Assessment of Regional Ventricular Function
Lori B. Heller
Solomon Aronson
Assessment of global and regional ventricular function has become the cornerstone for evaluating patients with ischemic heart disease. The dynamic assessment of regional ventricular function is based on echocardiographic derived indices of muscle contraction and relaxation, which are obtained from analysis of moving objects. Echocardiography is therefore an inherently qualitative technique. When using transesophageal echocardiography (TEE) to evaluate regional ventricular function one is really attempting to make inferences about a three-dimensional structure from two-dimensional images. Therefore, multiple images must be acquired from multiple planes and assumptions (explicitly or implicitly) must be made about the shape of the ventricle and the coronary artery distribution within the ventricle.
SEGMENTAL MODEL OF THE LEFT VENTRICLE
Dividing the LV into segments allows more accurate description of the location of the regional wall motion abnormalities (RWMA) detected with echocardiography and is necessary for their correlation with coronary artery anatomy. Models developed during the era of single plane TEE are based on the transgastric midpapillary short-axis view of the LV and divide the midpapillary image of the LV into four equal segments: septal, anterior, lateral, and inferior. Although simple, there is no true correlation with coronary artery anatomy and the base and the apex are not taken into account. The Society of Cardiovascular Anesthesiology and American Society of Echocardiography has recommended a 16-segment model for regional LV assessment, dividing the basal and mid levels into six segments and the apex into four (Fig. 12.1). An advantage of using this segmental model is a common standard and terminology in discussions about regional function of the LV.
Regional function assessment of the LV can be accomplished quickly and easily in most patients using multiplane TEE by obtaining five standard views of the LV, three from the midesophageal window and two from the transgastric window (Fig. 12.1).
The first step in obtaining the midesophageal views of the LV is to position the transducer posterior to the left atrium (LA) at the mid level of the mitral valve. The imaging plane is then oriented to simultaneously pass through the center of the mitral annulus and the apex of the LV. In many patients, the esophagus is lateral to this point and the tip of the probe must be flexed to the right to position the transducer directly posterior to the center of the mitral annulus. The LV is usually oriented with its apex somewhat more inferior to the base and the tip of the probe must next be retroflexed to direct the imaging plane through the apex. Getting the proper amount of retroflexion is best done by rotating to multiplane angle 90 degrees and then retroflexing until the apex of the LV is pointing straight down in the image display. The depth should be adjusted to include the entire LV (usually 16 cm). Rotating to multiplane angle 0 degrees should keep the center of the mitral annulus and LV apex in view. The midesophageal four-chamber view is now obtained by rotating the multiplane angle forward from 0 degrees until the aortic valve is no longer in view and the diameter of the tricuspid annulus is maximized, usually between 10 and 30 degrees. The midesophageal four-chamber view shows all three segments (basal, mid, and apical) in each of the septal and lateral walls (Figs. 12.2 and 12.3). The midesophageal two-chamber view can next be obtained by rotating the multiplane angle forward until the right atrium (RA) and the right ventricle (RV) disappear, usually between 90 and 110 degrees. The midesophageal two-chamber view of the LV shows the three segments in each of the anterior and inferior walls (Figs. 12.4 and 12.5). Finally, the midesophageal long-axis view is developed by rotating the multiplane angle forward until the LV outflow tract, aortic valve, and the proximal ascending aorta come into view, usually between 120 and 160 degrees (Figs. 12.6 and 12.7). This view shows the basal and midanteroseptal segments,
as well as the basal and midposterior segments. Therefore, with the imaging plane properly oriented through the center of the mitral annulus and the LV apex, one can examine the entire LV without moving the probe and simply rotating the multiplane angle from 0 to 180 degrees.
as well as the basal and midposterior segments. Therefore, with the imaging plane properly oriented through the center of the mitral annulus and the LV apex, one can examine the entire LV without moving the probe and simply rotating the multiplane angle from 0 to 180 degrees.
![]() FIGURE 12.1. Sixteen-segment model for regional left ventricular assessment. The basal and mid levels are each divided into six segments and the apex into four. |
![]() FIGURE 12.2. Four-chamber view (obtained at 0-10 degrees) showing the basal, mid, and apical portions of the septal and lateral walls (left and right, respectively). |
![]() FIGURE 12.4. Two-chamber view (obtained at 90-110 degrees) showing the basal, mid, and apical portions of the inferior and anterior walls (left and right, respectively). |
![]() FIGURE 12.6. Long-axis view of the left ventricle (obtained at 120-160 degrees) showing the basal, mid, and apical portions of the posterior and anteroseptal walls (left and right, respectively). |
![]() FIGURE 12.8. Posterior and anteroseptal walls of the left ventricle according to the 16- segment model. |
The transgastric views of the LV are acquired by advancing the probe into the stomach and anteflexing the tip until the heart comes into view. At multiplane angle 0 degrees a short axis of the LV should appear and the probe is then turned to the right or left as needed to center the LV in the display. The depth should be adjusted to include the entire LV, usually 12 cm (Figs. 12.8 and 12.9). There are three levels of transgastric views: basal, where the mitral valve is seen; mid, at the level of the papillary muscles; and apical. These transverse short-axis views have the advantage of simultaneously showing portions of the LV supplied by the right, circumflex, and the left anterior descending coronary arteries (Figs. 12.10 and 12.11). The midpapillary level, which reveals the approximate center of the left ventricular cavity, is used to determine information regarding the cardiac function and volume status of the patient. Withdrawing the probe from the midpapillary view until the mitral apparatus develops the basal transgastric short-axis view (Fig. 12.11). In some patients, advancing the probe from the mid position develops the apical transgastric short-axis view, but in many the probe moves away from the heart and the image is lost.
The transgastric two-chamber view is developed from the basal transgastric short-axis view by rotating the multiplane angle forward until the apex and the mitral annulus come into view, usually close to 90 degrees (Figs. 12.12 and 12.15). The probe should be turned to the left or right as needed to open up the LV chamber, maximizing its size in the image. This view usually shows the basal and mid segments of the inferior walls, but not the apex.
When assessing wall motion, it is important to recognize two artifacts of imaging that can occur with any real-time tomographic imaging technique, such as echocardiography: foreshortening and pseudothickening. Foreshortening occurs when the imaging plane is not correctly aligned along the axis of the chamber being examined, creating an image that is shorter than the true length. With TEE this most commonly occurs at the apex of the LV (Fig. 12.14). Pseudothickening occurs when the heart moves from side to side through the imaging plane, creating the illusion of a change in wall thickness (Fig. 12.15). In order to accurately assess wall motion and thickness, the imaging plane must be perpendicular to the region of the LV being examined.
In current clinical practice, analysis of LV segmental function is based on a qualitative visual assessment of the motion and/or thickening of a segment during systole. Regional wall motion is characterized by observing the movement of the endocardium during systole. As the myocardial oxygen supply-to-demand balance worsens, graded regional wall motion abnormalities progress from mild hypokinesia to severe hypokinesia, akinesia, and finally dyskinesia (1,2). The following qualitative grading
scale for wall motion has been used extensively in the intraoperative echocardiography literature and is recommended: 1 = normal, or the endocardium moves toward the center of the LV cavity during systole greater than 30%; 2 = mildly hypokinetic, or the endocardium moves toward the center of the LV cavity < 30%, but greater than 10% during systole; 3 = severely hypokinetic, or the endocardium moves toward the center of the LV cavity but < 10% during systole; 4 = akinetic, or he endocardium does not move or thicken; and 5 = dyskinetic, or the endocardium moves away from the center of the LV cavity during systole (Fig. 12.16 and 12.17). All 16 segments are examined by obtaining five cross-sectional views of the LV, three through the midesophageal window and two through the transgastric window.
scale for wall motion has been used extensively in the intraoperative echocardiography literature and is recommended: 1 = normal, or the endocardium moves toward the center of the LV cavity during systole greater than 30%; 2 = mildly hypokinetic, or the endocardium moves toward the center of the LV cavity < 30%, but greater than 10% during systole; 3 = severely hypokinetic, or the endocardium moves toward the center of the LV cavity but < 10% during systole; 4 = akinetic, or he endocardium does not move or thicken; and 5 = dyskinetic, or the endocardium moves away from the center of the LV cavity during systole (Fig. 12.16 and 12.17). All 16 segments are examined by obtaining five cross-sectional views of the LV, three through the midesophageal window and two through the transgastric window.
![]() FIGURE 12.14. Four-chamber view demonstrating foreshortening of the LV apex. Foreshortening occurs when the imaging plane is not correctly aligned along the axis of the chamber being examined. |
REGIONAL VENTRICULAR FUNCTION AND ISCHEMIA DETECTION
Although new onset of RWMA is the most common way of detecting myocardial ischemia using echocardiography, there are many other important echocardiographic features that are seen in ischemic heart disease. These include abnormal relaxation or diastolic dysfunction, mitral insufficiency with normal mitral valve anatomy, dilation of the ventricle, thin-walled myocardium, and papillary muscle dysfunction. The usefulness of echocardiography for ischemia detection should be based on all findings and not just limited to detection of RWMA. Nevertheless, the relationship of RWMA to ischemia has been compared to changes that occur with surface electrocardiography (ECG), pulmonary capillary wedge pressure (PCWP), and the onset of chest pain. As early as 1935 it was recognized by Tennet and Wiggers that acute myocardial ischemia results in abnormal inward motion and thickening of the affected myocardial region (2). Since then wall motion abnormalities have been shown to occur within seconds of inadequate blood flow or oxygen supply (3). These abnormal contraction patterns typically occur at the same time as regional lactate production (4,5).
The precise sequence of regional functional changes that occurs in the myocardium after interruption of flow has been studied in models of acute ischemia, including percutaneous transluminal coronary angioplasty (6,7,8). Ischemia of the heart produces a number of changes in mechanical and electrical activity. When ischemia starts, one of the first changes that occurs is abnormal relaxation
of the segment involved. This is followed by a decrease in the systolic function as evidenced by a decrease in wall thickening and decrease in inward motion of the ventricular walls. As the ischemia progresses, the myocardial segment involved will progress from decreased motion (hypokinetic) to the total absence of motion (akinesis). In its most severe form, the segment involved not only fails to contract but cannot withstand the increase in pressure in the ventricle and moves away from the segments that are contracting (dyskinetic). Systolic function is estimated qualitatively and is reflected echocardiographically by regional wall thickening and wall motion during systole. Systolic wall thickening can be calculated from the equation: PSWT = SWT – DWT/SWT × 100, where PSWT = percentage systolic wall thickening, SWT = end systolic wall thickness, and DWT = end diastolic wall thickness.
of the segment involved. This is followed by a decrease in the systolic function as evidenced by a decrease in wall thickening and decrease in inward motion of the ventricular walls. As the ischemia progresses, the myocardial segment involved will progress from decreased motion (hypokinetic) to the total absence of motion (akinesis). In its most severe form, the segment involved not only fails to contract but cannot withstand the increase in pressure in the ventricle and moves away from the segments that are contracting (dyskinetic). Systolic function is estimated qualitatively and is reflected echocardiographically by regional wall thickening and wall motion during systole. Systolic wall thickening can be calculated from the equation: PSWT = SWT – DWT/SWT × 100, where PSWT = percentage systolic wall thickening, SWT = end systolic wall thickness, and DWT = end diastolic wall thickness.
When evaluating regional wall changes, one must be aware of other factors that influence the movement of the heart as seen on echocardiography. In addition to the inward movement of the walls during systole, the heart is affected by translational and rotational movements. Respiration tends to displace the heart and is an example of translational movement. Translational movement also occurs with the cardiac cycle, for example, displacement of the apex during systole. In the long-axis views, the apex may become foreshortened due to its superior and medial movement. With foreshortening, what appears to be the tip of the heart or apex is really an oblique cut at a higher level and if this is not properly identified, RWMA of the apex can be completely missed. During contraction, the heart tends to rotate along its vertical axis. A way of differentiating rotational movement from regional wall motion is to establish an imaginary point of reference in the center of the ventricle, and observe the movement of the walls towards this central point. Rotational movement will be identified as displacement in a circular fashion in relation to this point instead of displacement towards it.
Clinical studies have indicated that abnormal changes in segmental wall motion occur earlier and are a more sensitive indicator of myocardial ischemia than the abnormal changes detected with an electrocardiogram (4,9,10,11,12) or pulmonary artery catheter (13,14,15). In one study (12), 30 patients undergoing PTCA were simultaneously monitored with twelve-lead electrocardiograms and echocardiography. All the patients had isolated obstructive lesions in their left anterior descending coronary arteries, stable angina, normal baseline electrocardiograms, normal baseline myocardial function with no prior history of infarction and no angiographic evidence of collateralization. In the study, all patients developed segmental wall motion abnormalities (SWMA) approximately 10 seconds after coronary artery occlusion and 27 of the 30 developed repolarization changes in their ECG at approximately 22 seconds.
Smith et al. (11) evaluated 50 patients at high risk for myocardial ischemia during peripheral vascular or cardiac surgery with transesophageal echocardiography (TEE), multilead ECG, and a twelve-lead electrocardiogram. In their study, six patients had repolarization changes diagnostic of ischemia while 24 had new evidence of SWMA. SWMA occurred minutes before ECG changes in three of these six patients and no ST-segment change occurred before or without new SWMA. Three patients who sustained intraoperative myocardial infarctions (MI) developed SWMA in the corresponding area of myocardium, which persisted until the end of surgery, but only one of these three had ischemic ST-segment changes intraoperatively.
The value of pulmonary capillary wedge pressure (PCWP) monitoring for ischemia has also been compared to changes in regional LV function assessed with transesophageal echocardiography. In one study, PCWP, twelve-lead ECG, and left ventricular wall motion were evaluated in 98 patients before coronary artery bypass grafting at predetermined intervals (13). Myocardial ischemia was diagnosed by TEE in 14 patients, and in 10 of the 14 patients ischemia was also detected by repolarization changes on the ECG, while an increase of at least 3 mm Hg in PCWP (an indicator for ischemia) was sensitive only 33% of the time with a positive predictive value of only 16%. Overall, most studies indicate that the sensitivity of wall motion analysis for the detection of myocardial ischemia is generally superior to electrocardiography or pulmonary capillary wedge pressure monitoring.
Although transesophageal echocardiography appears to have many advantages over traditional intraoperative monitors of myocardial ischemia, there remain potential limitations as well. There are various pitfalls that can be encountered in the analysis of wall motion (16). The septum in particular must be given special consideration with respect to wall motion and wall thickness assessment (16,17,18). The septum is composed of two parts, the lower muscular portion and the basal membranous portion. The basal septum does not exhibit the same degree of contraction as the lower muscular part. At the most superior basal portion, the septum is attached to the aortic outflow track. Its movement at this level is normally paradoxical during ventricular systole. The septum is also a unique region of the left ventricle, because it is a region of the right ventricle as well, and is, therefore, influenced by forces from both ventricles. In addition, sternotomy, pericardiotomy, and cardiopulmonary bypass (CPB) have been suggested to alter the translational and rotational motion of the heart within the chest that may cause changes in ventricular septal motion (18). Consequently, the exact imaging plane for wall motion assessment is
critical. The short-axis view of the left ventricle at the level of the midpapillary muscles is used to ensure constant internal landmarks as reference (anterolateral and posteromedial papillary muscles) and to ensure monitoring of the muscular septal region. It must be recognized that although myocardial blood flow from the coronary arteries is best represented at the short-axis, midpapillary muscle level, there may be other myocardial regions that are underperfused and not adequately represented in one echocardiographic imaging plane (19). One solution to this problem is to frequently reposition the probe to view other cross sections of the heart. Another potential problem of wall motion assessment is evaluation of the discoordinated contraction that occurs due to bundle branch block or ventricular pacing. In this situation, the system used to assess SWMA must compensate for global motion of the heart (usually done with a floating frame of reference) and evaluate not only regional endocardial wall motion but myocardial thickening as well.
critical. The short-axis view of the left ventricle at the level of the midpapillary muscles is used to ensure constant internal landmarks as reference (anterolateral and posteromedial papillary muscles) and to ensure monitoring of the muscular septal region. It must be recognized that although myocardial blood flow from the coronary arteries is best represented at the short-axis, midpapillary muscle level, there may be other myocardial regions that are underperfused and not adequately represented in one echocardiographic imaging plane (19). One solution to this problem is to frequently reposition the probe to view other cross sections of the heart. Another potential problem of wall motion assessment is evaluation of the discoordinated contraction that occurs due to bundle branch block or ventricular pacing. In this situation, the system used to assess SWMA must compensate for global motion of the heart (usually done with a floating frame of reference) and evaluate not only regional endocardial wall motion but myocardial thickening as well.
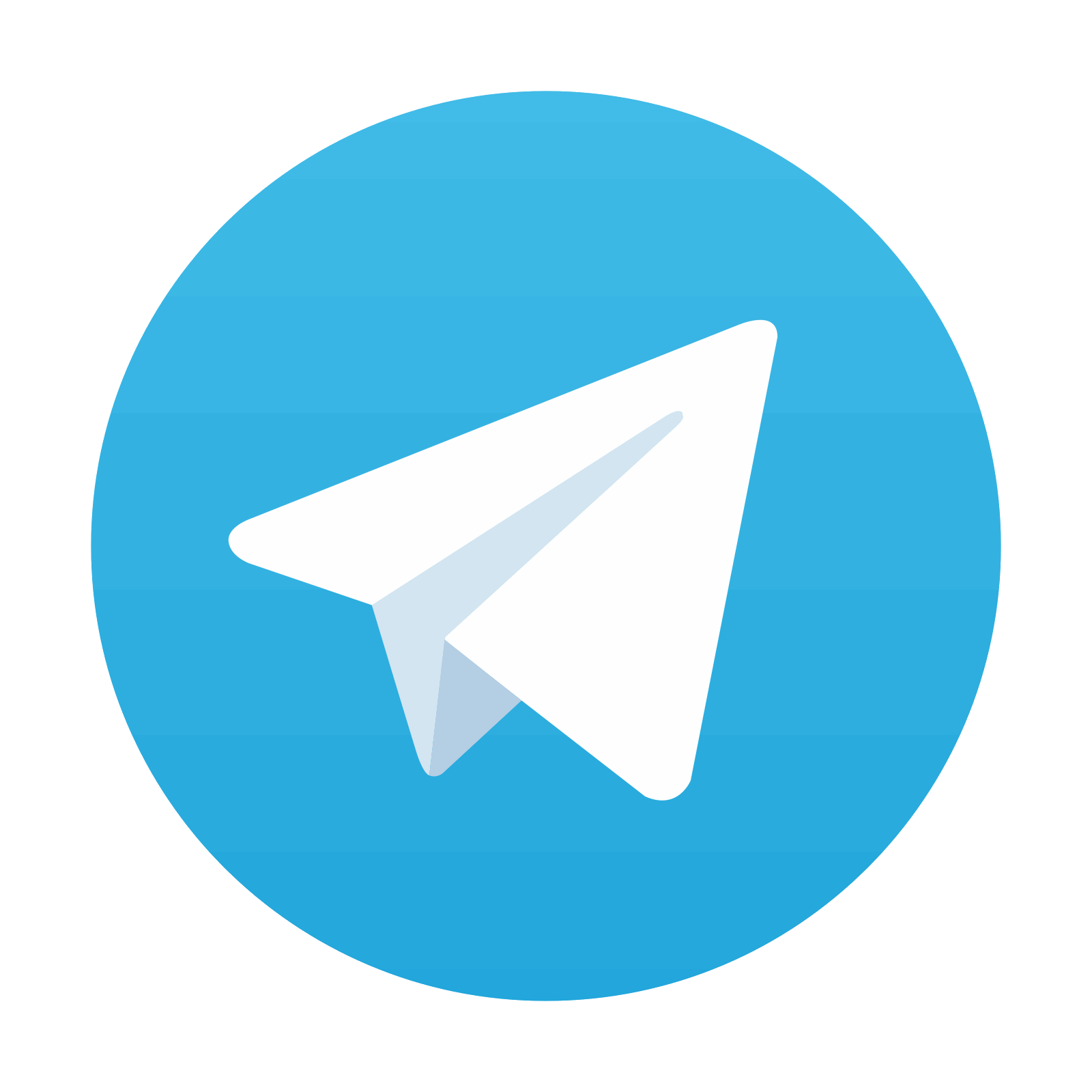
Stay updated, free articles. Join our Telegram channel

Full access? Get Clinical Tree
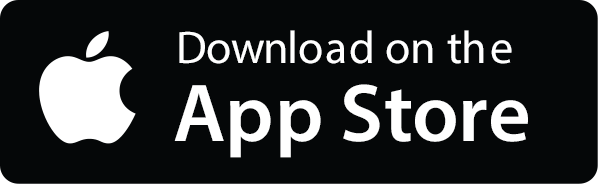
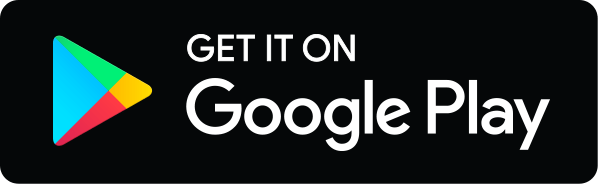