Assessment of Cardiac Transplantation
Mihai V. Podgoreanu
Joseph P. Mathew
Although Barnard performed the first human cardiac transplant in 1967, it was only by the early 1980s that it gained widespread acceptance as a realistic therapeutic option for patients with end-stage heart disease. The consistent advancements in donor management, surgical techniques, immunosuppressive therapy, and antibiotic therapy resulted in a dramatic growth of cardiac transplantation in the 1980s, and have led to successful heart-lung and lung transplantation. In 2001, the Registry of the International Society for Heart and Lung Transplantation listed a cumulative total of 57,818 heart transplants and 2,861 heart-lung transplants performed in 211 centers worldwide (1). The annual number of heart transplants reached a plateau in the mid- 1990s at approximately 4,500 per year, and has been declining in recent years (1). The limiting factor has been a shortage of suitable donors, further compounded by a tendency to relax the recipient selection criteria in an effort to extend the benefits of transplantation. As of January 2002, the United Network for Organ Sharing national cardiac transplant waiting list (www.unos.org) included 4,119 patients, whereas only 2,197 heart transplants were performed in the U.S. in the year 2000 (2). Although the majority of heart transplant recipients cluster between the ages of 35 and 64 years, 12.4% of all cardiac transplants in the year 2000 were performed in pediatric patients (< 18 years old) and 9.8% in patients 65 years and older, with the recipients being predominantly male (73.3%) and white (83.2%) (2). The most common indications for adult cardiac transplantation are coronary artery disease (46.1%) and cardiomyopathy (45.3%), with valvular heart disease and congenital heart disease contributing only 3.6% and 1.6%, respectively (1). The retransplantation rate in the year 2000 was 3.1% (1,2).
The overall one-year survival for cardiac transplantation is 80%, with a subsequent mortality rate of 4% per year (1). Risk factors for 1- and 5-year mortality in adult heart transplantation have been associated with recipient factors (repeat transplant, ventilator dependence, pulmonary vascular resistance, ischemic or congenital heart disease, ventricular assistance, older age, female gender, risk for primary cytomegalovirus infection, panel reactive antibody, body length, body mass index), medical center factors (volume of heart transplants performed, ischemic time), and donor factors (advanced age, female). Early mortality is most frequently due to primary nonspecific graft failure, intermediate-term deaths are caused by acute rejection or infection, whereas late deaths after cardiac transplantation are most frequently due to allograft vasculopathy, lymphoproliferative or other malignancies, and chronic rejection (1).
The expanding role of transesophageal echocardiography (TEE) in adult cardiac surgery has included its use as a perioperative diagnostic and monitoring technique during cardiac transplantation. There are currently five categories of applications of TEE in the assessment of cardiac transplantation:
1. Cardiac donor screening
2. Intraoperative monitoring in the pretransplantation period
3. Intraoperative evaluation of cardiac allograft function and surgical anastomoses in the immediate posttransplantation period
4. Management of early postoperative hemodynamic abnormalities in the intensive care unit
5. Postoperative follow-up studies of cardiac allograft function
THE ROLE OF TEE IN CARDIAC DONOR SCREENING
The chronic shortage of ideal donor hearts has led some cardiac transplant centers to liberalize the originally established donor selection criteria (3,4) to include older donors and marginally acceptable hearts, such that, of all the hearts transplanted in the year 2000, 11.5% were harvested from donors > 50 years old (1).
Echocardiography has become an integral component in the evaluation of potential cardiac transplant donors (5). This evaluation should be performed at a time when dosages of intravenous inotropic agents have been lowered to a minimum compatible with adequate blood pressure and cardiac output, and after adequate fluid resuscitation. The echocardiographic assessment allows for the inclusion of donor hearts demonstrating normal function in patients otherwise considered at risk for cardiac injury by clinical criteria (known chest trauma, prolonged hypotension, hemodynamic instability requiring high doses of catecholamines). Additionally, it can circumvent the need for costly and time-consuming direct surgical inspection or cardiac catheterization in potential donors with severely depressed cardiac function (5). However, transthoracic echocardiography (TTE) is technically inadequate in up to 29% of mechanically ventilated brain-dead potential donors (6). In these patients, TEE consistently allows for unobstructed tomographic imaging of the heart, becoming a safe and useful adjunct in the assessment of ventricular function, chamber sizes, valvular structure and function, and septal wall motion and integrity (6).
Brain death is associated with hemodynamic deterioration and biventricular dysfunction, which is usually reversible shortly after transplantation. Studies in potential clinical donors and in experimental animals have suggested that brain death can have major histopathological and functional effects on the myocardium, with very typical focal lesions consisting of petechial subendocardial hemorrhage, contraction bands, and coagulative myocytolysis. Although the mechanism of myocardial injury and contractile dysfunction after brain death remains incompletely understood, it is believed to be caused by a catecholamine excess that occurs during the process of brain death, resulting in cytosolic calcium overload (79). Previous studies have shown that segmental wall motion abnormalities and global left ventricular systolic dysfunction (fractional area change (FAC) < 50%) are frequent in brain-dead donors (67.5% and 36%, respectively), improve shortly after heart transplantation, and remain improved 15 months later (4,10) thus suggesting that potential cardiac donors should not be excluded on the basis of segmental wall motion abnormalities. A recent multi-institutional study, however, identified wall motion abnormalities on the donor echocardiogram as an independent powerful predictor of fatal early graft failure (relative risk of 1.7), especially with increasing donor age and prolonged ischemic time (11). As of yet, the lowest FAC enabling the heart to be transplanted without risk is not known, but one study recommends harvesting and heart transplantation when the FAC is above 35% if there are no other severe cardiac abnormalities (right ventricular failure or valvular dysfunction) (5). One retrospective study suggests that the presence of LVH in the donor heart increases the incidence of early graft dysfunction (12). Such marginal donor hearts should not be used in high-risk recipients (those of ventilator support, with prior sternotomies, or in renal failure, for example), and carefully monitored postoperatively for allograft dysfunction.
INTRAOPERATIVE MONITORING IN THE PRETRANSPLANTATION PERIOD
The vast majority of patients referred for cardiac transplantation suffers from ischemic or idiopathic cardiomyopathy with a dilated left ventricle and depressed ejection fraction. The currently accepted indications for transplantation, however, include impaired functional status (peak VO2 < 14ml/kg/min) and/or refractory hemodynamic decompensation, manifested as severe ischemia not amenable to revascularization, or recurrent symptomatic ventricular arrhythmias despite optimal medical management (13). As such, these patients have a relatively fixed low stroke volume and depend on appropriate preload and heart rate to maintain a marginal cardiac output. Due to the characteristics of the end-systolic pressure volume relationship in the myopathic heart, even mild increases in afterload can markedly decrease the stroke volume (14). Sympathetic tone is increased in patients with heart failure, leading to generalized vasoconstriction as well as salt and water retention. The combination of vasoconstriction and ventricular dilation result in a substantial increase in myocardial wall tension. Moreover, in patients with long-standing left-sided cardiac failure, right ventricular impairment may result by a process of ventricular interdependence, independent of neurohumoral or circulatory effects, and can be further compromised by elevations in pulmonary vascular resistance (15). Almost all cardiac transplant candidates will be maintained on a combination of vasodilators for afterload reduction (usually angiotensin-converting enzyme inhibitors, ACE-I), diuretics to minimize volume overload, and antiarrhythmics (usually amiodarone). This combination therapy was associated with a decrease in the overall 1-year mortality, as well as a decrease in the incidence of sudden death in end-stage heart failure (16). The average waiting time for patients at home is currently more than 18 months and continues to lengthen, but patients who develop refractory hemodynamic decompensation will require continued hospitalization for hemodynamic monitoring, prolonged inotropic therapy, or various degrees of mechanical assistance as a bridge to transplantation (intraaortic balloon counterpulsation, uni- or biventricular assist devices). In the United States, priority (Status I) is accorded to hospitalized patients requiring assist devices or intravenous inotropic therapy in
intensive care units; all other patients are Status II. In the year 2000, 56.6% of heart transplant recipients were reported to be on life support at the time of transplantation, of which 32.3% were in the intensive care unit (2).
intensive care units; all other patients are Status II. In the year 2000, 56.6% of heart transplant recipients were reported to be on life support at the time of transplantation, of which 32.3% were in the intensive care unit (2).
The main goal during the prebypass period is to maintain adequate end-organ perfusion. However, in these patients with end-stage heart failure, cardiovascular decompensation during induction or maintenance of anesthesia can result from multiple mechanisms. Decreases in sympathetic outflow (especially when the renin-angiotensin system is blocked by ACE-I) with resultant hypotension and impaired coronary perfusion, alterations in preload (hypovolemia from exaggerated diuresis), decreases in heart rate (with absent compensatory preload reserve), or increases in pulmonary vascular resistance can be extremely deleterious (14).
In these patients with precarious hemodynamic status, TEE is ideally suited to evaluate and guide intraoperative management decisions in the pretransplantation period. A complete TEE examination will often reveal information not readily available from other sources. Some examples follow.
Left Ventricular (LV) Volume
Due to a right shift in the left ventricular pressure-volume relationship, the failing heart requires a larger preload to maintain marginal performance. Moreover, superimposed diastolic dysfunction results in a poor correlation between LV filling pressures and volumes, further compounded by the effects of positive-pressure ventilation. Thus, optimization of left ventricular filling is best achieved by monitoring LV volumes under TEE guidance.
Left Ventricular Contractility
Performed in conjunction with the assessment of LV preload, monitoring global LV systolic function in the pretransplantation period may help achieve hemodynamic stability in the face of reductions in sympathetic outflow associated with induction of anesthesia, by guiding the adjustments in inotropic infusions.
Intracavitary Thrombus
Particular attention should be paid to the LV apex, the most common site for ventricular thrombus associated with cardiomyopathy or apical infarcts. The left atrial appendage should be inspected for possible thrombi, especially in atrial fibrillation. If intracavitary thrombi are identified, manipulation of the heart should be limited and dissection should proceed with great caution prior to cardiopulmonary bypass to avoid systemic embolization (Fig. 36.1).
Atherosclerosis of the Ascending Aorta and Aortic Arch
Aortic atheroma burden is assessed well by TEE, and should guide aortic cannulation site and cross-clamp placement. The ascending aorta and aortic arch can be assessed in the midesophageal aortic valve long-axis, ascending aortic short-axis and long-axis views, and in the upperesophageal aortic arch long-axis and short-axis views (18). The descending aorta is also assessed for the presence of mobile atheroma, in case an intraaortic balloon pump is required posttransplant. This can be achieved in the descending aortic short-axis and long-axis views (18).
Right Ventricular (RV) Dilation or Hypertrophy
Their presence would be suggestive of long-standing pulmonary hypertension, and should heighten the awareness for possible acute RV dysfunction in the transplanted heart.
Assessment of Left Ventricular Assist Device (LVAD) Explant
In patients who required mechanical circulatory assistance as a bridge to transplant, microperforations can occur at the time of LVAD explant, during the lengthy dissection onto the inlet cannula and its connections. This
can result in air entrainment and subsequent ejection into the aorta (17). TEE monitoring for such an event can be performed in the midesophageal aortic valve long-axis view (see Chapter XXX) (18).
can result in air entrainment and subsequent ejection into the aorta (17). TEE monitoring for such an event can be performed in the midesophageal aortic valve long-axis view (see Chapter XXX) (18).
Hemodynamic Calculations
Most cardiac anesthesiologists agree that a pulmonary artery catheter (PAC) is useful in the hemodynamic evaluation of patients undergoing cardiac transplantation. However, floating the PAC into correct position may be difficult due to cardiac chamber dilation and severe tricuspid regurgitation. Furthermore, PAC placement may be more prone to induce dysrhythmias, resulting in rapid hemodynamic decompensation in these patients with marginal cardiovascular reserve. Consequently, the PAC may be placed in a sterile sheath with the tip advanced to the end of the cordis introducer, during the prebypass period, and subsequently advanced by the surgeon under direct vision before the completion of the right atrial anastomosis. This minimizes the risk of blindly passing the PAC across fresh surgical suture lines. When a PAC cannot be placed, TEE can be used to determine the cardiac output and pulmonary artery pressures in the prebypass period.
The area-length formula can be used to calculate the stroke volume (SV) across a cardiac valve:
SV = cross-sectional area (CSA) × velocity time integral (VTI)
The SV across a valve can be subsequently used to calculate the cardiac output (CO) if there is no stenosis and/or regurgitation across that valve. Because most of these patients with dilated cardiomyopathies present with various degrees of mitral regurgitation, the aortic valve is usually used for CO measurements. The left ventricular outflow tract (LVOT) diameter is measured from the midesophageal aortic valve long-axis view; the LVOTVTI is measured from the LVOT Doppler spectrum in the transgastric long-axis or deep transgastric long-axis views, which provide windows for continuous wave Doppler interrogation, that is nearly parallel to aortic flow (18). The final formula for CO (in L/min) becomes:
CO = 0.785 (LVOT diameter)2 × LVOTVTI × heart rate/1000
This TEE determination of CO in the prebypass period should be performed even if the PAC has been successfully advanced into the pulmonary artery, because most of these patients present with various degrees of tricuspid regurgitation, which renders thermodilution cardiac output measurements inaccurate (19).
Doppler echocardiography is an ideal tool for determining pulmonary artery pressures. In the presence of tricuspid regurgitation (TR), the systolic pulmonary artery pressure (PAS) can be calculated using the simplified Bernoulli equation from the peak velocity of the TR jet (VTR, measured by continuous wave Doppler in the midesophageal right ventricular inflow-outflow view) (18), and the central venous pressure (CVP) as:
PAS = 4 (VTR)2 + CVP
In the presence of pulmonic regurgitation (PR), the diastolic pulmonary artery pressure (PAD) can be calculated in similar fashion, using the end-diastolic velocity of the PR jet (VPR, measured by continuous wave Doppler in the upperesophageal aortic arch short-axis view), and the CVP as:
PAD = 4 (VPR)2 + CVP
As with all Doppler measurements, the ultrasound beam must be parallel to the regurgitant flows or the velocities (hence the PA pressures) will be underestimated.
Intraoperative Monitoring in the Posttransplantation Period
The pathophysiology of the transplanted heart is dependent upon several factors, including the amount of donor inotropic support, the degree of subclinical myocardial damage, the ischemic time, the myocardial protection during the ischemic interval, cardiac denervation, and the degree of pulmonary hypertension in the recipient.
Assisting with Venting and De-Airing Maneuvers
Prior to weaning from cardiopulmonary bypass (CPB), TEE is used to detect retained intracardiac air and to assist the de-airing maneuvers. The most common sites of air retention are the right and left upper pulmonary veins, the LV apex, the left atrium, and the right coronary sinus of Valsalva (20). Reports of acute RV dysfunction caused by air embolization to the right coronary artery exist in the literature (21). Detection of echogenic material by TEE in the left atrium or left ventricle after the heart is allowed to fully eject, followed by RV dilatation, loss of contraction, and ST segment changes in inferior leads should raise the suspicion of right coronary air embolus. Full CPB should be reinstituted and the coronary perfusion elevated to allow the dissolution of the coronary embolus. Without the aid of TEE, the real cause of RV dysfunction in this scenario may be missed (see also “Assessment of the Right Ventricle”).
In the majority of cardiac transplantations, separation from CPB is readily achieved. After separation from cardiopulmonary bypass, TEE allows for the online assessment of biventricular function and flow dynamics across
the surgical anastomoses, with important diagnostic and prognostic implications.
the surgical anastomoses, with important diagnostic and prognostic implications.
Assessment of the Left Ventricle
The global and segmental LV systolic function should be assessed in the midesophageal four-chamber, two-chamber, and long-axis views, as well as in the transgastric mid short-axis, basal short-axis, and long-axis views (18). To minimize intra- and interobserver variability, the LV fractional area change should always be measured in the transgastric midpapillary muscle short-axis tomographic plane, by manually tracing the endocardial border, or defined with an automated border detection system. Intraoperative TEE assessment of allograft LV systolic function early after separation from cardiopulmonary bypass (CPB), in contrast to routinely measured hemodynamic variables, has been shown to better predict early requirements for inotropic and mechanical support, particularly in patients with longer ischemic times (22).
The following 2D-echocardiographic findings of the LV, which would be considered abnormal in the general population, are characteristic of transplant recipients:
An increase in LV wall thickness (especially the posterior and septal walls), LV mass, and LV mass index may be observed. It has been hypothesized to represent myocardial edema, resulting from the manipulation and transport of the heart (23,24,25).
Paradoxical or flat interventricular septal motion, and decreased interventricular septal systolic thickening compared to normals (31% vs. 44%) (23).
Because the donor heart is normal in size, it is typically smaller than the original dilated failing heart and, therefore, it is positioned more medially in the mediastinum and tends to be rotated clockwise. This usually necessitates using nonstandard transducer locations and angles for echocardiography. Residual fluid accumulations in the posterior pericardial space contribute to the common small postoperative pericardial effusions (30).
TABLE 36.1. Mitral Inflow Doppler Velocities | |||||||||||||||||||||||||||||||||||
---|---|---|---|---|---|---|---|---|---|---|---|---|---|---|---|---|---|---|---|---|---|---|---|---|---|---|---|---|---|---|---|---|---|---|---|
|
Assessment of LV Diastolic Function
The postischemic condition of the allograft decreases diastolic compliance of both ventricles, necessitating greater than normal filling pressures to adequately preload the heart. The echocardiographic assessment of LV diastolic function in the transplanted heart is complicated by the variability of the Doppler velocity profiles from the atrioventricular valves, which may be observed when the remnant recipient atria retain mechanical activity. This results in asynchronous atrial contractions, modifying the filling patterns of both ventricles (23,24,25,26,27). The beat-to-beat variations in transmitral (and tricuspid) diastolic velocities, assessed by pulsed wave Doppler, relate to the timing of contraction of the recipient atria within the cardiac cycle. Therefore, it is important to locate the recipient P waves within the donor cardiac cycle before making the required Doppler measurements. When the recipient P waves occur during late systole to mitral valve opening, the flow signals should not be used to measure diastolic indices. Recipient P waves occurring during this time frame decrease isovolumic relaxation time and pressure half time, and increase the mitral inflow peak Ewave velocity. If the recipient P waves occur anywhere from late diastole through midsystole, the flow signals may be used (23,24,26) (Table 36.1).
Several studies have demonstrated an abnormal transmitral flow pattern compatible with restrictive diastolic LV dysfunction in orthotopic heart transplant recipients (reduced late maximum flow velocity, increased early-tolate diastolic maximum flow velocity ratio) (25,28). Similarly, an abnormal pulmonary venous flow pattern, characterized by reduced peak flow velocity and reduced time velocity integral in the systolic phase with relatively enhanced flow during the diastolic phase has been observed (22,28). This decreased systolic to diastolic maximum pulmonary venous flow velocity ratio, found despite normal pulmonary capillary wedge pressures and associated with a reduced left atrial area change and a reduced mitral annulus motion is suggestive of left atrial dysfunction (25) secondary to altered atrial anatomy and dynamics
(29). Beat-to-beat variability of all pulmonary venous flow parameters was found to be higher in transplant recipients than in controls, especially in the systolic phase parameters (25).
(29). Beat-to-beat variability of all pulmonary venous flow parameters was found to be higher in transplant recipients than in controls, especially in the systolic phase parameters (25).
Therefore, mitral Doppler inflow analysis alone appears to be inadequate for the assessment of diastolic LV function in heart transplant recipients, as LV diastolic dysfunction cannot be differentiated from atrial dysfunction, and should be therefore corroborated with the analysis of pulmonary venous flow patterns. The assessment is further complicated by the various pacing modalities used in the perioperative period.
Assessment of the Right Ventricle
Etiology, Pathophysiology, and Diagnosis of Acute RV Dysfunction
Despite advances in perioperative management, ISHLT registry data shows that right ventricular (RV) dysfunction accounts for 50% of all cardiac complications and 19% of all early deaths in patients after heart transplantation (1). Acute RV dysfunction in heart transplant recipients is of multifactorial etiology, resulting either from an increase in pulmonary vascular resistance (PVR) in the recipient, or from a loss of contractility in the donor heart (9,31).
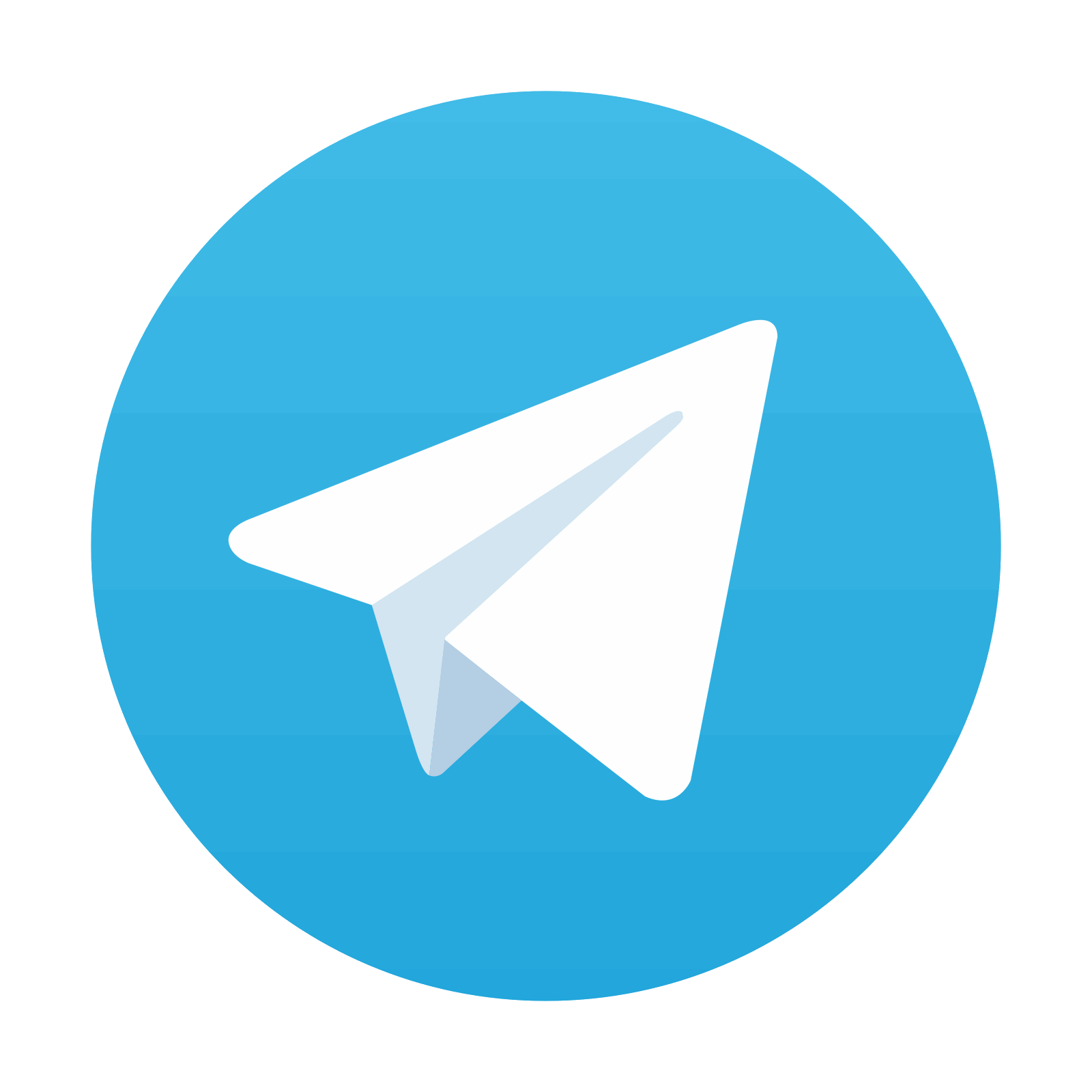
Stay updated, free articles. Join our Telegram channel

Full access? Get Clinical Tree
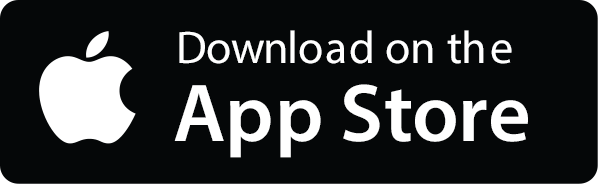
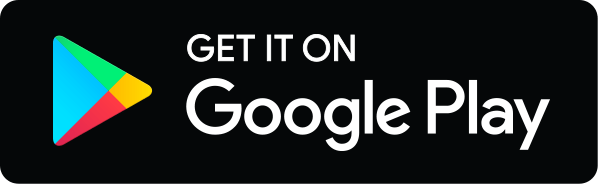
