Fig. 8.1
Kaplan–Meier survival curves for the panel of biomarkers analyzed. Cutoff values correspond to the 95 % percentile determined in the nonsmoking control subjects included in the Evaluation of COPD Longitudinally to Identify Predictive Surrogate Endpoints study. CC-16 Clara cell secretory protein-16, CCL-18/PARC chemokine ligand 18/pulmonary and activation-regulated chemokine, hsCRP high-sensitivity C-reactive protein, SP-D surfactant protein D. This figure was referred with permission from the publisher (Reprinted with permission from the American Thoracic Society. Copyright © 2015 American Thoracic Society. Celli BR, et al. Inflammatory biomarkers improve clinical prediction of mortality in chronic obstructive pulmonary disease. Am J Respir Crit Care Med. 2012;185:1065–72 [5]. The American Journal of Respiratory and Critical Care Medicine is an official journal of the American Thoracic Society)
In addition, a biomarker preferably can guide phenotyping of COPD. It is unlikely that all patients with COPD have the same underlying disease process and the same response to a specific medication. If a biomarker can differentiate a category of patients who have good response to a specific treatment from those with poor response, this is regarded as predictive biomarker [7].
Given the current evidence, it is conclusive that COPD is associated with systemic inflammation. A subgroup of patients with COPD that have persistently raised concentrations of inflammatory biomarkers often have significantly increased all-cause mortality and exacerbation frequency. However, whether persistent systemic inflammation can be a target of pharmacological treatment is far from clear. Some promising systematic anti-inflammatory medications, namely, long-term oral systemic steroids and statins, have failed to improve survival [8, 9].
Biomarkers have often been discussed as a tool to distinguish subtypes of COPD. The Evaluation of COPD Longitudinally to Identify Predictive Surrogate Endpoints (ECLIPSE) study was a large-scale 3 year observational controlled multicenter international study to classify clinically relevant subtypes of COPD and to identify novel biomarkers and genetic factors [6]. ECLIPSE confirmed that many previously known biomarkers truly have relation with COPD. This also revealed some novel biomarkers that required further validation to be used in practice. The ongoing subpopulations and intermediate outcomes in COPD study is another large-scale study that deals both with phenotyping and biomarkers. This is a multicenter longitudinal, observational study to identify novel phenotypes and biomarkers of COPD [2].
In this chapter, we will review COPD biomarkers that can be used for diagnosis, phenotyping, and evaluating severity, prognosis, therapeutic effect, and future risk to develop COPD, focusing on inflammatory substances. In addition, we will review biomarkers that are related to comorbidities.
8.2 Sputum
Patients with COPD usually produce more sputum than healthy persons. Sputum samples were expected to be ideal to investigate the airway inflammation because sputum is produced in the airway, in contrast to blood, which is made in bone marrow, and because sputum can be obtained noninvasively. However, the examination of sputum on inflammatory biomarkers seemed difficult and unreliable until 1992 when the sputum induction technique was established to obtain sputum from healthy subjects and subjects with airway diseases. The sputum analysis technique has been refined to provide reliable results for an increasing number of inflammatory biomarkers. Initially, these biomarkers were studied mainly in patients with asthma and chronic bronchitis. Later, sputum biomarkers were used in research to study the airway inflammation in COPD cases. Sputum induction is required when the sputum cannot be obtained spontaneously or obtained sputum is not of sufficient quality. Sputum induction is usually conducted with a hypertonic saline aerosol in concentrations of 4.5 %. The considerable limitation with sputum induction in patients with COPD and asthma is that the saline aerosol can cause bronchoconstriction. We can obtain sufficient sputum from more than 90 % of patients with COPD. After processing, cellular markers can be analyzed using flow cytometry [10, 11].
In healthy persons, macrophages and neutrophils account for approximately 60 % and 40 % of sputum cell count, respectively. Few bronchial epithelial cells, lymphocytes, eosinophils, and metachromatic cells have been observed [10].
8.2.1 Sputum Neutrophils
The number or proportion of neutrophils is labile in both healthy persons and patients with COPD cases depending on many stimuli, such as sputum induction, smoking, air pollutants, endotoxins, occupational exposures, and infections. Although it is well known that sputum neutrophilia is common in COPD subjects, it is not specific to COPD. As smoking itself induce sputum neutrophilia, sputum neutrophilia among non-COPD smokers largely diminishes the diagnostic value of sputum neutrophilia for COPD. However, among smokers with COPD, the degree of sputum neutrophilia relates weakly to the degree of airflow limitation and the degree of disease progression. Leukocyte-specific integrin CD11b/CD18 expressed on neutrophils may also be related to the severity of COPD [10].
Increased numbers of neutrophils have been found in sputum samples of stable COPD cases. Furthermore, a negative correlation has been confirmed between the proportion of neutrophils and FEV1. The neutrophilic inflammation partially explains why most COPD patients are resistant to steroid treatment.
The effect of drugs on neutrophilic inflammation in COPD was investigated in some studies. One study has reported that the sputum neutrophil count was reduced after 2 months of treatment with inhaled beclomethasone [12]. However, this requires further study, since this result conflicts with the known effect of corticosteroids to prolong the life of neutrophils. In another study, theophylline reduced induced sputum neutrophils [13].
8.2.2 Sputum Eosinophils
Although sputum samples contain relatively a small number of eosinophils compared to that of neutrophils, the significance of sputum eosinophils for COPD has been extensively investigated. Many studies have demonstrated sputum eosinophilia (sputum eosinophils > 3 %) in stable COPD patients and an inverse correlation between the number of sputum eosinophils and FEV1 value [11]. Saetta et al. reported in 1994, sputum eosinophil number increases when COPD patients experience exacerbations [14]. Fujimoto replicated increased sputum eosinophil numbers during exacerbations in Japanese cases [15]. According to Papi et al., sputum eosinophil numbers were increased, especially during virus-associated exacerbations [16].
This biomarker is clinically meaningful since COPD patients with sputum eosinophilia respond better to treatment with inhaled steroids, though controversy exists on whether eosinophils in COPD are actually activated or not. Recently proposed asthma-COPD overlap syndrome (ACOS) is a commonly encountered yet loosely defined clinical entity. Both COPD and bronchial asthma are respiratory diseases with airflow limitation. COPD and bronchial asthma share symptoms, decreased FEV1, and medications. ACOS accounts for approximately 15–25 % of the obstructive airway diseases. Patients with ACOS experience worse outcomes compared to those with asthma or COPD alone. ACOS patients are generally younger than patients with “lone COPD,” have the combined risk factors of smoking and atopy, and experience acute exacerbations with higher frequency and greater severity than lone COPD. However, these characteristics are not sufficiently sensitive or specific to distinguish ACOS from lone COPD. One of the most important reasons why we have to distinguish ACOS and lone COPD is that there are differences in treatment options [17].
The current GOLD documents recommend the first-line use of ICS only for a patient with groups C and D COPD: severe to very severe airflow limitation, ≥ 2 exacerbations per year, and/or ≥ 1 with hospitalization for exacerbation [1]. However, recent evidence questions whether ICS actually reduces exacerbations and is truly beneficial for COPD cases [18]. Both COPD and asthma are associated with chronic inflammation of the lung accompanied by airflow limitation. However, there are differences in the type of inflammatory cells and mediators involved. While ICS is indicative for bronchial asthma in which IgE and eosinophils play an important role, ICS cannot be so effective on neutrophils observed in the respiratory tract of COPD cases. The importance of ICS treatment for ACOS has been emphasized recently. Schematically, we have two COPD phenotypes. One is non-ACOS COPD characterized by low sputum eosinophils for which ICS does not have a good indication. The other is ACOS, which is accompanied by an increased number of sputum eosinophils for which ICS has good indication [18, 19].
Kitaguchi et al. observed 63 patients with stable COPD, of which 46 had COPD without asthma and 17 had COPD with asthma. A significant correlation was observed between the increases in FEV1 in response to treatment with ICS and sputum eosinophil counts (r = 0.42, P = 0.0006). Using 2.5 % as the cutoff value, receiver operating characteristic curve analysis revealed 82.4 % sensitivity and 84.8 % specificity of the sputum eosinophil count for detecting COPD with asthma [20].
Blood eosinophils and T helper type 2 may have a similar meaning, which we will discuss later.
8.2.3 Sputum Interleukin (IL)-6, IL-8
Not only inflammatory cells, but also inflammatory mediators in the sputum are associated with COPD exacerbation. Bhowmik et al. collected induced sputum samples from 57 patients with moderate to severe COPD. Median IL-6 and IL-8 levels were significantly higher in patients with ≥ 3 exacerbation a year than those with ≤ 2 exacerbation a year. In addition, the IL-6 levels of patients with exacerbations were associated with the presence of cold symptoms and to the total cell count, eosinophil count, and lymphocyte count. IL-8 level was correlated with all sputum cell counts [21].
8.3 Blood Biomarker
The majority of COPD biomarkers are derived from serum or plasma. This is because blood is easily available from veins with acceptable invasiveness and its measurements can be easily standardized. However, it is not entirely clear how the blood measurements relate to the underlying disease activity in the lungs. Another weakness of serum/plasma biomarkers is that they are often easily affected for non-COPD reasons [3].
Among numerous biomarkers in blood, inflammatory biomarkers play essential role for both stable and exacerbated COPD. According to the ECLIPSE study, systematic inflammation evaluated by white blood cell count, C-reactive protein (CRP), IL-6, IL-8, fibrinogen, and TNF-α levels potentially select a subgroup of COPD cases who may be the target of specific research and treatment [22]. Simultaneously elevated levels of CRP, fibrinogen, and leukocyte count in individuals with COPD were also associated with increased risk of having exacerbations [23]. Among these biomarkers, fibrinogen has become the most solidly established biomarker that can estimate COPD activity [24].
Eosinophil count helps in identifying subgroups that have a good response to ICS. Some other biomarkers derived from blood will be also mentioned.
8.3.1 Plasma Fibrinogen
Fibrinogen is a soluble glycoprotein in the plasma that helps in the formation of blood clots by being broken down to fibrin by the enzyme thrombin. It has a rodlike shape and shows a negative net charge at physiological pH. Fibrinogen is primarily synthesized by the hepatocytes. The concentration of fibrinogen in the blood plasma is 200–400 mg/dL in the non-acute phase and can increase threefold higher during an acute phase of stimulation such as IL-6-induced acute inflammatory response [5, 25].
Fibrinogen, which is the most robust biomarker investigated in relation to COPD so far [6], was approved as the first COPD biomarker by the US Food and Drug Administration in July 2015. The use of this biomarker is expected to make it easier to identify patients that are at a higher risk of exacerbation or death in clinical trial [26]. Researchers believe the use of fibrinogen will accelerate development of new COPD drugs.
Many published cross-sectional studies have shown that blood fibrinogen levels are higher in individuals with COPD compared with non-COPD healthy controls. According to a meta-analysis by Gan et al, the fibrinogen level was higher in COPD cases than healthy controls with the standardized mean difference of 0.47 units (95 % CI 0.29–0.65) [25].
As well as in the cross-sectional studies, large-scale general-population cohort studies have revealed that high serum fibrinogen level increased the risk for COPD-specific outcomes, future incidence of COPD, and COPD exacerbation. Valvi et al. studied 20,192 individuals from the atherosclerosis risk in communities study and the cardiovascular health study cohort and showed that fibrinogen levels at entry can predict future incidence of COPD diagnosis and COPD-related hospitalization [25]. A cohort of 5247 men randomly selected from a Malmö birth cohort also revealed that people with higher baseline fibrinogen had more hospital admissions with COPD after 25 years of follow-up, even after adjustment of covariables [25]. Dahl et al. followed up 8955 people in the general population and found that individuals with plasma fibrinogen in the upper, middle, and lower tertiles had COPD hospitalization rates of 93, 60, and 52 per 10,000 person-years [27] (Fig. 8.2). This finding is interesting as it suggests that fibrinogen can predict future COPD admission among a cohort of which most did not have a COPD diagnosis. Notably, fibrinogen is the only marker that can predict future FEV1 decline [25]. In the same study, smokers and nonsmokers with plasma fibrinogen in the upper tertile had an excess annual decline in FEV1 of 6 ml (3–9 ml) and 4 ml (2–7 ml) compared with smokers and nonsmokers with fibrinogen in the lower tertile. Based on this study by Dahl et al, fibrinogen may act as a surrogate marker of disease activity in individuals with COPD. Furthermore, fibrinogen indicates risk of COPD among a non-COPD cohort.


Fig. 8.2
Kaplan–Meier curves showing rate of COPD hospitalizations during follow-up stratified by baseline serum fibrinogen. Number at risk at the beginning of each year is shown below the horizontal axis. P < 0.001 for > 3.3 g/L versus < 2.7 g/L, p = 0.003 for > 3.3 g/L versus 2.7–3.3 g/L, and p = 0.31 for 2.7–3.3 g/L versus < 2.7 g/L on log-rank test (Reprinted with permission from the American Thoracic Society. Copyright © 2015 American Thoracic Society. Dahl M, et al. Elevated plasma fibrinogen associated with reduced pulmonary function and increased risk of chronic obstructive pulmonary disease. Am J Respir Crit Care Med. 2001;164:1008–11 [27]. The American Journal of Respiratory and Critical Care Medicine is an official journal of the American Thoracic Society)
A high level of fibrinogen can clearly predict all-cause death. Mannino et al. observed 8507 subjects of which 245 had stage III/IV COPD and 826 had stage II COPD. In this study, an elevated fibrinogen level increased the risk of mortality in subjects with stage III/IV (hazard ratio 2.11, 95 % confidence interval (CI) 1.27–3.50) and stage II (hazard ratio 1.45, 95 % CI 1.08–1.96) COPD [25].
A moderate-scale RCT in 2012 by Lomas et al. investigated whether an oral inhibitor of p38 MAP kinase, losmapimod, affects patients with COPD. In this study, 302 individuals with GOLD stage II COPD were randomized to take 7.5 mg of oral losmapimod twice daily, to take inhaled salmeterol/fluticasone propionate in a 50 μg/500 μg combination, or to take placebo. Only losmapimod was well tolerated, and it reduced plasma fibrinogen by 11 % (ratio of effect of losmapimod/placebo 0.89, 95 % CI 0.83–0.96, P = .002) [24]. However, losmapimod did not have significant effect on sputum neutrophilia, which was the primary endpoint.
Fibrinogen may be a tool for distinguishing subgroups of COPD. In a small study with 49 COPD cases, a group with emphysematous lesions involving more than 15 % of the lung parenchyma had higher fibrinogen levels than controls (n = 25) [24]. However, fibrinogen is not currently confirmed to guide a specific treatment option.
8.3.2 Serum CRP
CRP is an annular, pentameric protein, or pentraxin, which is composed of five identical subunits, found in blood plasma. The levels of CRP rise in response to inflammation, especially when caused by infection [28]. CRP is one of the most commonly used inflammatory biomarkers in practice, thanks to its availability and low cost [29]. CRP was discovered in humans in 1930 as a serum component that binds the C polysaccharide of Streptococcus pneumoniae. The reference range of CRP is < 3 mg/L, and its level often surges over 300 mg/L in severe infection when IL-6 and IL-1β trigger its production in the liver [28]. CRP has some actions in response to inflammation. It can also trigger anti-inflammatory immunoglobulin crystallizable fragments gamma inhibitory receptors. In contrast, CRP can also act as a pro-inflammatory agent by activating the classical complement cascade, through binding to the complement fragment. CRP also activates nuclear factor-kB in endothelial cells and mononuclear cells to induce proteases and pro-inflammatory cytokines [28].
In three large population-based case–control studies, levels of CRP were demonstrably higher in stable COPD patients than in controls after adjusting for the confounding factors [24]. In 2012, Zhang et al. conducted a systematic review and a meta-analysis to summarize the association between serum concentration of CRP and COPD. In this meta-analysis from 18 original studies, patients with COPD had higher serum CRP concentrations than healthy controls (mean weighted difference: 4.72 mg/L, 95 % CI 2.98–6.47) [30].
Regarding the positive significant relation between the COPD severity and inflammation, it is thought that inflammatory biomarkers and lung functions may be linked with each other. Although this issue has often been investigated, results are inconsistent partly due to study design [29]. According to a meta-analysis by Zhang et al., patients with severe COPD had higher serum CRP concentrations than those with moderate COPD (mean weighted difference 1.26 mg/L, 95 % CI 0.78–1.73) [30].
Exacerbation of COPD is usually associated with bacterial or viral infection, and CRP is a sensitive inflammatory marker to detect infection. Actually, the serum CRP level has been shown to be very sensitive to change in response to exacerbation. It is also well known that CRP is usually elevated in pneumonia patients. In a study by Lacoma et al. including 318 consecutive COPD patients, wherein 46 were in a stable phase, 217 were undergoing an exacerbation, and 55 had pneumonia, CRP showed significant differences among the three patient groups, being higher in patients with pneumonia, followed by patients with exacerbation (P < 0.0001) [31]. According to Soler et al., serum CRP level is also associated with purulent sputum during COPD exacerbation [32]. In addition, serum CRP level is useful as a prognostic marker for stable COPD [33].
Whether serum CRP level can predict a hard endpoint, death is an issue of concern. Man et al. measured serum CRP levels in 4803 participants in the Lung Health Study with mild-to-moderate COPD. The risk of all-cause and disease-specific causes of mortality was determined, adjusting for important covariates such as age, sex, cigarette smoking, and lung function. The CRP level was associated with all-cause, cardiovascular, and cancer-specific causes of mortality. Patients in the highest quintile of CRP had a relative risk of all-cause mortality of 1.79 (95 % CI 1.25–2.56) compared with those in the lowest quintile of CRP [34].
To be an acceptable surrogate biomarker, CRP should correlate with hard endpoints in RCTs. The levels of inflammatory biomarkers are expected to be ameliorated in response to treatment that suppresses inflammation. ICS is currently accepted as one of the first-choice medications for a case with high risk of exacerbation in the belief that ICS prevents future exacerbations [1]. A small observational study with 41 mild-to-moderate COPD patients was performed by Sin et al. The patients took to fluticasone (500 μg twice a day), oral prednisone (30 mg/day), or placebo over 2 weeks, followed by 8 weeks of fluticasone at 500 μg twice a day and another 8 weeks at 1000 μg twice a day. Two weeks with inhaled fluticasone reduced CRP levels by 50 % (95 % CI 9–73 %), while prednisone reduced it by 63 % (95 % CI 29–81 %). No significant changes were observed with the placebo [29]. In another study by Perng et al. 99 subjects were randomized to receive salmeterol/fluticasone, tiotropium/fluticasone, and tiotropium alone. A significant decrease in CRP was observed in all of the three arms; however, there were no statistically significant differences between the three groups. In this study, there was a significant reduction in IL-8 and matrix metalloproteinase (MMP)-9 in the salmeterol/fluticasone group compared with patients treated with tiotropium alone. The authors concluded that the anti-inflammatory effects of salmeterol/fluticasone probably contribute to the clinical benefits seen in COPD patients. This indicated that CRP could not detect the anti-inflammatory effect of tiotropium/fluticasone [29]. In another study in 2008, Sin et al. randomized 289 patients to investigate whether inhaled fluticasone alone or in combination with salmeterol over 4 weeks effect on circulating biomarkers of systemic inflammation. As a result, inhaled fluticasone with/without salmeterol did not reduce serum CRP level [29]. In conclusion, we do not have consistent evidence from RCT that CRP is modifiable by interventions mainly using ICS.
8.3.3 Blood Eosinophils
Assessment of eosinophilic airway inflammation using sputum samples is a method of judging whether a patient will benefit from ICS. However, it is technically demanding and not always successful, which reduces the usefulness of the test in routine clinical practice. Blood eosinophil count might be an alternative strategy, thanks to good correlation between blood and sputum eosinophil count.
Pascoe et al. conducted a post hoc analysis of a large-scale study including 3177 patients in the analyses, with 2083 patients (66 %) having an eosinophil count of 2 % or
higher at entry. Adding ICS reduced exacerbations by 29 % (mean 0.91 vs 1.28 exacerbations per patient per year, P < 0.0001) in patients with eosinophil counts of 2 % or higher and by only 10 % (0.79 vs 0.89, P = 0.2827) in patients with eosinophil counts lower.
This study suggested that blood eosinophils with a cutoff of 2 % are a simple predictive biomarker to know the responsiveness of ICS [35].
8.3.4 Serum Procalcitonin
Since last decade, several studies featured procalcitonin as promising biomarker for severe bacterial infection. Schuetz et al. performed a systematic review and meta-analysis to compare treatment algorithms with and without procalcitonin for acute respiratory tract infections. This review was not COPD-specific research but included patients with COPD exacerbation and patients with background COPD. They concluded that use of the procalcitonin algorithm shorten the antibiotic treatment duration for acute respiratory infections without increasing mortality or treatment failure [36].
8.3.5 White Blood Cell/Neutrophil Count in Blood
White blood cell and neutrophil counts were weakly associated with persistent systemic inflammation, frequent exacerbations, and mortality in the ECLIPSE cohort. A recent study in the general population has replicated similar observations concerning exacerbations. Similar results were also obtained absolute neutrophil counts [6].
8.3.6 Other Blood Biomarkers
In addition to fibrinogen, CRP, and eosinophils, many inflammatory biomarkers for COPD have been identified. However, the significance of most of these biomarkers as regards COPD diagnosis or COPD-related outcomes has not been sufficiently validated in replicate studies. Even if replicated, the observed relevance between most of these biomarkers and outcomes was weak, thus clinical meaning is often not clear. Although these markers are interesting for future research, they are not regarded as useful biomarkers for practical purposes at present.
CC-Chemokine Ligand-18 (CCL-18)/ Pulmonary and Activation-Regulated Chemokine (PARC)
CCL-18, previously known as PARC, is a 7-kD protein that is constitutively expressed by monocytes/macrophages and dendritic cells and is secreted predominantly in the lungs. Although the exact biological role of CCL-18 is still not clear, serum level of CCL-18 is elevated in pulmonary disease, namely, idiopathic pulmonary fibrosis, wherein serum CCL-18 levels seem to reflect fibrotic activity and correlate with survival. Concerning COPD, in one small study of patients with mild-to-moderate disease, serum CCL-18 level was associated with deteriorated FEV1 and the body mass index, airflow limitation, dyspnea, and exercise capacity index score, and acute exacerbations [37]. According to ECLIPSE and the Lung Health Study data set, serum CCL-18 levels were higher in subjects with COPD than in smokers or never smokers without COPD. Elevated CCL-18 levels were also associated with increased risk of overall mortality in the ECLIPSE cohort [6, 24]. Although these data are plausible, some questions remain unclear regarding the possible use of CCL-18 as an effective biomarker in COPD, including its relationship with clinical outcomes such as hospitalization and mortality and its responsiveness to pharmacological therapy [24]
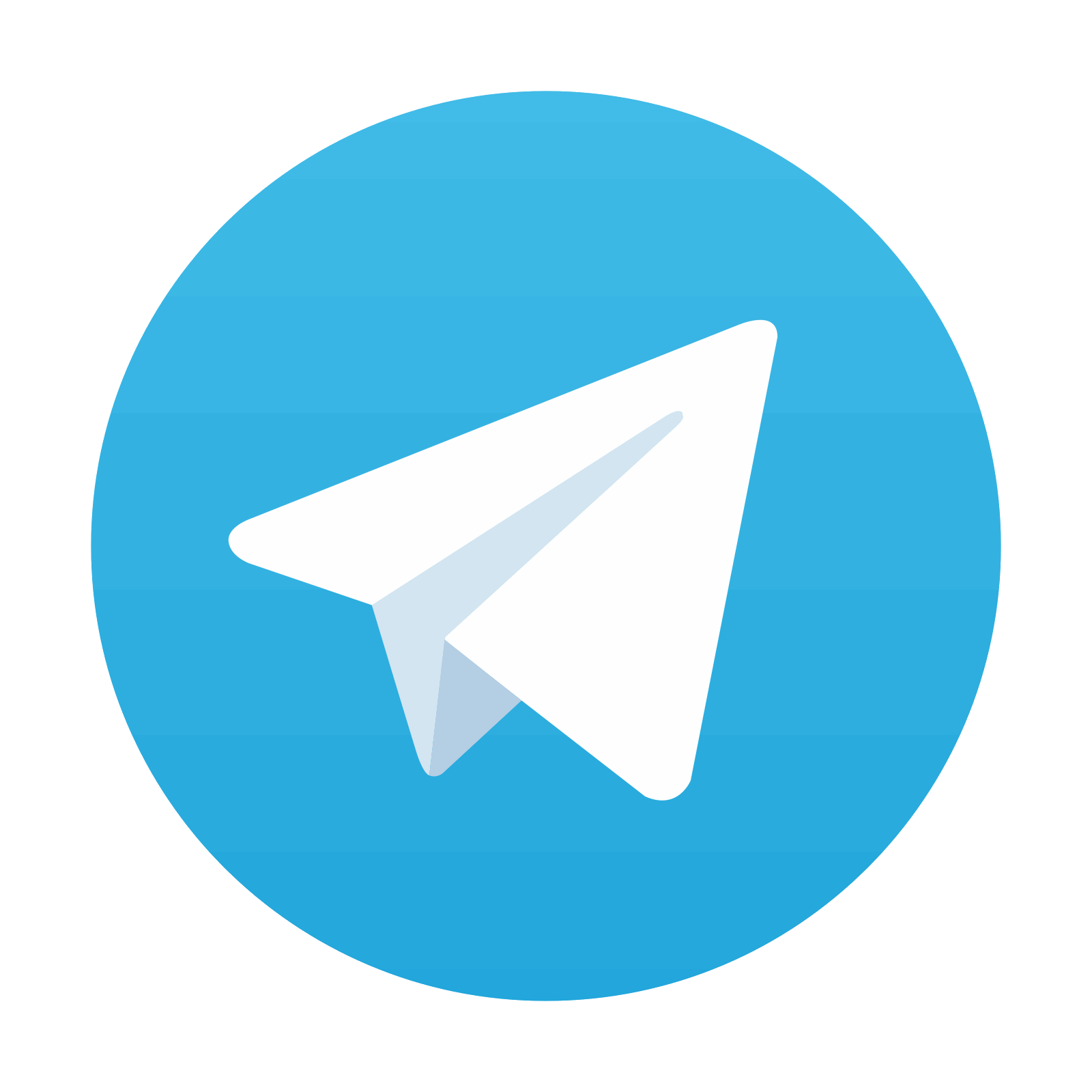
Stay updated, free articles. Join our Telegram channel

Full access? Get Clinical Tree
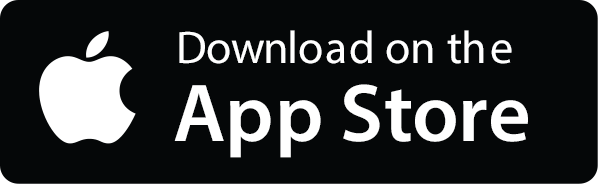
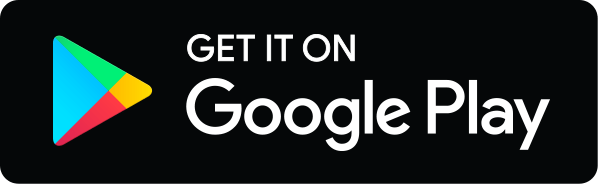