Assessment of Prosthetic Valves
Michelle Capdeville
Michael G. Licina
The first clinical artificial heart valve was introduced more than 45 years ago. Mechanical and biological prostheses developed in parallel. In 1953, Huffnagel treated aortic regurgitation by placing a caged ball valve in the descending thoracic aorta (1). In 1956, Murray implanted a cadaveric aortic valve in a similar fashion (2). With the subsequent development of cardiopulmonary bypass techniques, intracardiac valve replacement became possible, with Starr and Edwards performing the first successful valve replacement using a caged ball prosthesis in 1960 (3).
TYPES OF PROSTHETIC VALVES
Currently available prosthetic heart valves can be divided into two broad categories: mechanical and bioprosthetic. Multiple sizes are available for each valve type (Table 18.1). With prolonged implantation of a prosthetic valve, regardless of type, one can expect to see some degree of malfunction or failure, making this a form of palliative treatment (4,5).
TABLE 18.1. Prosthetic Valve Types | ||||||||||||||||||
---|---|---|---|---|---|---|---|---|---|---|---|---|---|---|---|---|---|---|
|
Mechanical Valves
The term “profile” is frequently used when describing prosthetic valves and refers to the height from the base of the prosthesis to the top of the struts.
High Profile Valves
Ball-Cage Valves
Ball-cage valves were the earliest mechanical valves used. The Starr-Edwards valve is the only ball-cage valve currently in use in the United States. Other modifications of this valve have included the Smeloff-Sutter valve and the Magovern-Cromie sutureless valve.
Starr-Edwards Valve
The Starr-Edwards prosthesis is the most well known caged ball valve, having the longest history and most extensive follow-up. It was designed in 1960 and consists of a round silastic ball and a metal cage (poppet or occluder) with a circular sewing ring. Over the years this valve has undergone constant modification to eliminate problems, such as thrombogenicity, noise, and hemolysis (Fig. 18.1) (6).
When blood flows across the valve, the ball moves to the apex of the cage; the ball moves to the base of the cage to close the valve and prevent regurgitation. The motion of the ball, with its continually changing points of contact, is thought to reduce the risk of thrombus formation. These valves have a high profile with the potential for left ventricular outflow tract obstruction in the mitral position. They also have a relatively small orifice and can cause some degree of hemolysis, though the latter is relatively insignificant in the absence of a perivalvular leak. Axisymmetrical and turbulent flow occurs because of the central obstruction by the ball and flow around its edges (flow downstream remains evenly distributed and converges). Stagnation can occur behind the ball, predisposing to thromboembolism. These valves have a very small regurgitant volume.
The Starr-Edwards valve has three orifices: a primary orifice at the annulus; a secondary orifice at the angle made by the ball in the open position and the annular opening; and a tertiary orifice between the ball perimeter and the ventricular or aortic walls. Therefore, a large primary orifice can result in a small tertiary orifice because of the larger size ball. This is because the ball must be larger than the valve opening in order for it to occlude the orifice completely. Consequently, in the mitral position, a relatively large ball lying in the middle of the ventricle partially obstructs blood flow. Compared to other mechanical valves, the inlet orifice diameter compared to the overall valve diameter is less favorable. Effective orifice size may not reflect the actual (larger) measured orifice because the former is affected by the shape of the valve orifice (Fig. 18.2).
![]() FIGURE 18.2. A: Ball-cage valve in a closed position. B: Complex flow around a ball-cage valve. This illustrates how the effective orifice area is affected by a high profile valve. |
Caged Disc Valves
Caged disc valves include the Cooley-Cutter, the Beall, the Cross-Jones, the Harken, the Starr-Edwards (6500 series), the Kay-Suzuki, and the Kay-Shiley. Although these valves have all been discontinued, some are still functional more than 20 years after implantation (Fig. 18.3).
Low Profile Valves
The introduction of low profile valves reduced the “mass to orifice ratio,” thereby improving hemodynamics. Most low profile mechanical valves are made of pyrolitic carbon to reduce thrombogenicity and improve durability.
Tilting Disc Valves
Tilting disc valves were introduced in 1967 with the goal of achieving a low profile and reducing bulk within the left ventricular cavity. They have an intermediate range of valve obstruction. The circular disc of the valve is attached to the sewing ring by way of an eccentric strut/hinge mechanism. Back pressure on the larger portion of the disc causes the valve to close. The opening angle of the disc is important, because the gradient will increase when the angle is less than 60 degrees from the sewing ring. The opening angle is typically 60-70 degrees. To ensure proper valve closure, this angle must be less than 90 degrees. If the opening angle is too great, the regurgitant volume will increase. A certain amount of valvular insufficiency is felt to be beneficial as it reduces the problem of periprosthetic flow stagnation, which could lead to thrombosis. In the open position, there is an area of stagnation behind the valve because the opening angle is less than 90 degrees. Flow across tilting disc valves is complex. These valves have a major orifice (70% of flow) and a minor orifice. The orientation of the orifice is important because it affects flow dynamics. As blood flow opens the disc, it is directed laterally through each orifice (Figs. 18.4 and 18.5) (7,8,9,10).
Bjork-Shiley Valve
The Bjork-Shiley valve was originally manufactured in 1969. It was removed from the United States market because of problems with strut fractures due to weld fractures and disc embolization. It consists of a free-floating disc, which is suspended in a stellite cage with a major inflow and minor outflow C-shaped struts.
The Bjork-Shiley Monostrut valve was subsequently developed and eliminated the two-armed welded outflow strut entirely; instead it has a single thick arm, which is part of the housing in a single piece. It is still sold in Europe.
Medtronic Hall Valve
The Medtronic Hall valve, previously known as the Hall-Kaster valve, was developed in 1975. It is the most commonly implanted single-disc valve in the United States. It consists of a disc, housing, struts, and ring. The disc moves up and down on the S-shaped guide strut. It is still in use (Fig. 18.4).
OmniScience Valve
The OmniScience valve is a descendant of the Lillehei-Kaster disc valve. It was manufactured in 1978. It has a curved pivoting disc, a one-piece cage, and a sewing ring.
Wada Cutter Valve
The Wada Cutter valve is a single-disc valve with two metal struts at the sides of the valve housing to hold the disc in place. It was manufactured in 1967, and subsequently discontinued in 1972.
Bileaflet Valves
Bileaflet valves have the advantage of being less obstructive (flow is across three orifices) and have the lowest pressure gradients, especially with the smaller sizes. They also have the greatest regurgitant fraction (10%), due in part to asynchronous closure of the leaflets and a long closing arc. Advantages of the bileaflet prostheses over the tilting disc valves include a reduced area of stagnation, and a larger unobstructed and symmetrical flow area.
Bileaflet valves make up approximately 90% of the mechanical valve market share in the U.S. The St. Jude valve is the prototype “gold standard” against which all mechanical prostheses are compared (Figs. 18.6, 18.7 and 18.8).
St. Jude Valve
The St. Jude valve was manufactured in 1977. It has two semicircular leaflets attached to a sewing ring by a midline hinge mechanism. The leaflets pivot open (no rotation) during valve opening. When the valve opens there are two large lateral semicircular openings and a smaller central rectangular opening. The leaflets open to 85 degrees from horizontal and the angle of closure is at 30 to 35 degrees to the orifice plane (varies with valve size). Because of the large opening angle, the effective valve orifice closely approximates the area of the sewing ring. This wide angle of excursion, however, allows for significant backflow across the valve (approximately 10% regurgitant volume) (11).
Carbomedics Valve
The Carbomedics valve was introduced in 1986. It is structurally different from the St. Jude valve but has the
same echo characteristics. Pressure gradients across this prosthesis are similar to the St. Jude valve.
same echo characteristics. Pressure gradients across this prosthesis are similar to the St. Jude valve.
Other Bileaflet Valves
Other manufactured bileaflet mechanical prostheses include the Edwards Duromedics valve (withdrawn due to disc fractures), the Medtronic Parallel valve (withdrawn due to a high incidence of thromboembolism), and the On-X valve, which was manufactured in 1996.
Bioprosthetic Valves
The first use of intracardiac tissue valves occurred in 1962 when Heimbecker, et al. implanted a stentless cadaveric aortic valve in the mitral position (12), and Ross implanted the same in the aortic position (13,14). The low availability of these cadaveric valves led to the subsequent use of heterografts in 1965 by Binet et al. (15). Limited durability was improved by the use of glutaraldehyde preservation as described by Carpentier et al. in 1969 (16); ease of implantation was facilitated by mounting these valves on a stent as described by Reis et al. in 1971 (17).
Tissue valves are distinguished by their tissue composition. They are typically made of animal (heterograft, xenograft) or human tissue (homograft), and the tissue can be of valvular or nonvalvular origin. Today’s bioprosthetic valves are made of biologic material and include homograft valves, porcine aortic valves, and bovine pericardial tissue valves. These valves are well suited for patients at risk for hemorrhage, women who plan to bear children, and those who will not reliably follow a strict anticoagulation regimen. Unfortunately, the bioprostheses have a limited durability. This is generally due to progressive collagen disruption and calcification, leading to stenosis and eventual leaflet disruption.
Mitral bioprostheses have a higher failure rate than aortic bioprostheses. This is believed to be related to mechanical closure stress, bending of the struts by left ventricular contraction, and atrial fibrillation. A greater back pressure between the left ventricle and atrium in systole compared to the aorta and left ventricle in diastole has also been implicated.
Smaller sized valves have a greater obstruction to flow. Flow characteristics are improved in the larger sized valves when compared to mechanical prostheses, and there is less shear stress to blood elements. Valve orientation during insertion is unimportant because the orifice is central. Care must be taken, however, to ensure that the struts do not impinge on the left ventricular outflow tract for mitral prostheses.
Mitral Homograft Valves
Homografts are cryopreserved human cadaveric valves either stented or unstented. They are harvested shortly after death (when the endothelium is still viable). Stented mitral homografts have not proven very successful, with a high incidence of failure within 5 years due to leaflet thickening, calcification, and the development of valvular insufficiency.
Mitral homografts are more difficult to implant, and the patient’s valve size must be known beforehand. Early attempts at homograft mitral valve replacement yielded a high failure rate because of technical problems, including inadequate sizing of the graft and early dehiscence of the papillary muscle anastomosis. Good results have recently been reported in Europe (18,19,20,21,22).
Aortic Homograft Valves
Aortic homografts are cryopreserved human cadaveric aortic valves. Typically, a mini root replacement is done with reimplantation of the coronary ostia. This is an attractive alternative for younger patients and patients with endocarditis. Another advantage of these valves is a notable resistance to infection. Earlier methods of chemical preservation led to early valve failure (24). Antibiotic sterilization and cryopreservation have resulted in significant improvements in long-term valve performance (25). Size limits the availability of this type of valve because the patient’s annulus must match that of the homograft.
The Ross procedure is a double valve procedure in which the patient’s pulmonic valve is placed in the aortic position as an autograft, and the pulmonic valve is then replaced with an aortic homograft (26). The right and left coronary ostia must be reimplanted (some surgeons use an inclusion technique where the pulmonic valve is inserted into the aortic root without removing the coronary ostia). A major concern with this procedure is the risk of injury to the first septal perforator (near the base of the
pulmonic valve), which can lead to high septal infarction and death. The procedure is well suited to younger, growing patients because the autograft can continue to grow with the cardiovascular tree (Fig. 18.9) (23).
pulmonic valve), which can lead to high septal infarction and death. The procedure is well suited to younger, growing patients because the autograft can continue to grow with the cardiovascular tree (Fig. 18.9) (23).
Stented Porcine Valves
The stented porcine bioprostheses in current use are the Carpentier-Edwards and Hancock valves. These valves consist of a stented preserved porcine aortic valve attached to a sewing ring. Prior to mounting, the valve leaflets are treated with glutaraldehyde, which reduces their antigenicity. These leaflets are stiffer than those on a homograft, because the tissue is nonviable. As a result of this stiffness, incomplete valve opening occurs at low flow rates.
The greatest disadvantage of these valves is their lack of long-term durability. Patient age greatly affects the rate of degeneration, with the highest failure rates occurring in patients under 20 years of age. This is thought to be due to a more active immune reaction to the foreign tissue. Valve failure is generally due to stenosis (cusp calcification), leaflet thickening, leaflet fracturing, or insufficiency (torn leaflets).
Porcine valve degeneration usually occurs gradually, but can also have an acute onset. The valve surface tends to undergo endothelial denudation with basement membrane exposure, leading to the deposition of fibrin, platelet aggregates, activated leukocytes, and small focal calcium deposits. The fixation process also makes the collagen more brittle. Approximately 10% of these valves have some degree of central regurgitation. With mitral annular calcification, the sewing ring may be distorted, leading to regurgitation (Fig. 18.10) (27,28,29,30,31,32,33,34,35).
Hancock Porcine Valve
The Hancock valve was the first commercially successful bioprosthetic valve, a treated stented porcine aortic valve. It was manufactured in 1970 and is still widely used. It comes in two models, which look alike on echocardiographic exam.
![]() FIGURE 18.9. Aortic valve homograft. Please note a portion of the left ventricular outflow tract muscle with the anterior mitral valve leaflet attached along with a section of the ascending aorta. |
Carpentier-Edwards Porcine Valve
The Carpentier-Edwards treated aortic porcine valve has been on the market since 1976, and is the most frequently implanted biological valve. The frame is flexible and the sewing ring is saddle-shaped.
Bovine Pericardial Valves
Pericardial valves make up approximately 40% of the U.S. tissue valve market, and 16% of all U.S. valves. Their first clinical use was in 1971. Bovine pericardial valves are similar to porcine valves; however, the three leaflets are made from bovine pericardium. The advantages over porcine valves include unlimited size and easy modification of valve design.
The most common causes of pericardial valve failure were abrasion of the leaflets by the support frame and leaflet calcification leading to valvular incompetence. Cusp tears tended to occur along the free edges of the cusp and at the leaflet crease. The cloth covering of the stents was believed to cause abrasion of the pericardial commissures and cusps. Cusp perforations were also caused by long suture ends. Calcification of pericardial valves tended to occur in the line of flexion parallel to the annulus (porcine valves tend to become calcified at the commissures) (Fig. 18.11) (36,37).
Ionescu-Shiley Valve
The Ionescu-Shiley valve is the prototype and was manufactured in 1976. Premature failure typically occurred after 6 to 8 years. Some of the failures were due to tears occurring along the suture line of the valve leaflets to the stent posts. This valve is no longer commercially available.
Carpentier-Edwards Perimount Valve
The Carpentier-Edwards Perimount pericardial bioprosthesis was introduced clinically in 1981 and approved for commercial use in the U.S. in 1991. It is the only pericardial valve that is commercially available in the United States. Its design is similar to the CE porcine valve, with thicker leaflets. It is made for use in the aortic position and recently for the mitral position. The valve shows a similar durability profile to the porcine valve.
Stentless Porcine Valves
The Medtronic Freestyle stentless aortic xenograft and the St. Jude Medical Toronto stentless porcine valve (SPV) are the two stentless aortic valves currently available on the U.S. market.
The Prima valve is another stentless valve undergoing clinical trials, but it is not yet commercially available. Because mechanical and stented aortic prostheses are relatively stenotic, the stentless valves were developed in an effort to provide a larger effective orifice area. Benefits of these stentless valves include regression of left ventricular hypertrophy, a factor that has been linked to survival (38,39,40,41). Both valves are derived from the porcine aortic root and lack any sewing ring or supporting struts. They have a single layer of polyester covering around the outer base of the valve (Fig. 18.12).
ECHOCARDIOGRAPHIC EVALUATION OF PROSTHETIC HEART VALVES
Because of the large number of prostheses, a wide range of sizes, and a wide variety of pathologic findings, the echocardiographer must have a good understanding of the appearance and function of the commonly implanted prosthetic heart valves.
A complete examination should include evaluation of:
Function of the prosthesis
Chamber size
Presence of intracardiac densities (e.g., thrombus, vegetations, pannus formation)
Ventricular function
Valvular lesions
Hemodynamic measurements (gradients, effective orifice area)
Regurgitant jets (normal vs. pathologic)
The 2D and color flow Doppler examination of prosthetic valves can be challenging because of:
Dense reverberations
Dense sewing ring
NORMAL IMAGING
Prosthetic heart valves have characteristic echo appearances. They produce a certain degree of acoustic shadowing and characteristic reverberations. Acoustic shadowing occurs because of the highly reflective material in prosthetic valves and usually occurs on the opposite side of the prosthesis. Reverberations need to be distinguished from vegetations or thrombus.
The elongated appearance of parts of the prosthesis on the echo exam is due to the differential speed of sound through the dense prosthesis versus through the tissue. The valve’s occluding mechanism determines the pattern of flow and shadowing/artifact. The sewing ring should be examined closely for stability (i.e., no rocking motion),
and disc/leaflet/poppet motion should be thoroughly evaluated. All valves should be scanned from multiple views and planes.
and disc/leaflet/poppet motion should be thoroughly evaluated. All valves should be scanned from multiple views and planes.
Baseline function should be determined after valve replacement prior to hospital discharge (immediate postcardiopulmonary bypass results may be different from findings prior to hospital discharge). This allows monitoring of valve function over time. The type and size of valve should be documented, as well as previously measured gradients and duration of implantation (46,47).
HEMODYNAMIC MEASUREMENTS
Normal Doppler hemodynamic measurements for the prosthetic mitral valve include:
Early peak velocity (E wave) of mitral inflow
Mean gradient
End-diastolic gradient
Pressure half-time
Estimated valve orifice area
Tricuspid regurgitation velocity (to estimate PA pressure)
Normal Doppler hemodynamic measurements for the prosthetic aortic valve include:
Peak and mean gradients
Dimensionless ratio
Valve Gradients by CW Doppler
Each valve type and size has its own hemodynamic characteristics. Even though peak and mean valvular gradients are only grossly correlated to the size of the prosthesis, such measurements are important in practice. They can reflect increased stroke work and can be abnormally elevated because of pathologic stenosis/obstruction, a high cardiac output state, increased stroke volume (e.g., regurgitation), or a mismatch between prosthesis size and body size. With the exception of the Starr-Edwards valve, the diameter of the valve is generally the size of the sewing ring. The point to remember is that transvalvular gradients obtained by Doppler are very flow-dependent. With increasing prosthetic valve size there is a notable decrease in peak velocity and mean gradient for all valve types (48,49).
There is a very good correlation between simultaneous Doppler mean and catheter gradients using the simplified Bernoulli equation (50). In a simultaneous Doppler/catheter study, left atrial pressure (LAP) by transseptal puncture correlated well with the mean Doppler gradient; however, pulmonary capillary wedge pressure (PCWP) significantly overestimated the mean gradient in several cases.
Normal prosthetic gradients depend on the size and type of the prosthesis, valve location, and ventricular function. For all measurements, an average of several beats should be taken (especially when there is an irregular cardiac rhythm). Furthermore, measurement of exercise hemodynamics can uncover mild dysfunction, and may augment the differences noted between different prostheses (51,52).
The phenomenon of “pressure recovery” must be considered because it can become a source of error. The point of highest velocity and lowest pressure occurs at the narrowest point in a prosthetic valve or at the vena contracta downstream (minimum jet cross section). Velocity decreases and pressure increases (“pressure recovery”) as one moves further from the prosthesis because of reexpansion of flow downstream. The pressure gradient will therefore depend on where the pressure is sampled. Using CW Doppler, the peak velocity should be recorded at the narrowest point of the prosthetic valve. “Pressure recovery” is more significant in prosthetic than native valves (especially aortic prostheses). Valve design and size will influence the extent of pressure recovery. As a result, Doppler-derived gradients can be misleading (53).
Effective Orifice Area
Effective orifice area (EOA) is superior to transvalvular gradients as a measure of prosthetic valve function and performance. All valve prostheses are mildly stenotic and the EOA is always less than the anatomic area. The prosthesis EOA gives a more flow-independent measure of resistance, as with stenotic native valves. Gradients, on the other hand, are more dependent on flow, diastolic filling time, heart rate, valve size, and valve type. Unfortunately, measurement of EOA for mitral prostheses has been imprecise (54,55,56,57,58,59,60,61,62,63,64,65). For native mitral valves, it is generally measured using the pressure half-time method as described by Hatle (55):
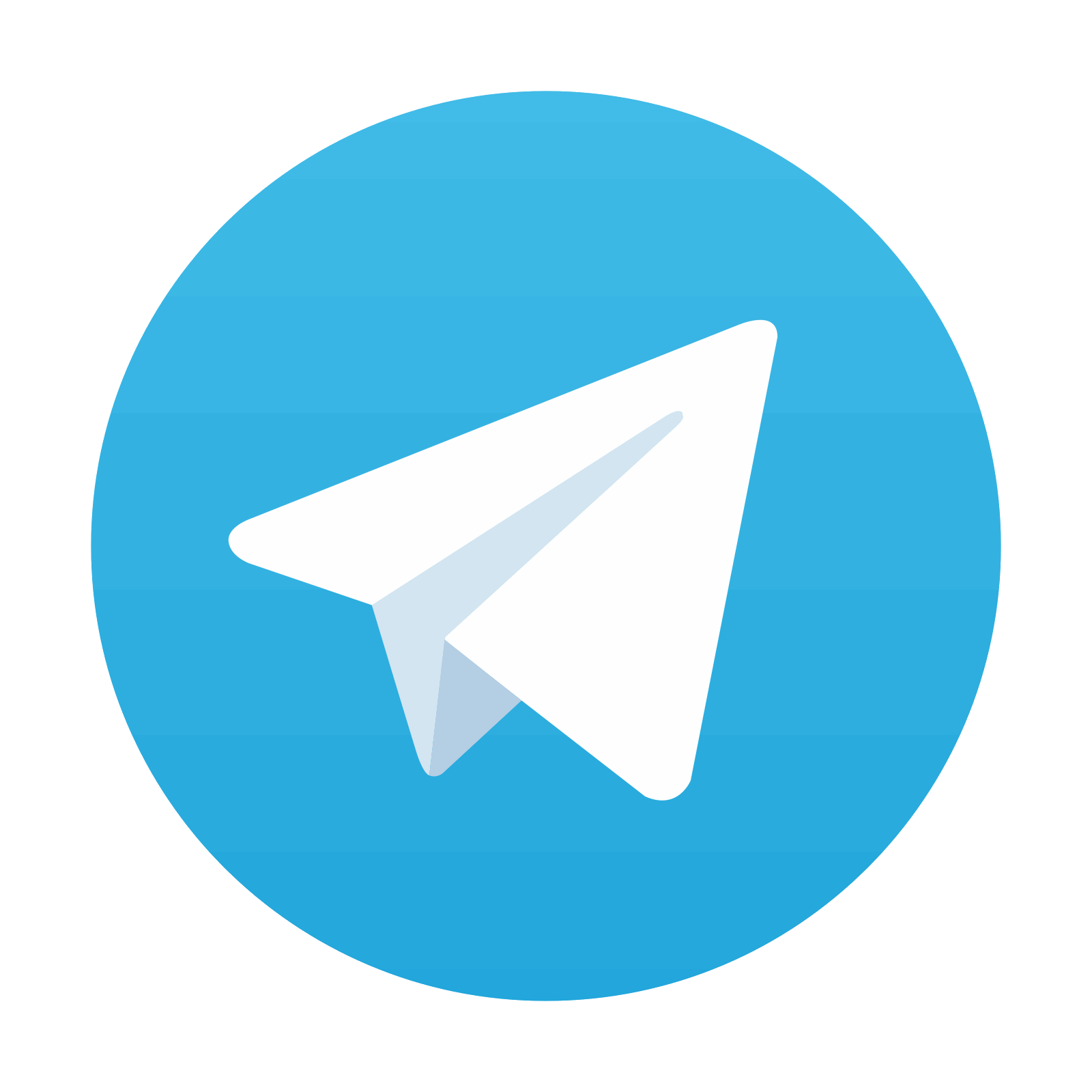
Stay updated, free articles. Join our Telegram channel

Full access? Get Clinical Tree
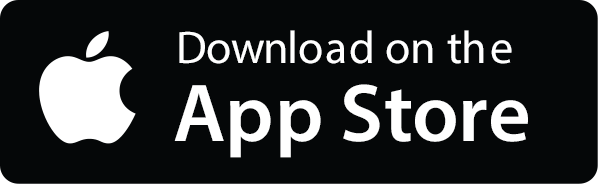
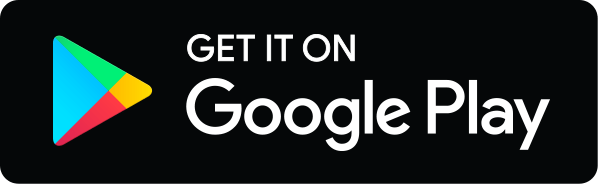