Background
The goal of this study was to determine changes in left ventricular (LV) and right ventricular (RV) function with three-dimensional (3D) speckle-tracking echocardiography (STE) after percutaneous mitral valve repair with the MitraClip system in high-risk surgical patients with moderate to severe or severe secondary mitral regurgitation (MR).
Methods
Thirty-two patients with MR undergoing MitraClip were prospectively included. Patients underwent two-dimensional (2D) and 3D transthoracic echocardiography before clip implantation and after 6-month follow-up. LV and RV longitudinal strain was obtained by 2D STE and 3D STE. LV circumferential, radial, and area strain was calculated by 3D STE. Data analysis was performed offline.
Results
At 6-month follow-up, significant improvements were seen in LV 2D global longitudinal strain ( P < .005), 3D global longitudinal strain ( P = .0002), and 3D area strain ( P = .0001). Overall, significant improvements were also seen in 3D RV ejection fraction ( P < .05) and 3D RV free-wall longitudinal strain ( P < .05). A poor increase in LV strain after clip implantation ( P = NS) occurred in patients with pronounced preexisting RV dysfunction. The areas under the receiver operating characteristic curves for LV and RV 3D speckle-tracking echocardiographic parameters showed high discriminative values (range, 0.87–0.91) in predicting unfavorable outcomes with persistent symptoms (New York Heart Association class > II) after the procedure.
Conclusions
Three-dimensional STE showed overall LV and RV strain improvement after clip implantation as well as lower postprocedural LV strain values in patients with worse preexisting RV function. These findings could help in guiding MR treatment strategies, suggesting different therapies in the presence of marked RV impairment or anticipating the procedure in case of evolving RV dysfunction.
The MitraClip device (Abbott Laboratories, Abbott Park, IL) is a novel percutaneous system to treat mitral regurgitation (MR) in patients with left ventricular (LV) dysfunction and high risk for surgical mitral valve (MV) repair. The clip implantation over the leaflets creates a double-orifice valve and thus mimics Alfieri’s surgical procedure. The Endovascular Valve Edge-to-Edge Repair Study was the first randomized controlled study to show higher safety, lower clinical efficacy, and the same improvement in clinical outcome in comparison with surgical MV repair.
The hemodynamic benefit of the percutaneous procedure is usually higher in a particular subset of patients with low cardiac index values and high left-sided filling pressures. However, because the acute correction of MR could lead to further impairment in cardiac output and LV function, it would be useful to show noninvasively that LV performance is preserved and not decreased as a result of the acute reduction in MR. It also is known that patients with impaired right ventricular (RV) function after MV replacement have higher 5-year mortality than patients without preoperative right heart failure. The benefits of MV repair on reverse RV remodeling have been shown in patients with degenerative or functional MR using two-dimensional (2D) and three-dimensional (3D) echocardiography.
Two-dimensional speckle-tracking echocardiography (STE) represents a new means of evaluating myocardial wall movements and deformation, and the use of indexes derived from STE has been proposed as an adjunctive tool in the overall assessment of LV and RV function and is more sensitive compared with ejection fraction in detecting early changes in ventricular performance. The capability of 2D STE is limited by the changes in heart morphology during the cardiac cycle and the difficulty in tracking speckles in different frames because of out-of-plane motion. The newly developed 3D STE provides quick and comprehensive quantitative assessment of ventricular myocardial dynamics and has been applied in various pathologic conditions but never in MR after clip implantation. Accordingly, the goal of this analysis was to determine the changes in LV and RV function with 3D STE after percutaneous MV repair with the MitraClip system in high-risk surgical patients with functional MR.
Methods
Population
Thirty-two patients with moderate to severe or severe MR undergoing MitraClip were prospectively included. All patients had secondary (functional) MR from ischemic cardiomyopathy ( n = 20) or nonischemic cardiomyopathy ( n = 12). All of them presented with symptomatic MR despite optimal medical and device therapy and were evaluated by a multidisciplinary team composed of an echocardiologist, an interventional cardiologist, an anesthesiologist, and a cardiac surgeon. Coronary angiography was performed in all patients before clip implantation to rule out significant coronary artery disease. Patients were treated with percutaneous angioplasty before MitraClip procedure, if applicable. The group of control subjects comprised 32 healthy age- and sex-matched adults with no histories of systemic or cardiopulmonary disease, no known coronary risk factors, and normal electrocardiographic and echocardiographic results.
Transthoracic and transesophageal echocardiography was performed in all patients to assess quantitative MR analysis and morphologic suitability for clip implantation. Patients were selected for the procedure if they had moderate to severe or severe MR, met class I or II recommendations for surgery in chronic secondary MR, according to current guidelines, and were considered high risk for surgery. A logistic European System for Cardiac Operative Risk Evaluation score > 20 defined high risk. Patients were excluded if implantation was technically impossible because of valve morphology (endocarditis, mitral stenosis) or unlikely beyond the classic Endovascular Valve Edge-to-Edge Repair Study criteria, if they had undergone coronary percutaneous procedures or bypass surgery within the preceding 30 days, or if they had severe clinical comorbidities with very poor prognosis. Patients were informed about the risks of the procedure and possible alternative treatment, and they gave written consent. Two-dimensional and 3D transthoracic echocardiography was performed before clip implantation and after 6 months of follow-up. The study was approved by the institutional research committee.
Two-Dimensional and 3D Echocardiography
Patients were examined in the left lateral decubitus position using a Vivid E9 commercial ultrasound scanner(GE Vingmed Ultrasound AS, Horten, Norway) with phased-array transducers. Grayscale recordings were optimized at a mean frame rate of ≥50 frames/sec. Measurements of cardiac chambers were made by transthoracic echocardiography according to established criteria. LV mass was calculated using the Deveraux equation. LV peak systolic meridional wall stress was obtained from M-mode and pressure data with the following formula: LV peak systolic meridional wall stress = 0.86 × (0.334 × P × LV end-diastolic diameter)/[posterior wall thickness × (1 + posterior wall thickness/LV end-diastolic diameter)] − 27 × 10 3 dynes/cm 2 ), where P is systolic cuff blood pressure. MV orifice area was assessed using planimetry. RV systolic pressure was obtained using standard Doppler practices. Mitral annular velocities(S a , E a , and A a ) were measured in transthoracic four-chamber views.
MR severity was graded at baseline according to the criteria of the American Society of Echocardiography and the European Association of Cardiovascular Imaging. Semiquantitative indices of MR severity included color jet size, pulmonary venous flow velocity, and vena contracta width. Quantitative parameters were effective regurgitant orifice area (EROA), regurgitant volume (RegVol), and regurgitant fraction (RF). For inclusion in the study, MR severity was required to be moderate to severe or severe (3+ or 4+) using multiple criteria and an integrative approach. The vena contracta is the smallest, highest velocity region of the MR jet and was measured in a plane perpendicular to mitral leaflet closure, whenever possible. EROA was determined with the proximal isovelocity surface area (PISA) method by lowering the Nyquist aliasing velocity to identify the hemispheric shell, measuring the radius from orifice to first aliasing, and applying the standard formula on the basis of the ratio between flow rate (aliasing velocity × radius) and peak orifice velocity (regurgitant jet velocity by continuous-wave Doppler). PISA is more accurate for central jets than for eccentric jets. Moreover, PISA underestimates EROA in secondary MR because of noncircular orifice geometry. LV volumes were measured using the biplane method of disks. Forward stroke volume was calculated using LV outflow tract diameter and the velocity-time integral by pulsed-wave Doppler (LV outflow tract diameter 2 × 0.785 × velocity-time integral). RegVol was obtained by subtracting forward stroke volume from total stroke volume, where total stroke volume = LV end-diastolic volume − LV end-systolic volume. RF was calculated as RegVol/total stroke volume. According to current guidelines, in primary MR, the thresholds of severity for EROA, RegVol, and RF are 40 mm 2 (moderate to severe, 30–39 mm 2 ), 60 mL (moderate to severe, 45–59 mL), and 50% (moderate to severe, 40%–49%), respectively. In secondary MR, although this is a controversial step, lower cutoff values are recommended, 20 mm 2 for EROA and 30 mL for RegVol. RF remains unchanged at 50%. Although an integrative approach using multiple echocardiographic and clinical variables should be used to assess the severity of secondary MR, we used a matrix of criteria and classified MR as moderate to severe (3+) or severe (4+) if at least three of the six criteria described above were present and at least two of those three were quantitative.
After clip implantation, MR severity was graded according to the above parameters except for vena contracta width and PISA-derived EROA, which were not used because of the double-orifice valve, in line with previous studies.
A fully sampled matrix-array transducer with almost 3,000 active elements (4V-D; GE Vingmed Ultrasound AS) was used to acquire real-time 3D echocardiographic images in the LV apical four-chamber, two-chamber, and long-axis views. Acquisitions were recorded at the LV apex at a mean volume rate of ≥30 volume/sec and a multibeat acquisition to obtain correct spatial registration of all subvolumes and optimal temporal-spatial resolution. Within a single breath-hold lasting 6 to 8 sec and during a constant RR interval, four to six wedge-shaped subvolumes were required to acquire a full-volume data set. In patients with permanent atrial fibrillation, two or four cardiac cycles with ratios of the preceding to the pre-preceding interval (RR1/RR2) of approximately 1 were acquired for data set selection. Acquired 2D and 3D data were digitally transferred to a separate workstation for offline analysis of LV volumes using EchoPAC BT12 (GE Vingmed Ultrasound AS).
Two-Dimensional STE
LV 2D longitudinal strain ( Figure 1 ) was calculated in three apical views in relation to the strain value at aortic valve closure and measured in 17 segments on the basis of the software bull’s-eye diagram. Strain values were not derived in the presence of more than two suboptimal segments in a single apical view. Circumferential and radial systolic strain was calculated as an average of strain values obtained from the basal, middle, and apical parasternal short-axis views. The time rotation curves were displayed along the cardiac cycle. Counterclockwise rotation was conventionally marked as positive values and clockwise rotation as negative values when viewed from the LV apex. Peak apical and basal rotation and peak LV torsion were obtained. LV twist was defined as the net difference (in degrees) of apical and basal rotation at isochronal time points. LV torsion was normalized twist divided by LV ventricular diastolic longitudinal length between the apex and mitral plane.


To assess regional and global RV systolic function in the longitudinal direction, we adopted a six-segment RV model (basal lateral free wall, midlateral free wall, apical free wall, apical septum, midseptum, and basal septum). Peak systolic strain was recorded for the three RV myocardial free wall and septal segments and the entire RV wall ( Figure 1 B). Global strain and strain rate were calculated by averaging local strain values along the entire right ventricle using machine software.
Three-Dimensional STE
The original raw data from 3D data sets were analyzed on a separate software workstation (EchoPAC BT12, 4D Auto-LVQ; GE Vingmed Ultrasound AS). Alignment was performed with presentation of four-chamber, two-chamber, and three-chamber apical views, as well as short-axis views. For the end-diastolic volumes, the operator placed one point in the middle of the mitral annulus plane and a second point at the LV apex, generating an end-diastolic endocardial border tracing and including the papillary muscles within the LV cavity. For the end-systolic volumes, the same process was repeated in end-systole and, acquisition of LV volumes and LV ejection fraction (LVEF) was obtained. The correct alignment of the endocardial contours during the cardiac cycle was checked to obtain the volume waveform. A second semiautomated epicardial tracking was performed to delineate the region of interest for strain analysis (3D STE). Three-dimensional STE was used to determine at end-systole global longitudinal strain (GLS), global circumferential strain (GCS), global area strain (GAS), global radial strain (GRS), and torsion. GAS was determined as the percentage decrease in the size of endocardial surface area defined by the vectors of longitudinal and circumferential deformations. Following a frame-by-frame analysis, a final 17-segment bull’s-eye map of strain values was displayed ( Figure 1 C). Global strain values were automatically calculated by the software and were not determined in the presence of more than three uninterpretable segments.
Three-dimensional RV speckle-tracking analysis was performed after imaging the RV chamber in apical views by using a 4V phased-array transducer with high volume rate (≥30 image frames/sec) and six-beat acquisition. Three-dimensional GLS of the whole right ventricle (17 segments) was calculated using Echo PAC BT12 ( Figure 1 D). Three-dimensional GLS of the RV free-wall only (free wall longitudinal strain [FWLS]) was then calculated.
MitraClip Implantation
All procedures were performed using a 24-Fr clip device (CDS01 or CDS02; Abbott Vascular, Santa Clara, CA) under general anesthesia and fluoroscopic and transesophageal echocardiographic guidance. Transseptal puncture was performed through peripheral venous access using a Brockenbrough needle. A steerable guide catheter was advanced into the left atrium, the delivery system was then inserted, and the MitraClip device was implanted at the origin of the regurgitant jet perpendicular to the coaptation line. The clip was deployed when the effect of the implant on valve regurgitation was satisfactory. Hemostasis was achieved by femoral vein compression for 12 hours. Procedural success was defined as the implantation of at least one clip and reduction in the severity of regurgitation of at least one grade.
Follow-Up Data
Patients were regularly followed up after discharge and at 6 months after clip implantation. Clinical evaluation of New York Heart Association (NYHA) class, blood sampling, and 2D and 3D echocardiography were performed at follow-up visits. If patients were able, a 6-min walk test was performed. Quality of life was assessed with the Minnesota Living with Heart Failure Questionnaire. N-terminal pro–brain natriuretic peptide was measured both at baseline and at 6-month follow-up.
Statistical Analysis
Continuous data are expressed as mean ± SD and categorical data as absolute numbers and percentages. Paired t tests were used to compare differences noted in patients with MR and those noted in control subjects. Chi-square tests were applied for categorical variables. Linear regression analysis was used to estimate correlations between continuous variables. Differences were considered statistically significant when the P value was <.05. Receiver operating characteristic (ROC) curves were used to determine diagnostic accuracy for the prediction of unfavorable outcome (NYHA functional class > II). Intra- and interobserver variability of measurements was evaluated in 10 randomly selected patients. To analyze intraobserver variability, measurements of strain parameters were made at multiple sites in different patients on two different occasions. For interobserver variability, a second investigator randomly made measurements at the above different sites without knowledge of other echocardiographic parameters. Intraobserver and interobserver variability was determined as the difference between the two sets of observations divided by the mean of the observations and expressed as a percentage. For the assessment of inter- and intraobserver reproducibility of measurements, intraclass correlation coefficients were calculated, with good agreement defined by a coefficient > 0.80.
Results
Thirty-two patients who underwent clip implantation were included in the study. Forty-one patients were initially evaluated. Patients who did not show initial device success ( n = 1), those who had partial clip detachment without further implantation before discharge ( n = 2), and those who died before 6-month follow-up ( n = 2) were excluded. Four patients were excluded from the study because of inadequate myocardial tracking ( n = 2 on both 2D and 3D STE, n = 1 on only 3D STE) or rhythm abnormalities ( n = 1). Global STE feasibility in the subjects included in the study was 89% (32 of 36).
The clinical profile is summarized in Table 1 . The mechanism of MR was functional in all cases, with ischemic etiologies in 20 patients (62.5%). Most patients had several comorbidities, which contributed to the high mean logistic European System for Cardiac Operative Risk Evaluation score. All patients were symptomatic, with NYHA functional classes ≥ II, with the majority (90.6%) in class III or IV. NYHA functional class improved acutely at discharge (from 3.2 ± 0.6 to 2.5 ± 0.5, P < .005) and continued to improve progressively at 6 months (2.3 ± 0.7, P < .001). During follow-up, N-terminal pro–brain natriuretic peptide values decreased (from 5,199 to 3,349 pg/mL, P < .05), consistent with a clinical improvement in congestive heart failure. Quality of life was improved at 6 months, both the physical and mental components (the mean Minnesota Living with Heart Failure Questionnaire score decreased from 38 to 22, P < .01).
Variable | Baseline ( n = 32) | 6-mo follow-up ( n = 32) | P |
---|---|---|---|
Men/women | 18/14 | — | — |
Age (y) | 79.4 ± 5.5 | — | — |
BSA (m 2 ) | 1.88 ± 0.41 | — | — |
BMI (kg/m 2 ) | 26.2 ± 4.7 | — | — |
HR (beats/min) | 71 ± 14 | 69 ± 12 | NS |
SBP (mm Hg) | 124.2 ± 22.5 | 125.1 ± 23.2 | NS |
DBP (mm Hg) | 73.7 ± 12.9 | 72.8 ± 11.5 | NS |
NYHA functional class | 3.2 ± 0.6 | 2.3 ± 0.7 | <.001 |
NYHA functional class III/IV | 29 (90.6%) | 7 (21.9%) | <.001 |
Serum creatinine (mmol/L) | 142 ± 37 | 118 ± 32 | <.05 |
NT-proBNP (pg/mL) | 5,199 ± 4,048 | 3,349 ± 2,267 | <.05 |
6-min walk distance (m) | 192 ± 104 | 327 ± 116 ∗ | <.01 |
Quality of life (MLWHFQ score) | 38 (24–53) | 22 (10–42) | <.01 |
Logistic EuroSCORE (%) | 22.9 ± 10.4 | — | — |
Systemic arterial hypertension | 27 (84.3%) | — | — |
Coronary artery disease | 20 (62.5%) | — | — |
Permanent atrial fibrillation | 6 (18.7%) | — | — |
Pacemaker/ICD | 5 (15.6%) | — | — |
CRT | 3 (9.4%) | — | — |
Dyslipidemia | 23 (71.8%) | — | — |
Type 2 diabetes mellitus | 13 (40.6%) | — | — |
COPD | 3 (9.3%) | — | — |
Previous stroke | 4 (12.5%) | — | — |
Mitral Valve Results
One clip was implanted in 17 patients (53.1%), with two or three clips used in the remaining patients. In the majority of patients, MR reduction was obtained soon after placement of one or more clips. There was some degree of residual MR in nearly all patients, but its severity was mild or moderate ( Table 2 ). Residual MR was frequently located at A2/P2. No clinical or echocardiographic signs of significant mitral stenosis (mean MV pressure gradient ≥ 5 mm Hg, at a heart rate < 100 beats/min) were observed. A ≥10% increase in 3D LV GLS was found in 20 of 24 patients (83.3%) with residual MR < 2 and in one of eight patients(12.5%) with residual MR ≥ 2 ( P < .0005). Pulmonary arterial pressure was assessed according to the presence of residual MR after the procedure, and there was a significant increase in RV systolic pressure in patients with residual MR ≥ 2 compared with those with residual MR < 2 ( P < .05).
Variable | Control subjects ( n = 32) | Patients ( n = 32) | |||||
---|---|---|---|---|---|---|---|
Baseline | P ∗ | Discharge | P † | 6-mo follow-up | P ‡ | ||
LV | |||||||
LVEDD (mm) | 48.6 ± 4.3 | 64.9 ± 10.2 | <.0005 | 63.5 ± 12.3 | NS | 62.3 ± 11.7 | .03 |
LVESD (mm) | 29.4 ± 2.7 | 52.7 ± 12.7 | <.0001 | 51.6 ± 12.1 | NS | 49.2 ± 13.1 | .02 |
LVPWT (mm) | 8.7 ± 1.8 | 11.2 ± 0.6 | <.005 | 11.2 ± 0.9 | NS | 10.3 ± 0.8 | .017 |
LVMI (g/m 2 ) | 74.5 ± 18.6 | 191.3 ± 50.6 | <.0005 | 193.7 ± 52.3 | NS | 179.8 ± 51.7 | .04 |
LVPSWS (dynes/cm 2 ) | 155.3 ± 19.2 | 236.9 ± 51.7 | <.005 | 239.8 ± 58.1 | NS | 191.4 ± 56.8 | .01 |
LVEDV (mL) | 112.3 ± 19.6 | 182.9 ± 41.3 | <.0005 | 161.3 ± 40.8 | <.05 | 155.2 ± 40.2 | .02 |
LVESV (mL) | 41.2 ± 9.3 | 97.2 ± 34.3 | <.0005 | 84.2 ± 33.4 | <.05 | 79.5 ± 32.7 | .019 |
LVEF (%) | 64.3 ± 5.1 | 34.4 ± 15.2 | <.001 | 34.9 ± 17 .7 | NS | 37.3 ± 17.4 | <.05 |
MVOA (cm 2 ) | — | 5.1 ± 1.1 | — | 2.8 ± 0.9 | <.05 | 2.8 ± 0.7 | <.05 |
Annular DD (cm) | — | 4.2 ± 0.4 | — | 3.9 ± 0.3 | <.05 | 3.8 ± 0.3 | <.05 |
TSV (mL) | — | 89.2 ± 15.8 | — | 69.1 ± 12.7 | .004 | 67.3 ± 13.2 | .0018 |
FSV (mL) | — | 47.3 ± 12.6 | — | 48.7 ± 13.7 | NS | 52.8 ± 14.3 | <.05 |
RegVol (mL) | — | 44.2 ± 11.4 | — | 23.1 ± 9.2 | .004 | 20.3 ± 6.8 | .003 |
RF (%) | — | 48.1 ± 9.8 | — | 30.5 ± 11.1 | .006 | 27.4 ± 7.9 | .005 |
Mean MV-ΔP (mm Hg) | — | 2.2 ± 1.7 | — | 3.6 ± 2.1 | .003 | 3.6 ± 1.7 | .003 |
RVSP (mm Hg) | 21.7 ± 3.4 | 56.4 ± 17.2 | <.0005 | 48.9 ± 9.3 | .0037 | 46.2 ± 8.7 | .002 |
E/E′ | 6.9 ± 1.6 | 12.9 ± 1.2 | <.01 | 12.1 ± 1.1 | NS | 12.3 ± 0.8 | NS |
MR (grade 0–4) | — | 3.2 ± 0.6 | — | 1.6 ± 0.9 | .003 | 1.5 ± 0.8 | .0024 |
MR grade < 2 | — | 0 | — | 23 (71.8%) | <.0001 | 24 (75%) | <.0001 |
Jet/LA area ratio (%) | — | 43 ± 11 | — | 20 ± 13 | .002 | 20 ± 12 | .002 |
Vena contracta (cm) | — | 0.68 ± 0.14 | — | — | — | — | — |
EROA (mm 2 ) | — | 33.1 ± 13.8 | — | — | — | — | — |
RV | |||||||
RVFAC (%) | 49.3 ± 14.1 | 36.6 ± 9.4 | .004 | 37.3 ± 10.1 | NS | 37.3 ± 10.3 | NS |
TAPSE (mm) | 22.3 ± 5.4 | 15.4 ± 4.7 | .003 | 15.9 ± 5.1 | NS | 16.2 ± 4.9 | NS |
TR (grade 0–3) | 0.3 ± 0.2 | 1.9 ± 0.5 | <.01 | 1.7 ± 0.3 | NS | 1.7 ± 0.6 | NS |
∗ Baseline versus control subjects.
Left Ventricular and RV Data
The overall changes in LV and RV variables from baseline to follow-up are presented in Tables 2 and 3 . Following the procedure, LV forward stroke volume increased significantly, whereas total stroke volume showed a significant reduction. Early improvements was seen in 2D LV longitudinal strain, 3D LV longitudinal strain, and 3D LV GAS. Significant improvements were also seen in 3D RV ejection fraction (RVEF) and 2D and 3D RV strain ( Table 3 ). A positive correlation was obtained between 3D LVEF measured with 3D volumetric data and the same values determined using 3D STE ( r = 0.88, P < .0001). Postimplantation increases in 3D LV GLS and 3D LV GAS were seen in patients with nonischemic cardiomyopathy (3D LV GLS, −8.3 ± 2.8 vs −12.5 ± 3.1 [ P = .0027]; 3D LV GAS, −17.1 ± 4.1 vs −20.1 ± 4.5 [ P = .0021]) as well as in patients with ischemic cardiomyopathy (3D LV GLS, −8.6 ± 2.5 vs −12.9 ± 3.6 [ P = .0034]; 3D LV GAS, −17.6 ± 4.2 vs −20.8 ± 4.7 [ P = .0014]).
Variable | Control subjects ( n = 32) | Patients ( n = 32) | |||||
---|---|---|---|---|---|---|---|
Baseline | P ∗ | Discharge | P † | 6-mo follow-up | P ‡ | ||
LV | |||||||
3D echocardiography | |||||||
LVEDV (mL) | 125.3 ± 23.1 | 191.2 ± 44.7 | <.0005 | 169.1 ± 38.8 | .05 | 163.4 ± 39.2 | .002 |
LVESV (mL) | 64.3 ± 14.2 | 104.6 ± 33.4 | <.0005 | 89.1 ± 29.6 | .05 | 83.8 ± 30.1 | .001 |
LVEF (%) | 63.2 ± 5.3 | 33.4 ± 14.1 | .002 | 34.1 ± 16.9 | NS | 38.3 ± 15.1 | <.05 |
LAVI (mL/m 2 ) | 28 ± 2.6 | 53.7 ± 11.6 | .003 | 52.4 ± 12.9 | NS | 49.6 ± 12.3 | <.05 |
2D strain | |||||||
LV GLS (%) | −19.6 ± 2.4 | −9.7 ± 2.5 | <.0005 | −12.2 ± 4.4 | .05 | −14.3 ± 4.1 | <.005 |
LV GCS (%) | −27.6 ± 5.2 | −19.1 ± 4.4 | <.0005 | −20.1 ± 4.2 | NS | −24.2 ± 4.3 | <.05 |
LV GRS (%) | 34.7 ± 7.6 | 30.1 ± 7.4 | <.05 | 30.8 ± 8.2 | NS | 31.3 ± 8.5 | NS |
LVtor (°/cm) | 1.7 ± 0.4 | 0.9 ± 0.2 | <.05 | 1.0 ± 0.5 | NS | 1.1 ± 0.3 | NS |
3D strain | |||||||
LV GLS (%) | −18.1 ± 2.2 | −8.5 ± 2.6 | <.0005 | −12.3 ± 3.4 | .003 | −13.1 ± 3.7 | .0002 |
LV GCS (%) | −26.5 ± 5.1 | −16.2 ± 4.5 | <.0005 | −17.4 ± 4.6 | NS | −19.8 ± 4.2 | <.01 |
LV GRS (%) | 33.6 ± 8.7 | 26.8 ± 8.3 | .02 | 27.6 ± 7.6 | NS | 27.3 ± 7.9 | NS |
LV GAS (%) | −39.7 ± 4.9 | −17.4 ± 4.3 | <.0001 | −20.4 ± 4.7 | .001 | −22.8 ± 4.6 | .0001 |
LVtor (°/cm) | 1.6 ± 0.5 | 0.9 ± 0.5 | <.01 | 1.1 ± 0.6 | NS | 1.2 ± 0.6 | NS |
RV | |||||||
3D RVEF (%) | 53.6 ± 7.2 | 42.5 ± 7.6 | .0013 | 52.9 ± 8.3 | <.05 | 52.2 ± 7.9 | <.05 |
RV GLS (%) | −24.1 ± 3.6 | −19.3 ± 3.8 | <.01 | −20.8 ± 4.2 | NS | −21.6 ± 4.6 | .05 |
RV FWLS (%) | −25.9 ± 5.7 | −17.2 ± 4.1 | <.005 | −23.2 ± 3.9 | <.05 | −23.9 ± 3.7 | <.05 |
3D RV GLS (%) | −23.4 ± 3.9 | −18.7 ± 4.5 | <.01 | −20.7 ± 3.8 | NS | −21.8 ± 4.2 | .05 |
3D RV FWLS (%) | −23.8 ± 5.8 | −16.2 ± 1.9 | .002 | −19.4 ± 1.7 | <.05 | −19.1 ± 1.9 | <.05 |
∗ Baseline versus control subjects.
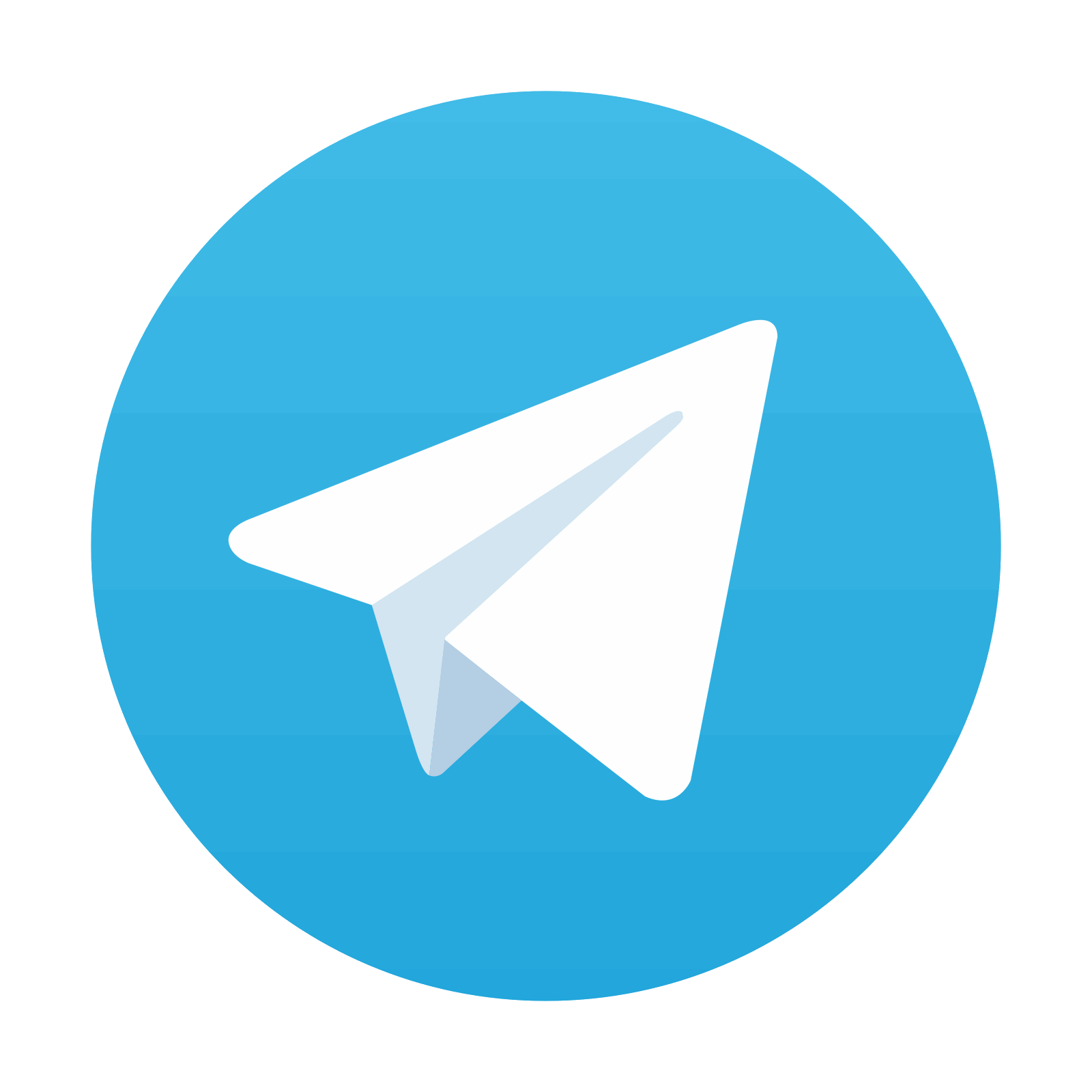
Stay updated, free articles. Join our Telegram channel

Full access? Get Clinical Tree
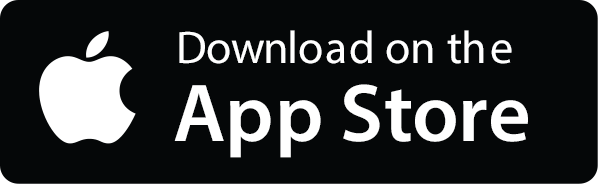
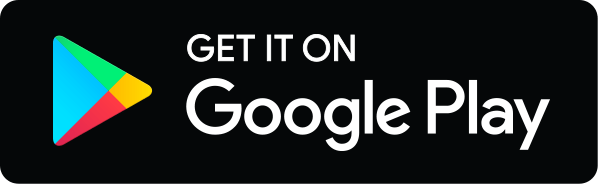