![]() FIGURE 28.3A. There are wide ranges of long-term survival and freedom from operation in patients with MR. Enriquez-Sariano et al. compared the cardiac morbidity and long-term survival at 5 and 10 years in patients diagnosed with flail MV leaflet to normal survival for age. There was over a 6.0% excess mortality per year, with a 5- and 10-year survival of 65% and 53%, respectively. Patients with ejection fractions < 50% had a 10-year survival of 32%. Over 90% of patients had either received surgical intervention or expired at the end of 10 years. Timing of mitral valve surgery. Br Heart J; 28:79-85, Jan. |
![]() FIGURE 28.4A. The 2003 Executive Summary of the Society of Thoracic Surgeons documents the continued growth of mitral valve surgery. More than 30% of all mitral valve surgeries since 1994 involved a reconstructive approach to MV dysfunction (34). The percentage of MVRep versus replacements continues to increase. |
![]() FIGURE 28.4B. The percentage of mitral valve repair procedures performed in patients undergoing isolated mitral valve surgery at the Cleveland Clinic has consistently increased since 1995. |
The first of these involves patients with preoperative mild to moderate MR or MS who are undergoing another cardiac surgical procedure and confirmation of the decision to avoid MV surgery is desired. In these circumstances, customary monitors are placed, prior to induction, to better understand the patient’s baseline hemodynamics. During the intraoperative assessment, hemodynamics similar to those recorded at a preoperative echocardiographic exam or just prior to induction are reproduced.
TABLE 28.1. Intraoperative Echocardiography Exam and Critical Issues in MV Surgery
1.
Confirm and Refine Preoperative Assessment
•
Confirm MV dysfunction and severity
•
Determine repairability of valve
•
Refine pathoanatomy and mechanism
•
Explain variances
2.
Determine the Need for Unplanned Surgical Intervention
•
Secondary pathophysiology
•
Associated abnormality
•
Unrelated process
3.
Determine Cardiac Dysfunction Impacting Management
•
Secondary pathophysiology
•
Dysfunction associated with primary etiology
•
Coexisting dysfunction
4.
Cannulation and Perfusion Strategy
5.
Address Surgical Procedure-Specific Issues
6.
Predict Complications
7.
Assess Surgical Results
•
Initial and ongoing patient management
•
Results of surgery
•
Complications
The second such scenario is significant MV dysfunction that was either undiagnosed or is more severe than was determined by the preoperative evaluation. When the MR is of surgical severity, adding a mitral procedure is often a good idea, as long as it does not prohibitively increase the surgical risk-to-benefit ratio.
The final situation is finding MR that is significantly less than expected or absent. When findings of the pre-CPB IOE suggest a change in the operative plan, considerable thought must be given to the reasons for this discrepancy. However, intraoperative conditions may underestimate the amount of MR present under “street conditions” that have led to the well-established plan to perform MV surgery. We often challenge such patients with afterload stress (volume loading and phenylephrine) to see if the underrepresentation of MR might be a transient or misleading issue. When the operative mission is changed substantially, it is helpful to contact the consultative clinicians who have been involved in the long-term management of the patient. These are also situations where it is advisable to use the most quantitative assessments of severity, such as those recommended by the ASE Nomenclature and Standards Committee Task Force on Native Valve Regurgitation (35).
The probability of successful MV repair
The necessity to perform other surgical interventions related to the patient’s secondary, associated, or coexisting cardiovascular dysfunction.
The assessment of the results of the surgical procedure
determination of the probability of certain post-CPB complications, and assessment of the results of surgical intervention. The information provided from the systematic IOE exam provides the intraoperative team with an up-to-date road map, which will guide the surgical decision-making process and direct the hemodynamic management of the patient throughout the operation (40).
The MVAp is a complex structure consisting of the fibrous cardiac skeleton, saddle-shaped mitral annulus, mitral valve leaflets, chordae, papillary muscles, and ventricular wall complex. Pathologic processes that lead to structural damage to the anatomic integrity of the components of the MVAp may result in mitral valve dysfunction.
The purpose of the intraoperative echocardiographic IOE exam is to confirm and refine the patient’s preoperative assessment as issues that are critical to their intraoperative management are resolved.
Mitral valve repair is the treatment of choice for MV dysfunction resulting from all etiologies because of its superior long-term survival, preservation of ventricular function, and greater freedom from thromboembolism, endocarditis, and anticoagulatant related complications.
For patients scheduled for elective MV surgery, the most significant surgical issues that the IOE exam assists in resolving include the repairability of the MV apparatus (MVAp), the necessity to perform other surgical interventions, and the postcardiopulmonary bypass assessment of the surgical procedure and complications.
The feasibility of MVRep is guided by the real-time assessment of the mitral valve apparatus and the mechanism of dysfunction by the IOE exam, in conjunction with the surgeon’s direct inspection of the MVAp.
The IOE exam is performed according to the unique demands of the cardiac surgical environment, with the pre-CPB and post-CPB exams organized by priority to ensure that critical issues are addressed should the patient require the initiation of CPB.
The pre-CPB IOE exam determines the severity and anatomic mechanism of MV dysfunction, in addition to assessing secondary or associated pathophysiology, cannulation-perfusion strategy, the potential for post-CPB complications, and providing an ongoing assessment of cardiac function.
The post-CPB IOE exam provides a quality assurance safety net with immediate assessment of the surgical procedure and diagnosis of complications related to the surgery or disease process.
The IOE exam relies upon integrated methods of severity assessment that may be efficiently performed in a multitasking environment. The methods included here are those recommended by the American Society of Echocardiography’s Task Force on Native Valvular Regurgitation. They have been validated by accepted standards to ensure their ability to reliably guide the intraoperative decision-making process.
The severity of mitral valve dysfunction is evaluated intraoperatively by the integration of multiple two-dimensional and Doppler parameters. The reliance on any one method of assessing severity is weighted by dependability of the specific data acquired and the quantitative reliability of a particular technique. Such an integrated approach minimizes the individual measurement error inherent to each.
![]() FIGURE 28.7A. Three-dimensional shape of MV annulus and with a three-dimensional annuloplasty ring. |
![]() FIGURE 28.7B. During systole, the circular annulus circumferentially narrows, becoming a 3-dimensional saddle-shaped annulus. This effectively decreases the size of the MV orifice. Fibrosis or calcification of the annulus interferes with its mobility and effectively increases the area requiring leaflet apposition to prevent regurgitant flow. |
![]() FIGURE 28.7D. The 3-dimensional shape of the annulus demonstrating the most superior (farthest from apex) points in the anterior-posterior orientation (ME LAX TEE plane). The most inferior (nearest the apex) points of the annulus are in the inferoseptal and anterolateral plane (ME Com TEE plane). Using the ME Com imaging plane will result in lower specificity in the diagnosis of MV prolapse or excessive leaflet motion. |
The mitral leaflets are attached to the fibrous annulus and to the free wall of the ventricle via papillary muscles and the primary edge and secondary midvalve chordae (43,44,45). The anterior mitral leaflet (AMVL) is triangular in shape and attached to the fibrous body at the left coronary cusp and anterior half of the noncoronary cusp of the aortic valve. The AMVL comprises about 55% to 60% of the total MV area and about 30% of the annular circumference (42,43). The posterior leaflet comprises 40% to 45% of the MV area and attaches to the mitral annulus posteriorly (Fig. 28.8) (41,42). As the heart normally lies in the chest cavity, the PMVL height (length) is usually less than the height of the AMVL (Fig. 28.9). During systole, the leaflets come together along a “line of coaptation,” which extends anteriorly to the anterolateral commissure and posteriorly to the posteromedial commissure (Fig. 28.10). During diastole, the middle of the leaflet initially moves toward the ventricle followed by opening of the valve at the leaflet edges (44,48) (Fig. 28.11). The middle of the leaflet opens before the commissures. Once the leaflet extends fully it may flutter and drift upwards until the atrial contraction. The surface of each leaflet is divided into a rough zone (coapting surface where primary and secondary chords attach), clear zone (midportion of leaflet, secondary chordae attachments), and basal zone (leaflet attachment to the annulus, insertion of posterior tertiary chordae) (49). The basal two-thirds of each leaflet are smoother than the distal third. The combined surface area of the mitral leaflets is twice that of the mitral orifice. This permits large areas of coaptation with a normal 3 mm to 5 mm of residual leaflet apposition distal to the point of coaptation (43,44,45). This line of coaptation between the two leaflets is semicircular and influences the segments of the valve leaflets, which are visualized in the standard imaging planes (Fig. 28.12). There is a range of mitral commissural orientations due to variation in the degree of annular size and rotation of the heart caused by individual variations and enlargement of chambers. Consequently, the commissure may be oriented more clockwise in some individuals, explaining the variability of segmental mitral anatomy visualized at the same transducer rotation in different patients (Fig. 28.13). The posterior MV leaflet consists of three scallops that are separated by prominently distinct indentations called clefts (Fig. 28.14). These scallops are referred to as lateral, middle, and medial. The lateral scallop is closest to the left atrial appendage (43,45). The anterior leaflet, for purely descriptive purposes, is segmented into the corresponding lateral, middle, and medial thirds.
![]() FIGURE 28.8. The MV has two leaflets referred to as the anterior and posterior leaflets (AMVL and PMVL). The mitral leaflets are circumferentially attached to the fibrous annulus. The anterior mitral leaflet (AMVL) is attached to the same fibrous body as the left coronary cusp (→). The anterior mitral valve leaflet (AMVL) comprises about 55% to 60% of the total MV area and about 30% to 40% of the annular circumference (—). The posterior leaflet comprises 40% to 45% of the MV area, and 60% to 70% of the circumference attaches to the mitral annulus posteriorly. |
![]() FIGURE 28.14. The posterior MV leaflet consists of three scallops that are separated by prominently distinct indentations called clefts (→). These scallops are referred to as the (antero) lateral, middle, and (postero) medial. The (antero) lateral scallop is closest to the left atrial appendage. The anterior leaflet, for purely descriptive purposes, is segmented into the corresponding lateral, middle, and medial thirds. |
![]() FIGURE 28.15. Chordae are fibrous tendon-like attachments extending from the leaflet to the papillary muscles and posterior ventricular wall. During systole, the papillary muscles contract and keep the chordae taut, thereby preventing prolapse of the leaflets into the left atrium. Tandler and Quain classified chordae as first, second, and third order. The first order attach to the leaflet edges adjacent to the commissure. Secondary rough zone chordae insert 8 mm from the free margin. There are two dominant secondary chordae (strut or stay chordae) going to the medial and lateral halves of the AMVL. Third order basal chordae only extend to the base of the PMVL. And maintain annular ventricular relation during the cardiac cycle. |
![]() FIGURE 28.16. Originating from the anterolateral and posteromedial walls of the left ventricle (between the middle and apical segments) are the two papillary muscles called by their segmental ventricular origin: anterolateral papillary muscle (ALPM) and posteromedial papillary muscle (PMPM). These papillary muscles run parallel to the adjacent ventricular wall. The larger ALPM usually has one or two heads whereas the smaller PLPM may have two or three. Chordal tendons from the head of each papillary muscle attach to both of the MV leaflets. The chordae that arise from the anterolateral PM extend to the lateral halves of the posterior and anterior MV leaflets. Consequently the ALPM and PMPM subtend and support their respective commissure (49). |
segments of the left ventricle in the region near the interface between the middle and apical thirds of the ventricular chamber (41,44,51). The mechanical tensor function of the MVAp is maintained through the continuity that the ventricular walls provide in connecting the papillary muscles with the MV annulus (41). If there is scarring in the anterolateral or posteromedial free walls of the left ventricle, this may result in traction on the MV annulus and deformity throughout the cardiac cycle. This may lead to restriction of the PMVL during systole, with a resulting override of the AMVL and a posteriorly directed regurgitant jet.
![]() FIGURE 28.17. Originating from the anterolateral and posteromedial walls of the left ventricle (between the middle and apical segments) are the two papillary muscles called by their segmental ventricular origin: anterolateral papillary muscle (ALPM) and posteromedial papillary muscle (PMPM). These papillary muscles run parallel to the adjacent ventricular wall. The larger ALPM usually has one or two heads, whereas the smaller PLPM may have two or three. Chordal tendons from the head of each papillary muscle attach to both of the MV leaflets. The chordae that arise from the anterolateral PM extend to the lateral halves of the posterior and anterior MV leaflets. Consequently the ALPM and PMPM subtend and support their respective commissure. |
posterior leaflet as P1 (lateral and closest to the left atrial appendage), PM (middle), and P2 (medial and adjacent to the posteromedial commissure) (Figs. 28.17, 28.18, 28.19 and 28.20) (53). The middle scallop (PM) is further subdivided into the PM1 and PM2, corresponding to the portion of the middle scallop that receives chordae from the anterolateral (M1) and posteromedial (M2) papillary muscles. The anterior leaflet is divided into two areas, A1 and A2, corresponding to the areas subtended by the corresponding chordal attachments from the anterolateral (M1) and posteromedial (M2) papillary muscles (53). In addition, the two commissural areas of the valve are defined as C1 (anterolateral, between A1 and P1) and C2 (posteromedial, between A2 and P2).
![]() FIGURE 28.18. There are three segmental nomenclatures used to describe the anatomy of the MV apparatus: the ASE-SCA (Carpentier adoption), the anatomic, and the Duran. The American Society of Echocardiography (ASE) Nomenclature and Standards Committee of the ASE and the Society of Cardiovascular Anesthesiologists (SCA) adopted the Carpentier system for standardizing the segmental leaflet nomenclature. The Duran nomenclature is an extremely valuable contribution to our understanding of the MV apparatus because it is established on the chordal distribution between the papillary muscles and the valve leaflet segments. SCA-ASE standardized nomenclature: P = PMVL segments (P1 = lateral scallop, P2 = middle scallop, P3 = medial scallop). A = AMVL segments (A1 = lateral segment, A2 = middle segment, A3 = medial segment). Chordae, commissures use anatomic descriptors (PMPM = posteromedial papillary muscle, ALPM = anterolateral papillary muscle) |
![]() FIGURE 28.19. The SCA-ASE nomenclature (Carpentier) defines the three scallops of the posterior leaflet as P1 (lateral), P2 (middle), and P3 (medial). The P1 (lateral) scallop is adjacent to the anterolateral commissure and is closest to the left atrial appendage. The P3 scallop is adjacent to the posteromedial commissure. This nomenclature defines the three corresponding areas of the anterior leaflet and A1 (opposite P1), A2 (opposite P2), and A3 (opposite P3). Originating from the anterolateral and posteromedial walls of the left ventricle (between the middle and apical segments) are the two papillary muscles called by their segmental ventricular origin, anterolateral papillary muscle (ALPM) and posteromedial papillary muscle (PMPM). These papillary muscles run parallel to the adjacent ventricular wall. The larger ALPM usually has one or two heads, whereas the smaller PLPM may have two or three. Chordal tendons from the head of each papillary muscle attach to both of the MV leaflets. The chordae that arise from the anterolateral PM extend to the lateral halves of the posterior and anterior MV leaflets. The ALPM and PMPM subtend and support their respective commissure (49). |
![]() FIGURE 28.20. The Duran nomenclature system is based on the chordal distribution and refers to the three scallops of the posterior leaflet as P1 (lateral and closest to the left atrial appendage), PM (middle), and P2 (medial and adjacent to the posteromedial commissure) (53). The middle scallop (PM) is further subdivided into the PM1 and PM2, corresponding to the portion of the middle scallop that receives chordae from the anterolateral (M1) and posteromedial (M2) papillary muscles. The anterior leaflet is divided into two areas, A1 and A2, corresponding to the areas subtended by the corresponding chordal attachments from the anterolateral (M1) and posteromedial (M2) papillary muscles. In addition, the two commissural areas of the valve are defined as C1 (anterolateral, between A1 and P1) and C2 (posteromedial, between A2 and P2). |
![]() FIGURE 28.24. Standard image planes and their variations are obtained by manipulations of the TEE probe, including advancing or withdrawing, turning right (clockwise) and left (counterclockwise), rotating the transducer angulations forward or back, and flexing the probe to the right or left. |
TABLE 28.2. Correlation of Image Plane and Cardiac Anatomy | |||||||||||||||||||||||||||||||||||||||||||||||||||||||||||||||||||||||||||||
---|---|---|---|---|---|---|---|---|---|---|---|---|---|---|---|---|---|---|---|---|---|---|---|---|---|---|---|---|---|---|---|---|---|---|---|---|---|---|---|---|---|---|---|---|---|---|---|---|---|---|---|---|---|---|---|---|---|---|---|---|---|---|---|---|---|---|---|---|---|---|---|---|---|---|---|---|---|
|
![]() FIGURE 28.25 A and B. Midesophageal Five Chamber MV (ME 5 Chr MV). Variation of ME 4 Chr MV; Transducer Depth: 30 cm; Probe Manipulation: Withdraw from ME 4 Chr MV. A: The TEE probe is slowly withdrawn 1-2 cm from the ME 4 Chr imaging plane to obtain the ME 5 Chr view. The LE 4 Chr imaging plane is obtained by advancing the probe 1-2 cm from the ME 4 Chr plane. B: This imaging plane cuts through the left ventricular outflow tract. Depending on the orientation of the MV commissure and transducer rotation angle, the 2-D plane may pass through the A1 segment, anterolateral commissure, and P1 segments of the MV. |
![]() FIGURE 28.26. Four Chamber MV (LE Four Chr MV). Variation of ME 4 Chr transducer; Depth: 32 cm; Probe Manipulation: Advance depending on the orientation of the MV commissure and transducer rotation angle. The 2-D plane may pass through the A2 segment, middle commissure, and the P2 scallop of the MV. The height of the P2 and A2 may be measured in this plane for comparison with the ME LAX MV measurements. |
![]() FIGURE 28.27. Lower-esophageal Four Chamber MV (LE Four Chr MV). Variation of ME 4 Chr transducer; Depth: 32 cm; Probe Manipulation: Advance depending on the orientation of the MV commissure and transducer rotation angle. The 2-D plane may pass through the A3 segment, posteromedial commissure, and the P3 scallop of the MV. |
![]() FIGURE 28.28. Midesophageal Commissural MV (ME Com MV). Transducer Depth: 30 cm: Transducer Rotation 45-70° A: The ME Com MV is obtained by rotating the transducer forward to 45-70°. Turning the probe to the right (clockwise) and to the left (counterclockwise) enable visualization of the variations of this imaging plane. B: Depending on the orientation of the MV commissure and transducer rotation angle, the 2-D plane may cut through the P3 scallop, the posteromedial commissure, tip of A2, anterolateral commissure, and the P1 segments of the MV. |
![]() FIGURE 28.29. Midesophageal Commissural Right MV (Probe Turned Right) (ME ComR MV). Variation of ME Com; Transducer Depth: 30 cm; Probe Manipulation: Turn left or counterclockwise; Transducer Rotation: 45-70°. Depending on the orientation of the MV commissure and transducer rotation angle, the 2-D plane may pass through the P3, A3, A2, and A1 segments. |
![]() FIGURE 28.30. Midesophageal Commissural Left MV (Probe Turned Left) (ME ComL MV): Variation of ME Com; Transducer Depth: 30 cm; Probe Manipulation: Turn left or counterclockwise; Transducer Rotation: 45-70°. Depending on the orientation of the MV commissure and transducer rotation angle, the 2-D plane may pass through the P3, P2, and P1 scallops. |
![]() FIGURE 28.31. Midesophageal Two Chamber MV (ME 2 Chr MV). Transducer Depth: 30 cm; Probe Manipulation: Midline; Transducer Rotation: 80-110°. A: The ME 2 Chr imaging plane is obtained by rotating the transducer forward to 80-110°. Variations of this plan are obtained by turning the probe to the right and left. B: Depending on the orientation of the MV commissure and transducer rotation angle, the 2-D plane may cut through the P3 scallop, the posteromedial commissure, the A3 segment, the A2 segment, and the A1 segment. |
plane may cut through the P2 scallop, the midcommissure, and through the A2 segment. On the monitor (proceeding from left to right) are P2, the midcommissure, and the A2 segment of the MV. The aortic valve is seen of the right of the screen. The MV annulus to the left of the screen and to the right—adjacent to the aortic valve—are the most superior (farthest from the apex) aspects of the annulus. A plane drawn between these points provides a more specific reference for diagnosing MV prolapse.
![]() FIGURE 28.32. Midesophageal Two Chamber Right MV (Turned Right) (ME 2 ChrR MV). Variation of ME 2 Chr MV; Transducer Depth: 30 cm; Transducer Rotation: 80-110°; Probe Manipulation: Turn right (clockwise). Depending on the orientation of the MV commissure and transducer rotation angle, the 2-D plane may cut through the P3 scallop, the apex of the posteromedial commissure, the A3 segment, and base of the A2 segment. |
![]() FIGURE 28.33. Midesophageal Two Chamber Left MV (Turned Left) (ME 2 ChrL MV). Variation of ME 2 Chr MV; Transducer Depth: 30 cm. Probe Manipulation: Turn left or counterclockwise; Transducer Rotation: 80-110°. Depending on the orientation of the MV commissure and transducer rotation angle, the 2-D plane may cut through the P3, P2, and P1 scallops. The commissure may not be visualized except during diastole. |
![]() FIGURE 28.34. Midesophageal Long Axis MV (ME LAX MV). Transducer Depth: 30 cm; Transducer Rotation: 110-150°. A: The ME LAX MV imaging plane is obtained by rotating the transducer forward to 110-150°. Variations of this plan are obtained by turning the probe to the right and left. B: Depending on the orientation of the MV commissure and transducer rotation angle, the 2-D plane passes through the minor axis of the MV annulus and aortic valve. The 2-D plane may cut through the P2 scallop, the midcommissure, and through the A2 segment. Height of the PMVL, AMVL, and C-sept are measured in this plane and compared to the ME 4 Chr MV measurements. This plane demonstrates the most superior aspects of the MV annulus. |
![]() FIGURE 28.35. Midesophageal Long-Axis Right MV (Probe Turned Right) MV ME LAXR MV. Variation ME LAX MV; Transducer Depth: 30 cm; Probe Manipulation: Turn right clockwise; Transducer Rotation: 110-150°. Depending on the orientation of the MV commissure, transducer rotation angle, and extent of probe manipulation, the 2-D plane may cut through the P2 scallop, the posteromedial commissure, and P3 scallops. The commissure may not be visualized except during diastole. |
![]() FIGURE 28.36. Midesophageal Long-Axis Left MV (ME LAXL MV). Variation ME LAX MV; Transducer Depth: 30 cm Probe Manipulation: Turn left or counterclockwise; Transducer Rotation; 110-150°. Depending on the orientation of the MV commissure, transducer rotation angle, and extent of probe manipulation, the 2-D plane may cut through the P2 scallop, the anterolateral commissure, and P1 scallops. The commissure may not be visualized except during diastole. |
![]() FIGURE 28.37. Transgastric Basal Short Axis (TG SAXB). Transducer Depth: 35 cm; Transducer Rotation: 0-5°. In this imaging plane, the AMVL (A1-2-3, bottom to top) is visualized on the left and the PMVL (P1-2-3, bottom to top) is visualized on the right. The clear space to the left of the AMVL edge and toward the bottom of the screen is the left ventricular outflow tract (LVOT), which is shaded in gray. Systolic turbulence in this area suggest SAM with LVOTO. |
TABLE 28.3. Principles of Intraoperative Echo Exam in Mitral Valve Surgery | |||||||||||||||||||||||||||||||||||||||||||||||||||
---|---|---|---|---|---|---|---|---|---|---|---|---|---|---|---|---|---|---|---|---|---|---|---|---|---|---|---|---|---|---|---|---|---|---|---|---|---|---|---|---|---|---|---|---|---|---|---|---|---|---|---|
|
![]() FIGURE 28.38. Transgastric Two Chamber (TG 2 Chr). Transducer Depth: 35 cm; Transducer Rotation 70-90°. This image is similar to the ME 2 Chr imaging plane except that the ventricle is horizontally oriented. Depending on the orientation of the MV commissure and transducer rotation angle, the 2-D plane may cut through the P3 scallop, the posteromedial commissure, the A3 segment, the A2 segment, and the A1 segment. |
TABLE 28.4. Systematic Intraoperative Echo Exam in MV Surgery | ||||||||||||||||||||||||||||||||||||||||||||||||||||
---|---|---|---|---|---|---|---|---|---|---|---|---|---|---|---|---|---|---|---|---|---|---|---|---|---|---|---|---|---|---|---|---|---|---|---|---|---|---|---|---|---|---|---|---|---|---|---|---|---|---|---|---|
|
TABLE 28.5. The Precardiopulmonary Bypass (Pre-CPB) Exam | ||||||||||||||||||||||||||||||||||||||||||||||||||||||||||||||||||||||||||||||||||||||||||||||||||||||||||||||||||||||||||||||||||||||||||||||||||||||||||||||||||||||||||||||||||||||||||||||||||||||||||||||||||||||||||||||||||||||||||||||||||||||||||||||||||||||||||||||||||||
---|---|---|---|---|---|---|---|---|---|---|---|---|---|---|---|---|---|---|---|---|---|---|---|---|---|---|---|---|---|---|---|---|---|---|---|---|---|---|---|---|---|---|---|---|---|---|---|---|---|---|---|---|---|---|---|---|---|---|---|---|---|---|---|---|---|---|---|---|---|---|---|---|---|---|---|---|---|---|---|---|---|---|---|---|---|---|---|---|---|---|---|---|---|---|---|---|---|---|---|---|---|---|---|---|---|---|---|---|---|---|---|---|---|---|---|---|---|---|---|---|---|---|---|---|---|---|---|---|---|---|---|---|---|---|---|---|---|---|---|---|---|---|---|---|---|---|---|---|---|---|---|---|---|---|---|---|---|---|---|---|---|---|---|---|---|---|---|---|---|---|---|---|---|---|---|---|---|---|---|---|---|---|---|---|---|---|---|---|---|---|---|---|---|---|---|---|---|---|---|---|---|---|---|---|---|---|---|---|---|---|---|---|---|---|---|---|---|---|---|---|---|---|---|---|---|---|---|---|---|---|---|---|---|---|---|---|---|---|---|---|---|---|---|---|---|---|---|---|---|---|---|---|---|---|---|---|---|---|---|---|---|---|---|---|---|---|---|---|---|---|---|---|---|---|---|---|
|
constitute the complete systematic examination, are achieved for future retrieval for comparison and reviewed under an organized CQI process as recommended by the Intraoperative Council of the American Society of Echocardiography (29).
TABLE 28.6A. Preseparation (Pre-Sep Examination | |||||||||||||||||||||||||||||||||||||||||||||||||||||||||||||||||||||||||||||||||||||||||||||||||||||||||||||||||||||||||||||||||||||||||||||||||||||||||||||||||||||||||||||||||||||||||
---|---|---|---|---|---|---|---|---|---|---|---|---|---|---|---|---|---|---|---|---|---|---|---|---|---|---|---|---|---|---|---|---|---|---|---|---|---|---|---|---|---|---|---|---|---|---|---|---|---|---|---|---|---|---|---|---|---|---|---|---|---|---|---|---|---|---|---|---|---|---|---|---|---|---|---|---|---|---|---|---|---|---|---|---|---|---|---|---|---|---|---|---|---|---|---|---|---|---|---|---|---|---|---|---|---|---|---|---|---|---|---|---|---|---|---|---|---|---|---|---|---|---|---|---|---|---|---|---|---|---|---|---|---|---|---|---|---|---|---|---|---|---|---|---|---|---|---|---|---|---|---|---|---|---|---|---|---|---|---|---|---|---|---|---|---|---|---|---|---|---|---|---|---|---|---|---|---|---|---|---|---|---|---|---|---|
|
TABLE 28.6B. Postseparation (Post-Sep) CPB Exam | ||||||||||||||||||||||||||||||||||||||||||||||||||||||||||||||||||||||||||||||||||||||||||||||||||||||||||||||||||||||||||||||||||||||||||||||||||||||||||||||||||||||||||||||||||||||||||||||||||||||||||||||||||||||||||||||||||||||||||||||||||||||||||||||||||||||||||||||||||||
---|---|---|---|---|---|---|---|---|---|---|---|---|---|---|---|---|---|---|---|---|---|---|---|---|---|---|---|---|---|---|---|---|---|---|---|---|---|---|---|---|---|---|---|---|---|---|---|---|---|---|---|---|---|---|---|---|---|---|---|---|---|---|---|---|---|---|---|---|---|---|---|---|---|---|---|---|---|---|---|---|---|---|---|---|---|---|---|---|---|---|---|---|---|---|---|---|---|---|---|---|---|---|---|---|---|---|---|---|---|---|---|---|---|---|---|---|---|---|---|---|---|---|---|---|---|---|---|---|---|---|---|---|---|---|---|---|---|---|---|---|---|---|---|---|---|---|---|---|---|---|---|---|---|---|---|---|---|---|---|---|---|---|---|---|---|---|---|---|---|---|---|---|---|---|---|---|---|---|---|---|---|---|---|---|---|---|---|---|---|---|---|---|---|---|---|---|---|---|---|---|---|---|---|---|---|---|---|---|---|---|---|---|---|---|---|---|---|---|---|---|---|---|---|---|---|---|---|---|---|---|---|---|---|---|---|---|---|---|---|---|---|---|---|---|---|---|---|---|---|---|---|---|---|---|---|---|---|---|---|---|---|---|---|---|---|---|---|---|---|---|---|---|---|---|---|---|
|
of the pulmonary vein, the LAP may be estimated. If there is blunting of the S wave in all pulmonary veins, an elevation of LAP (> 15 mm Hg) is usually present (55,56,57). In chronic MR, the left atrium may dilate to greater than 70 mm (56,57). With persistent elevations of LAP, the patient may develop fixed pulmonary hypertension with RV dysfunction, eventually causing RV dilatation, and lateral enlargement of the tricuspid annulus, leading to tricuspid regurgitation in up to 25% of patients having MV surgery (75). The RAP may be elevated leading to chronic hepatic congestion (diagnosed by a lack of respiratory variation in hepatic vein diameter) and in the present right-to-left shunting patent foramen ovale.
![]() FIGURE 28.39A, B, C, and D. A: The post-CPB TEE exam following MVRep may reveal short duration MR jets with a significant maximum jet area. The color m-mode clarifies the duration of the jet. B: Color flow Doppler is a good screening technique to distinguish the presence of severe or mild MR. Eccentric jets will lead to an underestimation of MV severity due to the wall hugging (Coanda effect). D: Due to the many factors influencing the jet area size (frequency, wall filter, gain, Nyquist limit, PRF, sector depth, and loading conditions), quantitative methods (including PISA ROA, and vena contracta) should be used to distinguish intermediate grades (mild to moderate, moderate, and moderate to severe). However, color flow Doppler is an essential component of the exam in determining mechanism of MR. |
and mortality, but it significantly increases the costs associated with valvular heart surgery. The cannulation and perfusion strategy is directed by the IOE assessment of the proximal ascending and descending aorta. Epiaortic scanning is performed if
![]() FIGURE 28.40A. Of 1,072 patients undergoing successful MV repair between 1985 and 1997, the long-term durability at 10 years was 93%. |
![]() FIGURE 28.40B. Hazard curves for reoperation following successful MV repair. Though reoperations are an infrequent event, the first year is time of highest incidence. |
In 1992, Stewart et al. reported the Cleveland Clinic’s initial experience with 286 patients undergoing MVRep (79). In those patients who received IOE guidance, the surgical diagnosis of mechanism and location was compared with the results of the pre-CPB exam. The IOE exam diagnosed the localized mechanisms most accurately with posterior-leaflet prolapse or flail (93%), anterior-leaflet prolapse or flail (94%), and restricted leaflet motion or rheumatic thickening (91%). It correctly diagnosed papillary muscle elongation or rupture in 75%, ventricular and annular dilatation in 72%, leaflet perforation in 62%, and bileaflet prolapse or flail in 44%. Of the 5% of patients who had more than one mechanism, the IOE was only able to diagnose both mechanisms in 38%, and one of the two mechanisms in 92%. Overall, the accuracy for diagnosing mechanisms was 85% (33). In a similar study, Foster et al. reported the high accuracy of utilizing a more regimented systematic IOE exam, reporting agreement between the TEE and surgical localizations of mechanisms in 96% of native MV segments or scallops (224 of 234 segments or scallops, p < 0.0001) and 88% of perivalvular prosthetic regurgitant segments (p < 0.001) (80). Lambert et al. reported on the ability of a consistent systematic examination to accurately determine the location and mechanism of MV dysfunction (compared with surgical inspection) in 96% compared with 70% in an IOE exam that was not systematically performed (81). Omran et al. prospectively evaluated 170 consecutive patients undergoing MVRep with a systematic segmental examination of the MVAp. They found that IOE accurately identified abnormal segments in 90 to 97% of patients (82). Segments that were most accurately identified as abnormal were P2 (97%) and least accurately identified were A3 (90%). The accuracy of correctly localizing to AMVL and PMVL was 95% and 100%, respectively (82). In 1999, Caldarera et al. discovered that IOE provided accurate anatomic measurements of the anteroposterior diameter of the mitral annulus compared with surgical inspection (83). A measured diameter > 35 mm in the ME 4 Chr imaging plane was indicative of annular dilatation at the time of surgery requiring annuloplasty. In 1999, Enriquez-Sarano et al. compared the ability of IOE to accurately diagnose the etiology and mechanism of MV dysfunction in patients undergoing MVRep surgery with TTE (29). They found that IOE provided a superior ability to diagnose the correct etiology (99% vs. 95%) and mechanism of dysfunction (99% vs. 94%). The IOE exam was also more accurate in diagnosing MV prolapse (99% vs. 95%) and flail segments (99% vs. 83%, p < 0.001). The IOE exam was able to predict, on the basis of accurately diagnosing etiology and mechanism, those patients who were most likely to have a successful MVRep (p < 0.001) and a higher 5-year survival (p < .001) (29). Similar studies have consistently demonstrated the diagnostic capabilities of the IOE exam to accurately provide the surgical team with the information that will enable them to correlate their surgical findings in determining the optimal surgical strategy for repair or replacement of the valve.
Based on this finding the decision was made not to surgically inspect the valve in 95 of these patients. In patients with ischemic MR undergoing myocardial revascularization, Cohn et al. reported on patients undergoing myocardial revascularization with moderate preoperative MR; 90% of these patients were downgraded to 0-2+ by the IOE exam (19). Seven of the patients went from moderate MR to no MR intraoperatively and did not receive a MVRep or MVR. Postoperatively, 3 out of 7 patients returned to their original 3+ MR, and 4 returned to 2+ MR on their postoperative TTE (19).
p = 0.012) prior to pre-CPB compared to those who did not develop LVOT obstruction with SAM (93). These findings were consistent with studies demonstrating that SAM with LVOT obstruction following MVRep surgery is associated with anterior malposition of the point of coaptation. This study lends further support to the strategy of the sliding annuloplasty to prevent post-MVRep LVOTO (90,91,92). From these studies the predictive indicators of post-MVRep SAM with LVOTO would be a AMVL: PMVL ratio less than 1.0, PMVL height > 1.5 cm, and a small systolic LVOT diameter (C-septal distance < 2.6 cm)
would indicate a higher risk MVRep patient population for developing post-CPB SAM and LVOT obstruction and the potential need for a posterior MV complete or modified sliding annuloplasty.
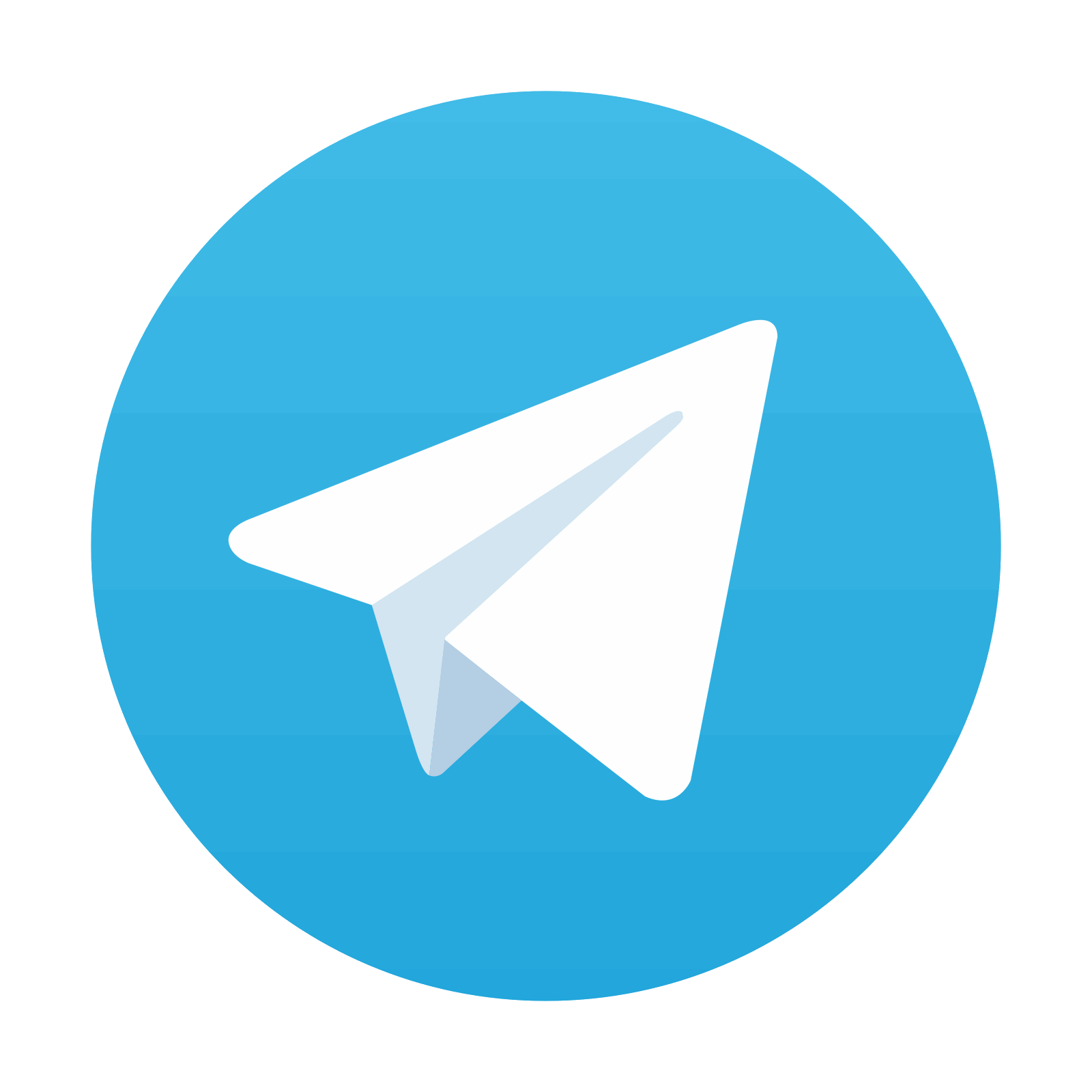
Stay updated, free articles. Join our Telegram channel

Full access? Get Clinical Tree
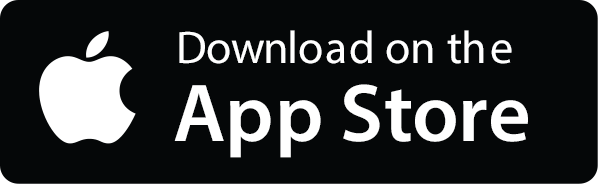
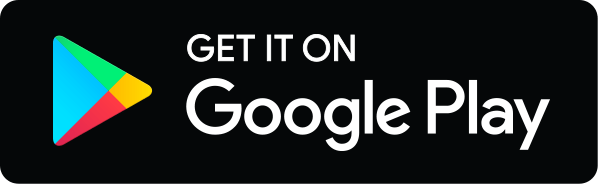
