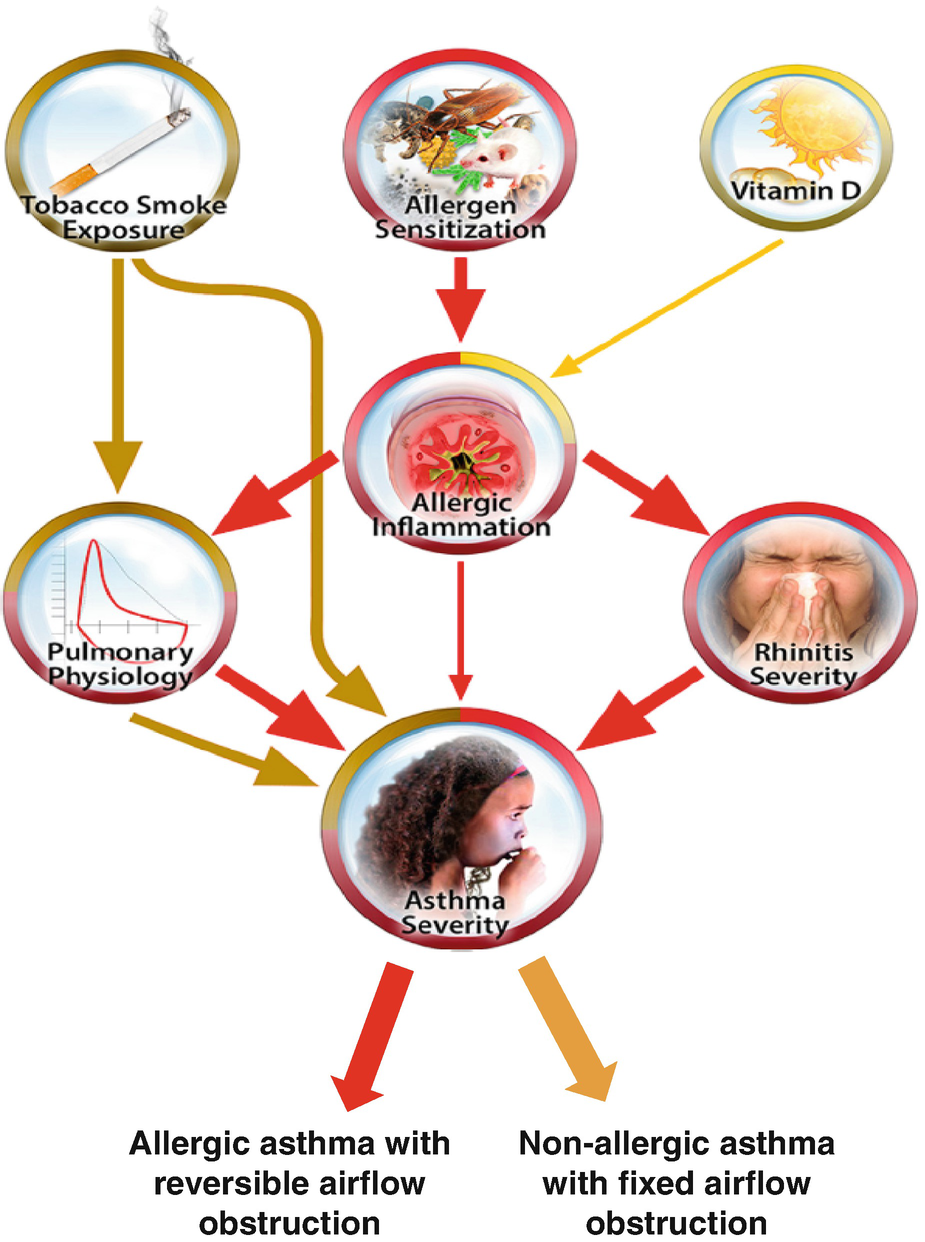
Contribution of environmental exposures to asthma severity
14.4 Determinants of Severe Asthma in Childhood
Asthma is a multifactorial disease with contributions from genetic susceptibility and environmental exposures. Since severe asthma in children may present as exacerbation-prone disease, persistent poor control of symptoms, or low lung function, there is a need for an improved understanding of the contribution of the known asthma determinants to these different manifestations of severe asthma. Here, we review recent literature which links known determinants with one or more of these aspects of severe asthma.
14.4.1 Genetic Susceptibility
Genetic variation influences asthma severity by affecting responses to environmental exposures and therapy [69, 70]. Genome-wide association studies have linked asthma susceptibility with variants in immune response and oxidative stress genes, such as IL12B [71], PDE4D [72], DENND1B [73], GSDMB, IL33, RAD50, IL1RL1 [74], and GSMT1 [75]. Further, variants in CDHR3, FCER2, and GLCCI1 genes are linked to the severity of asthma exacerbations [74, 76, 77]. The Genetics of Asthma in Latino Americans (GALA) and Study of African Americans, Asthma, Genes, and Environment (SAGE) studies have also highlighted the contribution of ethnicity or genetic admixture to severe asthma defined by low lung function [78]. To date, the ORMDL3 gene on chromosome 17q21 has been most consistently linked with asthma, with variants found in different ethnic groups [79, 80]. Despite this evidence on the genetic contribution to asthma severity, genetic testing for asthmatic children has not reached clinical practice.
14.4.2 Environmental Exposures
14.4.2.1 Respiratory Viruses
Several types of viral infections are linked with severe asthma, including severe exacerbations [81]. Rhinovirus infection is a well-known and extensively characterized trigger of asthma exacerbations [82]. Respiratory syncytial virus (RSV) can cause both initiation of airway reactivity, particularly in premature babies, and higher asthma severity [83, 84]. Influenza virus can cause secondary respiratory complications including pneumonia, which independently contribute to asthma severity [85–87].
Recently, much has been learned about the pathobiology of rhinoviruses and asthma exacerbations [88]. Of particular interest is CDHR3, which serves as the epithelial receptor for human rhinovirus type C. Allelic variation in CDHR3 increases cell surface expression of CDHR3 and RV-C infectivity [89] and is associated with severe asthma exacerbations in children [74]. Biologic agents, including anti-IgE therapy, have served as a probe to investigate impaired/dysregulated immune responses to rhinovirus. Through the NIH-/NIAID-sponsored Preventative Omalizumab or Step-up Therapy for Severe Fall Exacerbations (PROSE) study , effects of omalizumab on rhinovirus infection and associated asthma exacerbations were studied in nearly 500 children with exacerbation-prone asthma [90]. Children receiving omalizumab had decreased duration of rhinovirus infection, reduced peak rhinovirus shedding, and decreased frequency of rhinovirus illnesses [91]. Gill et al. [92] performed ex vivo assays on peripheral blood mononuclear cells and purified plasmacytoid dendritic cells from a subset of these children and found that generation of rhinovirus-induced IFN-α in the presence of IgE cross-linking was significantly increased in the post-omalizumab treatment group, especially in those who did not have an exacerbation. Thus, a plausible explanation emerged that explained the clinical effectiveness of omalizumab by restoring antiviral immune responses to rhinovirus.
14.4.2.2 Indoor Environment
Home Environment
Exposures Associated with Allergic Responses
Common asthma triggers
Viral illness |
Rhinovirus, RSV, adenovirus, influenza, parainfluenza |
Allergens (when sensitized) |
Indoor (home and school exposure) |
Pets (furred, feathered) |
Roaches, mice, dust mite |
Molds |
Outdoor |
Tree, grass, weed pollen, seasonal molds |
Airway irritants |
Tobacco smoke/marijuana |
Aerosols/fragrances |
Traffic-related air pollution: PM10, PM2.5, ozone, SO2, NO2 |
Woodburning stoves |
Exposures Associated with Non-allergic Responses
Exposure to indoor airborne irritants, particularly environmental tobacco smoke (ETS), has been associated with both asthma onset and poor control (Table 14.2 ) [99, 100]. Pathway analysis of APIC participants found that passive smoke exposure was associated with severe asthma with frequent symptoms via a distinct, nonatopic pathway (Fig. 14.3). It is important to highlight that self-reported tobacco exposure is less sensitive than objectively measured nicotine biomarkers for predicting exacerbations [101], with as many as 70% of children having positive testing for urine and salivary cotinine in families who claimed no smoke exposure in their residence. Hence, utilizing objective measures of ETS exposure such as cotinine may be a better predictor of the effect of tobacco smoke on exacerbations [101]. ETS may also contribute to asthma susceptibility since children, particularly boys, exposed to passive smoke in utero have smaller cross-sectional area of airways which contributes to more severe asthma exacerbations in early life [102]. Even low levels of ongoing smoke exposure during childhood are associated with increased airway inflammatory markers in children and reduced lung function [103, 104]. Since the severity of early exacerbations has been found to be an independent contributor to airway remodeling, we deduce that passive smoke exposure early on and then smoking among the subset of teens with asthma is one modifiable environmental exposure to decrease burden of severe asthma in children. Unfortunately, evidence for the effectiveness of counseling interventions aimed at reducing environmental tobacco smoke exposure in kids is lacking [105]; however, there is some evidence that legislation to ban indoor smoking in public places may be associated with a decrease in asthma exacerbations on a population level [106].
Marijuana smoking and e-cigarette usage have been rapidly rising in teenage children [107]. Although their effects on asthma severity are not well understood, there are reasons for concern. E-cigarettes contain numerous respirable toxicants relevant to asthma and are associated with epithelial, innate immune, neutrophilic, and mucous secretion responses similar to those seen in cigarette smokers in some regards, but also unique in others [108–110]. Marijuana smoking is associated with cough, sputum, and wheeze in adolescents and young adults and resolves with smoking cessation [111]. Moreover, marijuana allergens have been identified by allergy skin testing and serum allergen-specific IgE and associated with anaphylaxis [112].
School Environment: School as Workplace for Children
Since children spend the largest part of their waking hours in school, one may equate schools for children to workplaces for adults (Chap. 5).The NIAID-sponsored School Inner-City Asthma Study (SICAS), an invaluable epidemiologic, prospective study, demonstrates the impact of exposures in elementary public schools on asthma severity in US urban children. Important SICAS findings include the association of classroom nitrogen dioxide levels with airflow obstruction [113] and mouse allergen with increased odds of having daytime symptoms and lower lung function (FEV1) [114]. Based on these observations, Phipatanakul and colleagues are investigating the effect of a multilevel school exposure mitigation in reducing asthma severity in school children [115]. A pilot trial of classroom-based air cleaners demonstrated significant reductions in classroom fine particulate matter (PM2.5) and black carbon, supporting their use in the ongoing school intervention trial [116].
14.4.2.3 Outdoor Environment
In addition to the well-understood contribution of pollen as an atopic outdoor trigger [117], several outdoor irritants including coal smoke, diesel exhaust particles (DEPs), sulfur dioxide, and nitrogen dioxide are linked with incident asthma, exacerbations, and diminished lung function [118–120]. This is likely due to their influence on airway inflammation [121]. DEPs induce Th17 cells and increase IL-17 [121]. Similarly, high ambient ozone levels are associated with asthma morbidity, likely by causing oxidant-driven injury to the airways [122, 123]. Accordingly, national and international guidelines strongly state the importance of evaluating and addressing environmental triggers that can make asthma worse and trigger exacerbations; for example, in the NAEPP guidelines, identifying and addressing indoor allergens in sensitized individuals are considered Evidence Category A, and tobacco smoke, wood burning fireplaces, and strong odors are considered Evidence Category C [124, 125].
14.5 Management of Severe Asthma in Childhood (Fig. 14.4)
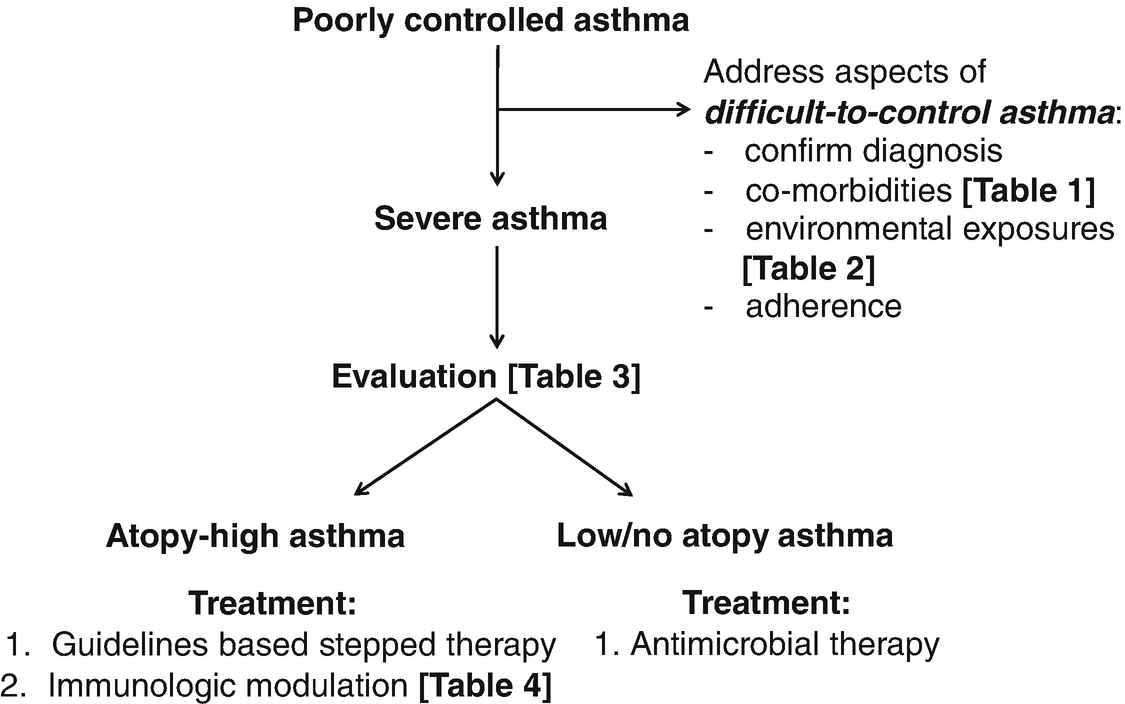
Approach to severe asthma management in children
Evaluation of severe asthma in children
Questionnaires |
Asthma severity |
NAEPP (https://www.nhlbi.nih.gov/files/docs/guidelines/asthma_qrg.pdf) |
Asthma control |
NAEPP (https://www.nhlbi.nih.gov/files/docs/guidelines/asthma_qrg.pdf) |
Asthma control test (ACT) (https://www.asthmacontroltest.com) |
Asthma control questionnaire (ACQ) (http://www.qoltech.co.uk/index.htm) |
Asthma therapy assessment questionnaire (ATAQ) (https://www.nhlbi.nih.gov/files/docs/guidelines/asthma.pdf) |
Pulmonary function |
Spirometry, including bronchodilator responsiveness and longitudinal follow-up |
Lung volumes (plethysmography or nitrogen washout) |
Bronchoprovocation: |
Methacholine challenge |
Exercise testing |
Cold air challenge |
Immune measures for evaluation of highly atopic phenotype |
Complete blood count for eosinophilia |
Total IgE |
Specific IgE or allergy skin testing to assess inhalant allergen sensitization |
Fractional exhaled nitric oxide (FeNO) |
Composite approach |
Composite asthma severity index (CASI): Includes symptoms, FEV1, controller treatment step, and exacerbations |
Seasonal asthma exacerbation index (SAEI): Includes eosinophils, IgE, allergen sensitization, FEV1/FVC ratio, FeNO, age, treatment step, and exacerbation in the previous 3 months |
Imaging |
Chest x-ray/airway fluoroscopy/HR CT scan (visualization for anatomical abnormalities (masqueraders (Table 14.1)) |
Bronchoscopy |
Airway visualization for anatomical abnormalities (masqueraders (Table 14.1)) |
Bronchoalveolar lavage for cytology (lipid laden macrophages for GERD, eosinophilia) and microbes (bacteria, fungi) |
Endobronchial biopsy (evaluation of submucosal cytology and airway smooth muscle) |
14.5.1 Evaluation
Questionnaires
Standardized questionnaires are the cornerstone for asthma control classification [126]. Asthma control assessment questionnaires are endorsed in NAEPP and GINA guidelines for standard use in clinical practice [124, 126]. The ACT/Childhood ACT (www.asthmacontroltest.com) is a good example of a simplified questionnaire that is self-administered by design [127–130]. Studies show the ACT/Childhood ACT in children with asthma to be predictive of difficult-to-control asthma [23] and modestly predictive of asthma exacerbations [131, 132]. In a Kaiser health system study, an ACT score <= 15, indicating very poor control, increased the 12-month risk of emergency hospital care for asthma [130]. Additional questionnaires to assess control include the Asthma Control Questionnaire (ACQ) [133] and Asthma Therapy Assessment Questionnaire (ATAQ) [134, 135].
Pulmonary function testing
Spirometry measures of lung function are endorsed in NAEPP and GINA guidelines as routine in asthma assessments in clinical practice [124, 126]. Spirometry parameters including FEV1, FVC, FEV1/FVC ratio, and FEF25–75 have been associated with exacerbation risk [136–138]. FEV1 < 60% predicted is a predictor of exacerbation risk in the following 4 months [139]. Severe asthma phenotypes have persistently low FEV1/FVC ratio, poorly responsive to guidelines-based management [23]. In particular, spirometry identifies exacerbation-prone asthmatics who are insensitive to symptoms and otherwise would not be detected as being inadequately controlled until asthma worsening is severe. For these severe, exacerbation-prone, symptom-insensitive asthmatics, home peak flows or spirometry may be particularly helpful as an early indicator and warning sign of worsening asthma.
Inhaled bronchodilator response, assessed by change in FEV1, is diagnostic of asthma [140] and a strong predictor of difficult-to-control asthma in children [23]. Air trapping and airway closure due to asthma that can be inferred by (a) testing for lung volumes by plethysmography or nitrogen washout or (b) improvement in lung volumes (e.g., FVC) to bronchodilator are commonly found in severe, exacerbation-prone asthmatic children [141, 142].
Longitudinal follow-up of spirometry (i.e., at least annually) identifies children with asthma who have reduced lung function growth and/or progressive rate of decline in lung function during childhood that has lifelong consequences [13, 143]. Exacerbation-prone asthmatics may be especially at risk since exacerbations have been linked to lung function decline [9–12].
Bronchoprovocation testing can also be helpful in the assessment of severe asthmatic children; for example, graded challenge to methacholine can objectively quantify bronchial hyperresponsiveness (BHR) in asthmatic children to support the diagnosis of asthma [144], assess improvement in BHR to therapy [145], and predict future exacerbations [146, 147]. Exercise challenge testing can also identify airway reactivity not evident on spirometry [144]. While exercise challenge testing can be viewed as a “real-world” challenge that is logistically feasible to administer, some caution is warranted in administering this test to severe asthmatic children since it is not a graded dose-response challenge and may provoke a severe obstruction.
Inflammatory biomarkers and phenotyping
Biomarkers such as fractional exhaled nitric oxide (FeNO) are also potential asthma exacerbation predictors. Increases in the slope of daily FeNO measurements have been observed in the time immediately preceding an asthma exacerbation [148]; however, data on predictive ability of FeNO alone in managing children have been mixed [149–151]. Elevated peripheral blood eosinophils (e.g., >300–400 cells/μL) have been shown to be predictive in some evaluations of both adults and children [137, 150–152]. Patterns and extent of atopic sensitization and total serum IgE contribute to asthma phenotyping and are highly elevated in the major severe, exacerbation-prone phenotype of highly atopic asthma in children [27]. Importantly, in the evaluation of children with severe asthma, high levels of FeNO, blood eosinophils, total IgE, and number of allergen sensitizations are indicators of the highly atopic immunophenotype of severe asthma that refine therapeutic management in a phenotype-directed manner for better outcomes (Fig. 14.4).
Putting these measures together
Standardized composite scoring indices that incorporate key asthma evaluation variables provide an effective and useful way to evaluate asthma severity, assess response to therapies, and predict exacerbation risk [137, 150, 151, 153]. The NIH-/NIAID-sponsored Composite Asthma Severity Index (CASI) exemplifies a composite scoring index with features that make it useful and adoptable. CASI incorporates NAEPP standard evaluation components—day and night symptoms, albuterol usage, controller step-level medication usage, lung function, and exacerbations—into a single composite score [126, 154]. CASI has been validated, a minimally important difference and threshold for greater than mild asthma has been established, and pdf forms and a simple online calculator are in the public domain (http://www.asthmaseverity.org/)—practically ready for widespread use [154, 155]. In children, an NIH-/NIAID-sponsored Seasonal Asthma Exacerbation Index (SAEI) has combined many relevant predictors mentioned above to optimize their utility as a single composite score to predict exacerbations [150, 151]. SAEI incorporates exacerbation risk factors into a single risk score: eosinophils, IgE, allergen skin test positivity, FEV1/FVC ratio, FeNO, age, treatment step, and exacerbation in the previous 3 months. In two studies, high SAEI scores were associated with increased exacerbation rates in each of the four seasons. Although such composite indices need further testing for predictive accuracy, it is evident that composite analyses of relevant and standard evaluation variables noted above will improve evaluation and provide better clinical prediction and decision-making.
Role of imaging
At this time, imaging in asthmatics primarily serves to evaluate for masqueraders and comorbidities (Table 14.1) [156]. A chest x-ray in children may identify congenital malformations and overt bronchiectasis, and lateral decubitus films may suggest a foreign body, while airway imaging, including fluoroscopy, frequently identifies large airway disease including laryngomalacia and tracheomalacia as well as congenital lesions, such as vascular ring or stenosis. In addition to identifying airway and parenchymal diseases (e.g., bronchiectasis), high-resolution CT scans are increasingly being used to study heterogeneity of ventilation, which has been found to be a feature of severe asthma [156, 157].
Role of bronchoscopy
There is increased recognition for a role of bronchoscopy in the evaluation of severe asthmatics [16, 50]. Direct airway visualization identifies masqueraders and comorbidities such as tracheomalacia [17], foreign body, and proximal GERD [50]. Analysis of the bronchoalveolar lavage can reveal aspiration, altered cellular composition (e.g., eosinophilia, neutrophilia), and infection with bacteria, viruses, and fungi (e.g., allergic bronchopulmonary mycoses) that could underlie asthma severity and would otherwise be missed [16]. Further, endobronchial biopsies can identify smooth muscle and mucous gland hypertrophy/hyperplasia, basement membrane thickening, and epithelial injury characteristic of severe asthma in children [158]. Saglani and colleagues have reported on the role of the airway smooth muscle in airway remodeling, which likely contributes to persistent lung function deficits in severe asthma [159].
14.5.2 Management
14.5.2.1 Preventing Exacerbations
Asthma exacerbations are a target of asthma research and personalized care, which aims for rigorous prediction and prevention of asthma exacerbations to revolutionize the status quo. Having addressed the intuitive aspects of exacerbation prevention like interventions to remove environmental triggers in the immediate environment to the extent possible, a major reason for identifying patients at risk for an asthma exacerbation is to develop interventions to prevent exacerbations from occurring. A recent (within the last 3–12 months) severe asthma exacerbation is a relatively strong and consistent predictor of future exacerbations [134, 160–162], and a prior asthma hospitalization increases the likelihood of subsequent asthma hospitalizations [163]. Similarly, loss of asthma control in young children, especially daytime cough/wheeze and nighttime albuterol use, is a good predictor of next-day exacerbations [164]. Although exacerbation prevention is a major objective of asthma controller therapies, exacerbation-prone severe asthma in children requires enhanced management to prevent recurrent events.
It is also important to recognize that predictors of asthma exacerbations in children can differ from predictors of symptoms or control. For instance, in a post hoc study of children in the Childhood Asthma Management Program (CAMP) , not being treated with inhaled corticosteroids, lower FEV1/FVC ratio, and greater BHR to methacholine predicted poor control, while younger age, history of exacerbation in the prior year, >3 days of prednisone use in the past 3 months, and higher total eosinophil counts in addition to lower FEV1/FVC ratio and greater BHR to methacholine predicted exacerbations [147].
Traditional Guidelines-Based Management: Inhaled Corticosteroids (ICS) in Preventing Exacerbations and Achieving Control
As observed in adults (Chap. 6), ICS controller therapy remains a mainstay of treatment for the prevention of asthma exacerbations, with several studies demonstrating ~40% reduction in the rate of asthma exacerbations over the course of multiple years with daily ICS use [165–167]. Up to 24% of asthma exacerbations may be attributable to ICS nonadherence [168]. The addition of long-acting beta-agonist (LABA) to ICS controller therapy in fixed-dose combination inhalers may increase adherence to medication [169, 170] and contribute to improved outcomes in those treated with ICS-LABA combination controller medication [171]. Recently published studies have well demonstrated the safety of ICS-LABA combination in adults [172] and children [173], leading to the removal of the FDA “black box warning” for these medications (https://www.fda.gov/Drugs/DrugSafety/ucm589587.htm).
Determining medication adherence, as detailed in Sect. 14.2 above, is key to determining treatment failure when making decisions about stepped up care. Since self-reported adherence significantly overestimates true levels of controller adherence [174], monitoring of FeNO may be one method to assess ICS controller adherence [175, 176]. In addition, new technologies such as text messaging of medication reminders based digital ascertainment of ICS usage is being currently investigated [61, 62] [177, 178]. Objective methods of adherence monitoring can better distinguish true treatment failure and classify patients into those who are adherent and controlled, adherent but not controlled, or nonadherent and not controlled for a differentiated approach to management.
Recent studies have demonstrated, in exacerbation-prone children with asthma, that increasing ICS provides little to no benefit to reducing exacerbation risk. In a NIH-/NIAID-sponsored study of well-managed children with exacerbation-prone asthma, doubling ICS did not reduce the risk of fall seasonal exacerbations [90]. In an NIH-/NHLBI-sponsored study of children with exacerbation-prone asthma, quintupling ICS dose for 7 days at the onset of asthma worsening did not reduce exacerbation risk over a year [179]. Hence, we suggest that adjunctive management approaches be considered for children with exacerbation-prone asthma.
One such approach is the use of macrolide antibiotics. Infection or colonization with atypical bacteria such as Mycoplasma and Chlamydia has been identified in asthmatic airways and associated with both atopic and nonatopic airway inflammation [180–182]. Children with refractory asthma can have chlamydia in their lower airways, along with neutrophilia and elevated IL-8 levels [183, 184]. These infections are difficult to diagnose and are typically identified in bronchoscopic specimens using PCR-based techniques. In preschool children with severe exacerbations, treatment with azithromycin has been associated with decreased exacerbation duration [185], severity [186] and frequency [187], and reduction in nasal IL-8 levels [187]. Although not mainstream for severe asthma evaluations in children, we propose that bronchoscopic evaluation be considered, especially in those with low-atopic/nonatopic phenotype, to evaluate for a number of treatable causes of severe asthma described above, including atypical bacterial infection with neutrophilia [184].
Immunomodulatory Biologic Therapies
Biologic therapies for children with severe asthma
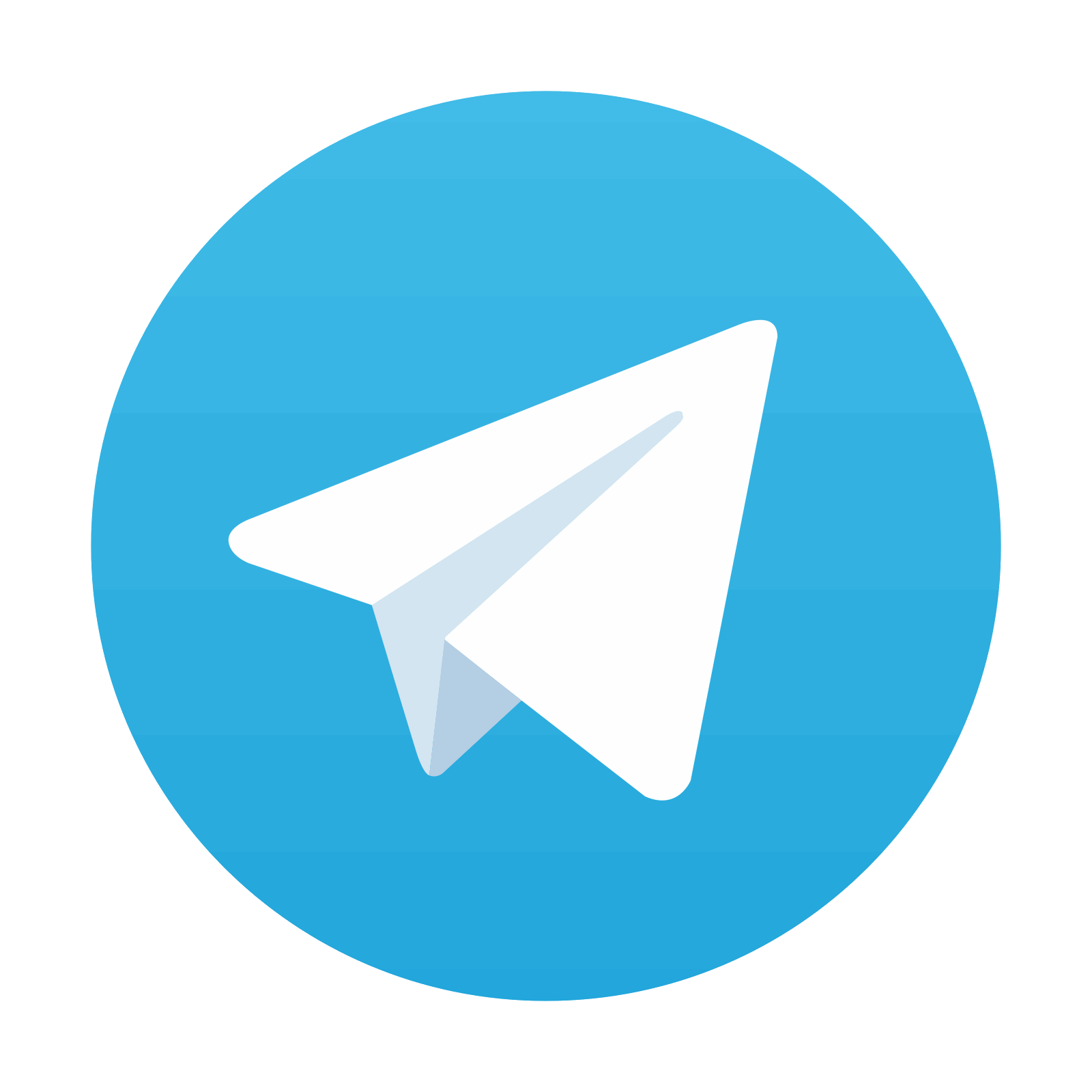
Stay updated, free articles. Join our Telegram channel

Full access? Get Clinical Tree
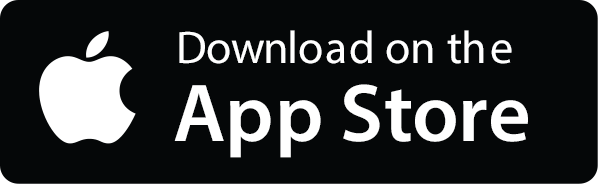
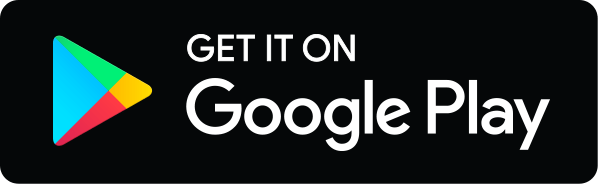