Background
Analysis of myocardial deformation from data stored in Digital Imaging and Communications in Medicine format using vendor-independent software may be useful for clinical and research purposes but has not been evaluated in children.
Methods
Grayscale images were prospectively acquired on Vivid 7 (GE Healthcare) and iE33 (Philips Medical Systems) ultrasound systems in 49 children. Digital Imaging and Communications in Medicine and raw data were analyzed using vendor-independent software (Cardiac Performance Analysis, Tomtec Imaging Systems) and vendor-specific software (EchoPAC and QLAB) and results compared. In addition, vendor-independent software using images at 30 frames/sec were compared with images at the higher acquisition frame rate.
Results
Measurement of short-axis radial and circumferential strain (ϵ) and apical four-chamber longitudinal ϵ by vendor-independent software was possible in >92% of the children. Intraobserver and interobserver coefficients of variation for global circumferential and longitudinal ϵ ranged from 7.1% to 15.3% and for radial ϵ from 23.9% to 30.2%. Strain values were somewhat higher when using GE images at acquisition frame rates compared with ϵ values using GE images stored at 30 frames/sec. Strain values obtained by vendor-independent software were comparable with those obtained by vendor-specific software for longitudinal ϵ and higher for circumferential ϵ. Radial ϵ values obtained by vendor-independent software were lower than ϵ values by EchoPAC and higher than ϵ values by QLAB.
Conclusions
Vendor-independent software–derived ϵ is feasible and potentially valuable for measuring myocardial deformation in research and in multicenter studies using images from different ultrasound systems, especially for longitudinal deformation. However, a systematic bias for circumferential ϵ and a high variability in radial ϵ measurements remain concerns.
Myocardial deformation imaging by echocardiography might lead to a more objective assessment of global and regional myocardial function. Assessment of myocardial performance by speckle-tracking echocardiography, which tracks the B-mode natural acoustic markers (speckles) frame by frame throughout the cardiac cycle, has increased, as this technique allows relatively accessible and angle-independent analysis of myocardial strain (ϵ) and ϵ rate by dedicated software. To date, this analysis has required software that is proprietary to the ultrasound machine on which the images were obtained. Hence, deformation analysis from echocardiographic data acquired on a GE machine can be done only using EchoPAC software (GE Healthcare, Milwaukee, WI), and deformation analysis on Philips images requires QLAB software (Philips Medical Systems, Best, The Netherlands). More recently, speckle-tracking echocardiography ϵ can also be analyzed from images stored in Digital Imaging and Communications in Medicine (DICOM) format regardless of which system was used to capture the data, referred to in this report as vendor-independent software (Cardiac Performance Analysis; TomTec Imaging Systems, Unterschleissheim, Germany). This application has potential practical implications for multicenter studies using different ultrasound machines as well as for the retrospective analysis of myocardial function from previously acquired images stored in DICOM format. Furthermore, the availability of a common, nonproprietary analysis system presents a unique opportunity to compare commonly used deformation analysis programs. However, the use of vendor-independent software for deformation analysis has not been extensively studied. In addition, it is unknown whether myocardial ϵ values by vendor-independent software using images acquired on different ultrasound machines are comparable. Therefore, the purposes of this study were (1) to assess the feasibility and reliability of ϵ measurements by vendor-independent software in children, (2) to compare vendor-independent software-derived ϵ values using DICOM data from two common ultrasound systems, and (3) to compare deformation results obtained by vendor-independent software with those derived from vendor-specific software.
Methods
Study Participants
Children with or without heart disease were prospectively enrolled between February 2009 and October 2009. The wide range of congenital and acquired cardiac diagnoses is shown in Table 1 . Participants were recruited in the outpatient clinic when scheduled to undergo clinically indicated echocardiography and from healthy volunteers. Children with single-ventricle physiology and cardiac arrhythmias were excluded. No children were excluded a priori on the basis of expected poor echocardiographic windows. The study was approved by the institutional research ethics board, and written informed consent was obtained from participants and their legal guardians.
Variable | Value |
---|---|
Age (years) | 12.4 ± 4 (4.4 to 17.7) |
Men | 31 (63%) |
Weight (kg) | 42 ± 16 (16.4 to 73.8) |
Height (cm) | 146 ± 19 (105 to 171) |
BSA (m 2 ) | 1.29 ± 0.3 (0.69 to 1.76) |
BMI (kg/m 2 ) | 19 ± 4 (12 to 30) |
Systolic blood pressure (mm Hg) | 102 ± 13 (81 to 114) |
Diastolic blood pressure (mm Hg) | 57 ± 9 (40 to 61) |
Diagnosis | |
Normal heart | 12 (24%) |
Heart transplant recipient | 6 (12%) |
Systemic arterial hypertension | 3 (6%) |
Cardiomyopathy | 5 (10%) |
Post–Kawasaki disease | 1 (2%) |
Post–anthracycline treatment | 1 (2%) |
Congenital heart disease | 21 (43%) |
Heart rate (beats/min) | 72 ± 15 (41 to 119) |
LV EDD Z score | 0.73 ± 1.61 (−3.2 to 6.4) |
LV ESD Z score | 0.40 ± 1.56 (−3.3 to 6.9) |
Shortening fraction (%) | 37 ± 6 (23 to 53) |
Ejection fraction (%) | 63 ± 7 (46 to 77) |
LV ejection time (msec) | 296 ± 44 (201 to 458) |
Echocardiographic Image Acquisition
Participants were scanned with GE Vivid 7 (GE Healthcare) and Philips iE33 (Philips Medical Systems) ultrasound systems within a 30-min time frame, by a single sonographer (C.S.). An S4 or S5 curved-array transducer was used for the GE system and an S5 curved-array probe for the Philips system.
Each participant first underwent an extensive initial assessment of cardiac anatomy and cardiac function with one ultrasound system, which included the acquisition of tissue Doppler data and grayscale images optimized for speckle-tracking analysis. Assessment with the other ultrasound system followed. The order in the examinations on the two machines were done was dependent on the clinical indication for the echocardiographic request. Per the clinical protocols instituted in our laboratory, for children with cardiomyopathy and children without heart disease, the first examination was performed on a GE system and the second on a Philips system. For patients who underwent echocardiographic examinations for predominantly anatomic and hemodynamic evaluation, the Philips system was used first, followed by the GE system. Participants were examined at rest in the left lateral decubitus position during sinus rhythm. M-mode images from the parasternal long axis and short axis were acquired to calculate ejection time, R-R duration, left ventricular dimensions, and shortening fraction.
B-mode images were acquired at a frame rate of 55 to 90 frames/sec, as recommended by the vendors for optimal speckle-tracking echocardiography. Parasternal short-axis images at the midventricular level and apical four-chamber and two-chamber images of the left ventricle were obtained from two cardiac cycles. Images were stored as raw data at the acquisition frame rate as well as in DICOM format on a syngo Dynamics version V5.1 server (Siemens Medical Solutions Health Services, Malvern, PA) at a default setting of 30 frames/sec for both GE and Philips. In addition, to compare ϵ analysis at the lower default storage frame rate of 30 frames/sec with the higher acquisition frame rate, GE raw data (at the acquisition frame rate) were uploaded as DICOM images into the vendor-independent software system. This was not possible for Philips images.
Offline Analysis
Vendor-independent software (Cardiac Performance Analysis, version 1.0; TomTec Imaging Systems) was used. The endocardial border was manually traced at end-systole (minimal volume) in short-axis and apical four-chamber views. Tracking of the endocardial border was visually inspected and manually adjusted if necessary. After obtaining satisfactory endocardial tracking, the epicardial border was added to incorporate the entire myocardial wall. Tracking was reinspected throughout the cardiac cycle and adjusted as necessary. The “average heart cycle” mode was not used as recommended by the manufacturer. Lagrangian ϵ curves were then rendered by the software ( Figure 1 A). The cardiac cycle with the best tracking and visually most credible ϵ curves was selected for analysis. The maximal ϵ value was recorded in each of 12 segments (radial and circumferential ϵ for the anterior septum, anterior, lateral, posterior, inferior, and septum from short-axis views and longitudinal ϵ for the basal septum, midseptum, apical septum, apical lateral, midlateral, and basal lateral from apical four-chamber views). Because one of the objectives of this study was to compare ϵ curves between systems using a common platform, only segments with ϵ values > 5% were included in the analysis. Images were subjectively categorized as good, reasonable, or immeasurable on the basis of a combination of image quality, tracking performance, and the quality of the ϵ curves obtained for the different vendor-independent software images.

Vendor-specific analysis software (EchoPAC version 7.0 and QLAB version 7.0) was used for GE and Philips images, as previously detailed. In brief, for both EchoPAC and QLAB, the endocardial border was manually traced at end-systole. Automatic tracking was accepted after visual inspection and when the software indicated adequate tracking. Peak radial and circumferential Lagrangian ϵ was measured from the short-axis view and peak longitudinal ϵ from the apical four-chamber view. Examples of circumferential ϵ curves are shown in Figures 1 B and 1 C.
Left ventricular ejection fraction was measured offline using the biplane Simpson’s method (syngo Dynamics).
Statistical Analysis
Data are presented as mean ± SD, ranges, and frequencies as appropriate. Global ϵ was calculated as the average of the segments when three or more of six segments were measurable. Agreement between machines, software, and techniques for continuous variables was calculated using Bland-Altman statistics, including mean bias (mean difference between paired measurements), statistical significance of the mean bias using the paired t test (the null hypothesis was zero bias), and the lower and upper limits of agreement (95% confidence interval). For Bland-Altman plots, the percentage difference (difference between paired measurements divided by the average of the paired measurements × 100) was calculated. In addition, Pearson’s correlation and its statistical significance were calculated as measures of raw associations between measurements. Fifteen participants were measured by the same observer after 2 weeks to obtain intraobserver variability and by a second observer to obtain interobserver variability. Interobserver and intraobserver agreement were calculated using Bland-Altman statistics and by calculating the coefficient of variation (standard deviation of difference of the paired measurements divided by the average of the paired measurements). A univariate linear regression model using maximum likelihood estimates for parameter determination was used to assess the influence of cardiac diagnosis (independent variable) on the difference between modalities (dependent variable). Statistical analysis used SAS version 9.1 (SAS Institute Inc, Cary, NC).
Results
General Characteristics
Forty-nine children were recruited, and their characteristics are shown in Table 1 . Of the five children with cardiomyopathy, three had dilated cardiomyopathy and two had hypertrophic cardiomyopathy. Congenital heart disease diagnoses ( n = 21) included tetralogy of Fallot ( n = 5), ventricular septal defect ( n = 6), left ventricular outflow tract obstruction ( n = 7), Ebstein’s anomaly ( n = 1), pulmonary stenosis ( n = 1), and transposition of the great arteries ( n = 1). Most children had normal shortening fractions and left ventricular dimensions.
Image Acquisition
The mean frame rates for GE images (acquisition frame rate) analyzed by vendor-independent software or EchoPAC were 73 ± 19 frames/sec for short-axis views and 65 ± 16 frames/sec for apical four-chamber views. The mean frame rates for QLAB was 73 ± 17 frames/sec for short-axis views and 71 ± 14 frames/sec for apical four-chamber views.
Feasibility and Reproducibility of ϵ Measurement by Vendor-Independent Software
Subjective image quality for short axis-views was as follows: for GE images at 30 frames/sec, good in 57%, reasonable in 37%, and immeasurable in 6%; for GE images at the acquisition frame rate, good in 57%, reasonable in 41%, and immeasurable in 2%; and for Philips images at 30 frames/sec, good in 59% and reasonable in 41%.
Image quality for apical four-chamber views was as follows: for GE images at 30 frames/sec, good in 33%, reasonable in 59%, and immeasurable in 8%; for GE images at the acquisition frame rate, good in 29%, reasonable in 69%, and immeasurable in 2%; and for Philips images at 30 frames/sec, good in 51%, reasonable in 43%, and immeasurable in 6%.
Overall, measurement of global short-axis radial and circumferential ϵ and apical four-chamber longitudinal ϵ by vendor-independent software was possible (quality score good or reasonable) in >92% of children for GE images at 30 frames/sec, GE images at the acquisition frame rate, and Philips images at 30 frames/sec. When segments were analyzed separately, feasibility was lowest for radial ϵ using GE images at the acquisition frame rate analyzed by vendor-independent software in the septal segment (76%).
Offline analysis time for all vendor-independent software ϵ values described was approximately 10 minutes per patient. For the vendor-specific software, feasibility for acquiring acceptable ϵ curves ranged from 78% for short-axis radial ϵ of the anterior segment using QLAB and 92% for circumferential ϵ of the posterior wall segment for EchoPAC to 100% for circumferential ϵ using both QLAB and EchoPAC. Measurement of global short-axis radial and circumferential ϵ and apical four-chamber longitudinal ϵ by QLAB and EchoPAC was possible in >98% of the participants. Intraobserver and interobserver variability for global ϵ parameters is shown in Table 2 .
GE-VIS-30 | GE-VIS-AFR | PH-VIS-30 | |||||||||||||
---|---|---|---|---|---|---|---|---|---|---|---|---|---|---|---|
Variable | Mean ± SD | R | Bias | 95% CI | COV | Mean ± SD | R | Bias | 95% CI | COV | Mean ± SD | R | Bias | 95% CI | COV |
Intraobserver variability | |||||||||||||||
Global radial ϵ SAX (%) | 23 ± 8 | 0.82 | 0.2 | −11 to 11 | 23.9 | 29 ± 8 | 0.67 | −1.8 | −16 to 12 | 24.8 | 27 ± 7 | 0.53 | 0.9 | −14 to 16 | 28.1 |
Global circumferential ϵ SAX | −23 ± 2 | 0.54 | 0.5 | −4 to 5 | 11.0 | −26 ± 3 | 0.85 | 0.8 | −3 to 5 | 7.4 | −25 ± 4 | 0.86 | 0.9 | −4 to 5 | 9.0 |
Global longitudinal ϵ AP4CH | −21 ± 3 | 0.64 | 0.7 | −5 to 6 | 14.1 | −22 ± 3 | 0.81 | 0.7 | −4 to 5 | 10.6 | −21 ± 2 | 0.45 | 0.5 | −5 to 6 | 14.3 |
Interobserver variability | |||||||||||||||
Global radial ϵ SAX (%) | 25 ± 8 | 0.73 | −3.9 | −17 to 10 | 27.3 | 32 ± 9 | 0.52 | −7.6 | −27 to 11 | 30.2 | 28 ± 8 | 0.57 | −2.0 | −18 to 15 | 30.0 |
Global circumferential ϵ SAX | −23 ± 3 | 0.85 | −0.1 | −3 to 3 | 7.1 | −26 ± 3 | 0.80 | −0.3 | −5 to 6 | 10.6 | −24 ± 4 | 0.84 | −0.1 | −5 to 5 | 8.2 |
Global longitudinal ϵ AP4CH | −20 ± 3 | 0.50 | −0.2 | −6 to 6 | 15.3 | −22 ± 3 | 0.72 | −0.2 | −6 to 5 | 12.9 | −21 ± 2 | 0.31 | 1.2 | −5 to 8 | 15.0 |
Comparison of Myocardial Deformation Analysis by Vendor-Independent Software Using Images Acquired With GE and Philips Machines
Table 3 and Figure 2 summarize comparisons of GE images at 30 frames/sec and Philips images at 30 frames/sec for circumferential, longitudinal, and radial ϵ using vendor-independent software. Global circumferential ϵ values were lower, and global longitudinal ϵ values were similar for Philips images at 30 frames/sec compared with GE images at 30 frames/sec. Limits of agreement of the bias were wider and correlations were lower for longitudinal and circumferential ϵ in individual segments compared with global ϵ values ( Table 3 ). Limits of agreement were wider and correlation was lower between GE images at 30 frames/sec and Philips images at 30 frames/sec for radial ϵ compared with circumferential and longitudinal ϵ. This was also true when individual segments were analyzed separately (data on radial ϵ for individual segments not shown). Using univariate linear regression analysis, the difference in ϵ results between GE images at 30 frames/sec and Philips images at 30 frames/sec could not be explained by the presence or absence of cardiac disease (for radial ϵ short axis: regression estimate, 3.49, P = .39; for circumferential ϵ short axis: regression estimate, −2.03, P = .09; for longitudinal ϵ apical four chamber: regression estimate −0.06, P = .96).
GE-VIS-30 | PH-VIS-30 | ||||||
---|---|---|---|---|---|---|---|
Variable | Mean ± SD | % | Mean ± SD | % | Bias | 95% CI | R |
Global radial ϵ SAX (%) | 24 ± 9 | 92 | 27 ± 9 | 96 | −3.9 ∗ | −26 to 18 | 0.17 |
Circumferential ϵ SAX | |||||||
Anterior septum | −24 ± 6 | 92 | −25 ± 6 | 100 | 1.4 | −13 to 15 | 0.40 ‡ |
Anterior | −24 ± 7 | 94 | −27 ± 8 | 100 | 2.2 | −14 to 18 | 0.41 ‡ |
Lateral | −26 ± 6 | 94 | −26 ± 8 | 100 | 0.4 | −15 to 16 | 0.38 ‡ |
Posterior | −22 ± 6 | 94 | −24 ± 8 | 100 | 2.0 | −13 to 17 | 0.43 ‡ |
Inferior | −21 ± 6 | 92 | −23 ± 6 | 100 | 2.3 | −13 to 18 | 0.01 |
Inferior septum | −25 ± 6 | 94 | −25 ± 6 | 100 | 0.7 | −11 to 12 | 0.52 ‡ |
Global | −23 ± 4 | 94 | −25 ± 4 | 100 | 1.5 † | −5 to 8 | 0.60 ‡ |
Longitudinal ϵ AP4CH | |||||||
Basal septum | −17 ± 6 | 89 | −17 ± 6 | 100 | 0.2 | −13 to 13 | 0.39 ‡ |
Midseptum | −17 ± 5 | 89 | −19 ± 5 | 100 | 1.6 ∗ | −8 to 11 | 0.52 ‡ |
Apical septum | −23 ± 6 | 92 | −23 ± 8 | 100 | −0.5 | −15 to 14 | 0.36 § |
Apical lateral | −21 ± 7 | 89 | −21 ± 9 | 100 | 0.0 | −16 to 16 | 0.51 ‡ |
Midlateral | −17 ± 5 | 90 | −18 ± 4 | 100 | 1.5 | −9 to 12 | 0.34 § |
Basal lateral | −19 ± 6 | 90 | −20 ± 6 | 96 | 1.6 | −14 to 17 | 0.19 |
Global | −19 ± 3 | 92 | −20 ± 3 | 100 | 0.8 | −6 to 8 | 0.42 ‡ |
∗ P < .05 (paired t test for difference between GE-VIS-30 and PH-VIS-30).
† P < .01 (paired t test for difference between GE-VIS-30 and PH-VIS-30).
‡ P < .01 (significance of Pearson’s correlation between GE-VIS-30 and PH-VIS-30).
§ P < .05 (significance of Pearson’s correlation between GE-VIS-30 and PH-VIS-30).



Table 4 shows the results when comparing vendor-independent software ϵ values using GE images stored at 30 frames/sec with GE images stored at the acquisition frame rate. Radial ϵ values were higher using GE images at the acquisition frame rate than at 30 frames/sec. A similar trend, although less pronounced, was seen for circumferential and longitudinal ϵ, with more negative values using GE images at the acquisition frame rate than at 30 frames/sec.
GE-VIS-30 | GE-VIS-AFR | ||||||
---|---|---|---|---|---|---|---|
Variable | Mean ± SD | % | Mean ± SD | % | Bias | 95% CI | R |
Global radial ϵ SAX (%) | 24 ± 9 | 92 | 33 ± 11 | 92 | −8.5 ∗ | −26 to 10 | 0.61 ‡ |
Global circumferential ϵ (%) | −23 ± 4 | 93 | −27 ± 4 | 98 | 3.2 ∗ | −3 to 10 | 0.69 ‡ |
Global longitudinal ϵ (%) | −19 ± 3 | 92 | −20 ± 4 | 96 | 1.4 † | −6 to 9 | 0.44 § |
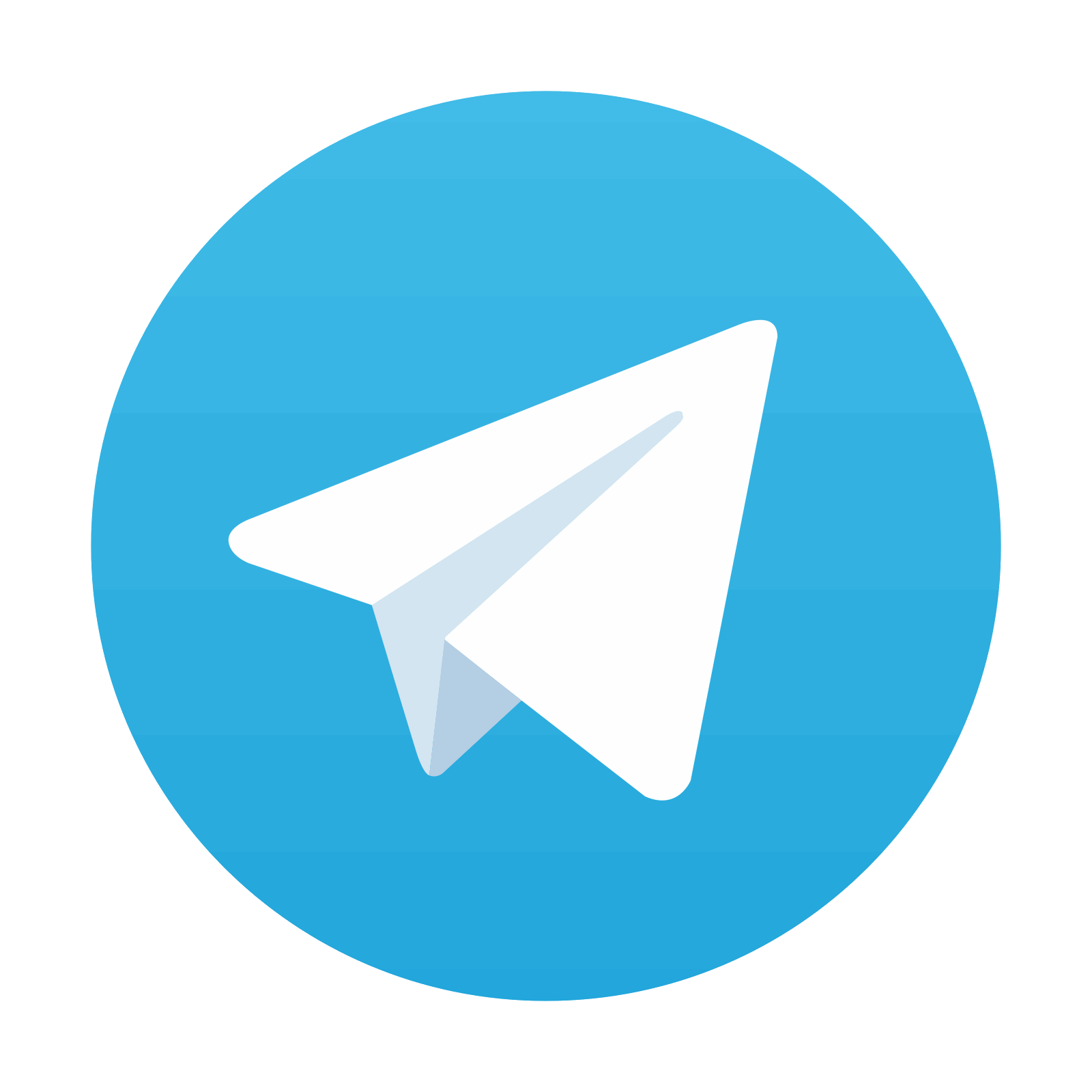
Stay updated, free articles. Join our Telegram channel

Full access? Get Clinical Tree
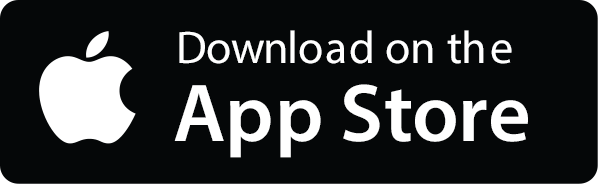
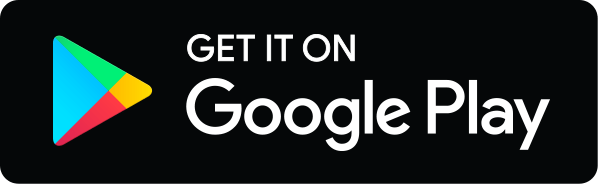
