Summary
Postoperative impairment of right ventricular (RV) systolic function can appear after surgical repair of complex congenital heart defects, such as tetralogy of Fallot; it is caused by chronic volume and/or pressure overload due to pulmonary regurgitation and/or stenosis. RV dysfunction is strongly associated with prognosis in these patients. Cardiac magnetic resonance imaging is the gold standard for quantification of RV volumes and ejection fraction in patients with congenital heart diseases; however, it is costly and is not widely available. Echocardiography is the imaging modality that is most available and most frequently used to assess RV systolic function. However, RV ejection fraction cannot be measured accurately by standard two-dimensional echocardiography because of its pyramidal shape. Surrogate parameters of RV systolic function are mostly used in routine practice. New techniques of two-dimensional strain and three-dimensional quantification of RV volumes and ejection fraction have been developed in recent years. The aim of this article is to show the pertinence of each variable of RV systolic function measured by echocardiography in patients with repaired congenital heart disease and residual chronic RV overload.
Résumé
Une dysfonction ventriculaire droite peut apparaître après réparation des cardiopathies cono-ventriculaires dont la plus fréquente est la tétralogie de Fallot. Elle est secondaire à une surcharge volumétrique et/ou barométrique causée par une fuite et/ou une sténose pulmonaire chronique. La dysfonction ventriculaire droite est un critère pronostique fort dans cette population. L’imagerie par résonance magnétique est l’actuel gold standard pour l’évaluation des volumes et de la fonction ventriculaire droite dans les cardiopathies congénitales. Cependant, il s’agit d’un examen coûteux et peu disponible. L’échocardiographie est la modalité d’imagerie la plus utilisée en routine pour évaluer la fonction du ventricule droit. Toutefois, à cause de sa géométrie pyramidale, les volumes et la fraction d’éjection du ventricule droit ne peuvent pas être calculés de manière fiable par les techniques standard d’échographie deux dimensions. Des paramètres de fonction systolique régionale sont le plus souvent utilisés. De nouvelles techniques de déformation myocardique et de quantification des volumes et de la fraction d’éjection en échographie trois dimensions ont été développées. L’objectif de cet article est de montrer la pertinence de chaque paramètre de fonction systolique du ventricule droit mesuré en échocardiographie chez des patients ayant eu une réparation d’une cardiopathie congénitale et qui ont une surcharge chronique du ventricule droit.
Introduction
In patients with repaired congenital heart disease (CHD), residual lesions can lead to impairment of right ventricular (RV) systolic function. Treatment of residual lesions is necessary to prevent irreversible dysfunction, which is associated with poorer prognosis . Detection of RV impairment is important in order to find the best timing for intervention.
Assessment of RV systolic function by non-invasive methods is challenging because of its complex shape, contraction pattern and response to overload, which differs from that of the better-known left ventricle (LV). Good knowledge of RV physiopathology in this particular condition is required to provide a pertinent assessment of RV systolic function.
RV physiopathology
RV shape
The shape of the right ventricle (RV) is complex: viewed from the front, it appears triangular; viewed in transverse section, it looks like a crescent. RV shape is also determined by the position of the interventricular septum, which, under normal conditions, is concave towards the LV in both systole and diastole . Under pathological conditions, it can be flat or reversed in systole or diastole or both, leading to dynamic changes in its geometry. The RV is composed of three parts: the inlet with the tricuspid valve, the trabeculated chamber and the outlet with the infudibulum and the pulmonary valve . Because of the particular shape of the RV, these three parts cannot be seen simultaneously in one cross-sectional view. Different RV views must be obtained to visualize all RV segments .
RV muscular architecture
The RV is composed of two myofibre layers : a superficial layer where the fibre arrangement is circumferential (the fibres in this layer are in continuity with the left ventricular [LV] superficial layer); and a subendocardial layer, with longitudinal arrangement of the fibres from the base to the apex. In addition, there are three muscular bands: parietal, septomarginal and moderator. The RV wall is six times thinner than the LV wall, which has an additional midwall layer with circumferentially orientated fibres .
RV normal contraction pattern
RV contraction is mainly longitudinal, with RV shortening from the inlet towards the apex and from the apex towards the outlet . LV participates in 20% to 30% of RV contractile performance with the motion of the shared superficial fibres . The free wall also contracts radially toward the septum, but the radial contraction contribution to RV output is minor .
Normal RV loading conditions
RV afterload is represented by the pulmonary circulation, characterized by low resistance and impedance. RV isovolumic times are very short, as RV pressure rapidly exceed pulmonary artery end-diastolic pressure and decreases progressively during ejection.
RV response to overload
RV tolerates volume overload better than pressure overload because of its thinner wall, which makes it more distensible than the LV , but less capable of hypertrophy.
In chronic volume overload, the RV dilates; its function declines after sustained overload, and is associated with LV impairment as the displacement of the septum alters the LV filling and contraction pattern .
In chronic pressure overload, the RV first hypertrophies in order to normalize the wall stress. The main fibres that hypertrophy are circumferentially orientated. The RV contraction pattern becomes predominantly radial and circumferential, resembling the LV contraction pattern . The capacity of the RV to hypertrophy is limited, however; its mass is normally one sixth of the LV . Ultimately RV dilates, with impairment of its function.
Post-operative changes in RV shape and contraction in CHD
After surgical repair of complex CHDs, such as tetralogy of Fallot, pulmonary atresia and truncus arteriosus, residual lesions are common because of an absent or hypoplastic pulmonary valve.
When the pulmonary valve is severely hypoplastic, the surgeon relieves the RV outflow tract obstruction with a transannular patch. Pulmonary regurgitation is a consequence of this surgical technique, and is well tolerated over several years. The RV dilates progressively, depending on the regurgitation severity. Ultimately, pulmonary regurgitation leads to RV dysfunction. Pulmonary valve replacement must be done before irreversible RV dysfunction occurs. Monitoring RV function is thus important in the follow-up of patients with pulmonary regurgitation, to assess the best timing for pulmonary valve replacement.
In pulmonary atresia and truncus arteriosus, surgical repair can necessitate the use of a conduit between the RV and the pulmonary artery. Pulmonary stenosis (PS) develops during growth because of the inadequate size of the conduit and calcifications; it leads to RV hypertrophy, with predominance of a transverse contraction pattern compared with normal or dilated RV, where longitudinal fibres are predominant . Pulmonary regurgitation can also occur because of the lack or incompetence of the pulmonary valve in the conduit. In this case, RV has a mixed overload, and is both dilated and hypertrophied. Impairment of RV function can also occur, depending on the severity of pulmonary stenosis and pulmonary regurgitation.
Post-operative changes in RV shape
The shape of the RV is dramatically modified by surgical repair of CHD, with infundibular bulging and apical dilation and deformation, leading to a large range of RV shapes . Moreover, pericardial section and suture during surgery influence RV geometry, as RV is normally more constrained by the pericardium then the LV because of its thinner wall .
RV geometry and contraction pattern in repaired CHD vary according to the type of surgery and overload. These changes can affect the reliability of the variables measured by echocardiography. The limits and advantages of each technique must be known to provide a relevant assessment of RV systolic function.
Surrogate variables of RV systolic function
Because of its complex shape, there is no geometrical assumption that can allow quantification of RV volumes and ejection fraction (RVEF) by standard two-dimensional (2D) echocardiography. For this reason, surrogate parameters of RV systolic function are used most frequently to assess RV systolic function, because they are easy to measure, feasible and reproducible.
Tricuspid annulus movement
Measurements of tricuspid annulus movement by M-mode (tricuspid annular plane systolic excursion [TAPSE]) or tissue Doppler imaging (peak systolic velocity [PSV]) are used most frequently to assess RV function; they are highly feasible and reproducible. However, several studies have shown their dependence on loading condition; TAPSE and PSV values are increased in volume overload and decreased in pressure overload , independent of RVEF. Moreover, in patients who underwent cardiac surgery, values of TAPSE and PSV were systematically reduced, contrasting with normal RVEF. Tamborini et al. found that TAPSE and PSV values were lower after mitral valve repair (normal RV loading condition), whereas RVEF remained unchanged . In adults with repaired tetralogy of Fallot, several studies showed similar results, with weak or no correlation between TAPSE or PSV and RVEF measured by magnetic resonance imaging (MRI) . TAPSE and PSV are thus not reliable measurements of RV systolic function after cardiac surgery.
2D global longitudinal peak systolic strain of the RV lateral wall
Speckle-tracking echocardiography is a new technology that allows quantification of myocardial regional deformation. The main advantage compared with tissue Doppler imaging is its angle independency; it was also thought to be less load dependent, but further studies demonstrated that 2D longitudinal strain values increase in volume overload and decrease in barometric overload .
In patients with repaired CHD, its interpretation is even more complex: RV global longitudinal peak systolic strain (GLPSS) is decreased in repaired CHD with pulmonary regurgitation and normal RVEF . Bonnemains et al. found no correlation between RV longitudinal strain and RVEF in patients with repaired tetralogy of Fallot . Pulmonary regurgitation is a volume overload that should increase the GLPSS value. Several hypotheses have been advanced to explain this result, such as impairment of RV longitudinal function and an increase in transverse function after cardiac surgery . Another hypothesis is that the degree of RV dilation is responsible for the decrease in RV GLPSS; indeed, GLPSS is correlated with RV dilation in this population . Two clinical studies corroborated this hypothesis, as they found improvement in RV GLPSS after pulmonary valve implantation . In repaired CHD with pulmonary stenosis or a combination of pulmonary stenosis and pulmonary regurgitation, RV hypertrophy leads to the development of more circumferentially orientated fibres, and a switch of contraction pattern from predominantly longitudinal (as in the normal RV) to predominantly circumferential (as in the normal LV) . This probably explains why different studies performed in this population showed contrasting results.
The complex interpretation and variability of reported normative values for 2D GLPSS explain why it is not yet ready for clinical use in this population.
Isovolumic acceleration time
Myocardial acceleration during isovolumic contraction is defined as the peak isovolumic myocardial velocity divided by time to peak velocity; it is derived from tissue Doppler imaging of the lateral tricuspid annulus. Isovolumic acceleration time (IVA) is a quantitative assessment of RV contractile function that is supposed to be unaffected by RV geometry or loading conditions. In patients with tetralogy of Fallot, studies have shown a good correlation between pulmonary regurgitation severity and IVA . However, few data are available on the reproducibility of this measurement. Furthermore, IVA varies with age and heart rate. For these reasons, it is difficult to interpret changes in IVA during serial clinical evaluation of patients with repaired CHD.
Myocardial performance index
Myocardial performance index (MPI) is another tissue Doppler-derived parameter of RV systolic function. MPI is calculated using the following formula: MPI = (isovolumic contraction time + isovolumic relaxation time)/ejection time. Eidem et al. showed that MPI was independent of loading conditions: its value didn’t differ between children with PS, children with RV volume overload (atrial septal defect) and controls . Furthermore, they showed that MPI was unchanged after closure of ASD . In patients with repaired tetralogy of Fallot, Schwerzmann et al. found a negative linear correlation between MPI and MRI-derived RVEF ( r = 0.73). MPI values of 0.4 were indicative of an RVEF < 35%, and when MPI values were < 0.25, they were predictive of an RVEF ≥ 50% .
The major limitation of this Doppler-derived variable is its angle dependence. Furthermore, MPI index calculation takes into account isovolumic relaxation time, which is shortened when RV filling pressures are high, lowering MPI values independent of RVEF . Interpretation of MPI must be cautious in repaired CHD patients with an RV restrictive pattern. In adults with repaired CHD, a restrictive pattern is frequent, which may explain the contradictory results of Selly et al., who found no correlation between MPI and RVEF in adults with repaired tetralogy of Fallot .
Fractional area change
Fractional area change (FAC) is the percentage change in RV diastolic and systolic area measured in the apical four-chamber view: FAC = 100 × ([RV end-diastolic area – RV end-systolic area]/RV end-diastolic area). FAC is a simple measure of RV systolic function, but it includes only the body and apex of the RV, and not the RV outflow tract (which is often dilated in repaired CHD). FAC necessitates endocardial border delineation , and is thus less reproducible and feasible than the previously described M-mode or Doppler-derived measurements, especially when RV is hypertrophied and in patients with a poor apical window. FAC has been shown to correlate well with RVEF measured by MRI in the general population . However, in patients with repaired CHD, FAC correlates less with MRI-derived RVEF because of regional RV remodelling, especially in the RV outflow tract region, which is not included in the apical four-chamber view; in patients with repaired tetralogy of Fallot, Selly et al. found a fair correlation of FAC with RVEF ( r = 0.70) .
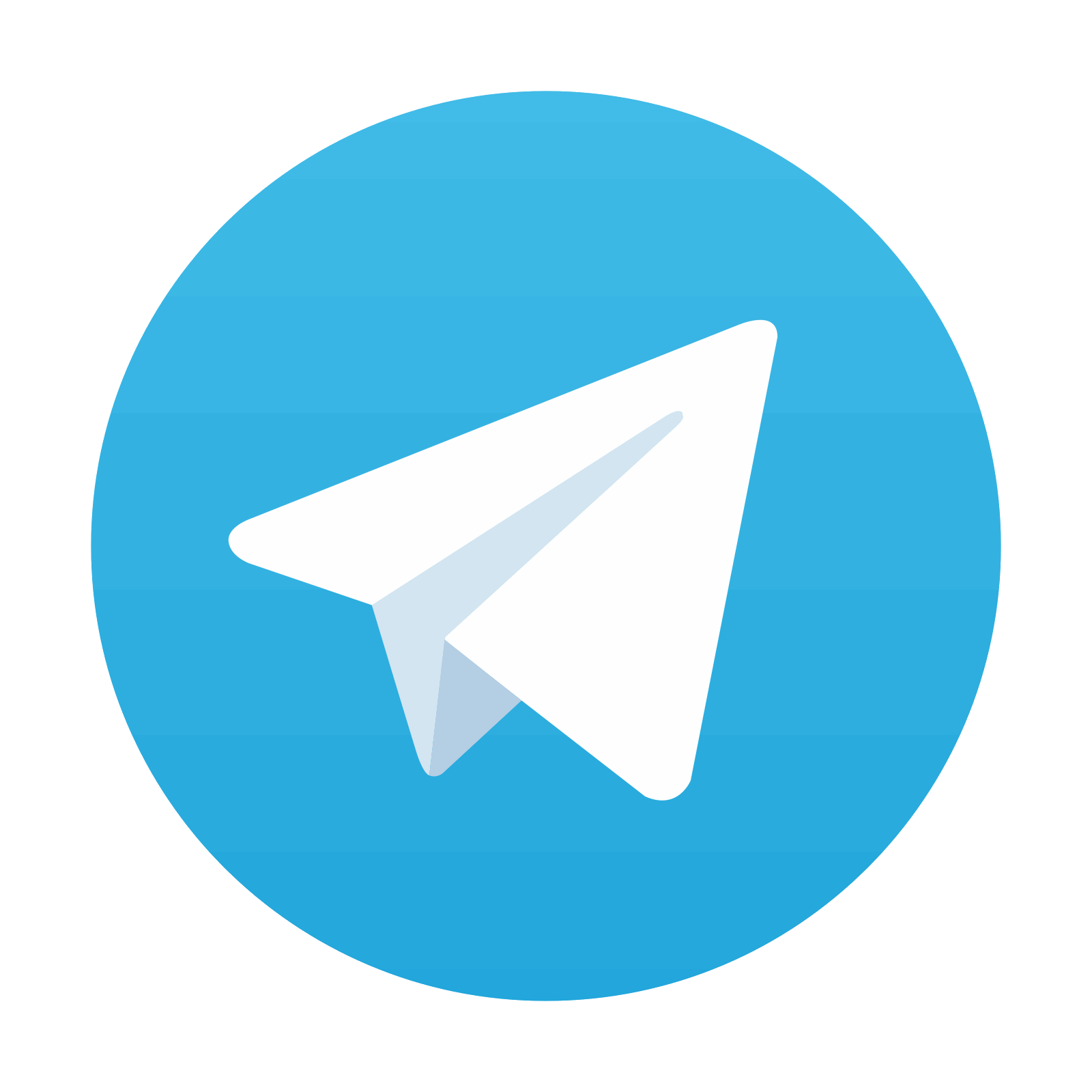
Stay updated, free articles. Join our Telegram channel

Full access? Get Clinical Tree
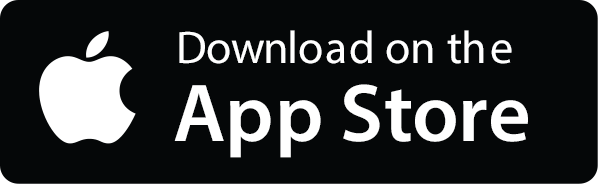
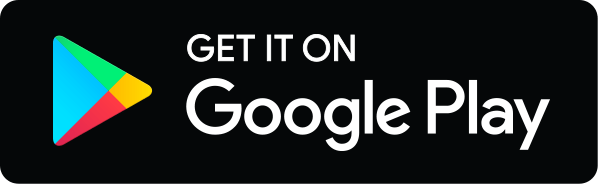