The introduction of routine absolute myocardial blood flow assessments for cardiac PET adds unique information that is difficult, if not impossible, to acquire using other noninvasive modalities. Specifically, the assessment of absolute myocardial blood flow with cardiac PET improves the determination of normalcy,1,2 detection of multivessel disease,3 detection of balanced flow reduction,4 detection of microvascular disease,5 and risk stratification.6–8 These benefits can only be obtained if the quantitative values are accurate.9
The assessment of myocardial blood flow with PET uses the measurement of the transport of tracer from the blood pool into the myocardium10–13 to calculate the blood flow in the coronary arteries. First, this requires an accurate dynamic scan across a wide range of count rates. In many systems, the count rate can be 10 times the activity of a conventional perfusion scan, with the entire, undecayed bolus in the field of view at once. In the case of 82Rb, that activity will decay to near background levels during the course of the study. This complex, quantitative study must be accomplished without adding to significantly the radiation dose or compromising the quality of the clinical study.14,15
The choice of the radiotracer used to measure blood flow, in principle, should not have an impact on the blood flow. However, protocols and flow models for measuring blood flow are typically specific to the radiotracer used and have challenges particular to that agent.10–13 In addition, quality control and data processing steps depend heavily on the choice of radiotracer. Clinicians must carefully assess data quality for patient motion,16 accuracy of the placement of the blood pool region of interest (ROI),17,18 and the accuracy of myocardial boundaries. Quality control in the assessment of myocardial blood flow carries a greater importance than in visual analysis of perfusion data because quantitative flow can correctly contradict visual assessment.
Ultimately, the assessment of myocardial blood flow with cardiac PET can greatly increase the diagnostic information available to the cardiologist; however, this information may complement, complicate, or even contradict the visual assessment of the study. Because of this, the utility of absolute blood flow assessment requires an understanding of the entire acquisition, processing, and quality control procedures by both the technologist and the interpreting nuclear cardiologist. This chapter describes the scientific foundation of myocardial blood flow assessment, methods for optimizing myocardial blood flow measurements, and strategies for assessing the quality of myocardial blood flow PET studies.
The assessment of myocardial blood flow with PET is made possible because of two characteristics of PET imaging: (1) all PET studies are attenuation and scatter corrected, and (2) PET studies are always acquiring a full 360 degrees of data. This constant collection of tomographic data allows for the creation of high-quality dynamic data at very short time intervals. It is this dynamic measurement of the kinetics of the uptake and washout of the tracer from the myocardium that allow for the quantitative assessment of the blood flow. The dynamic acquisition used for the assessment of myocardial blood flow uses a series of short tomographic images over the duration of the study to measure the transport of the tracer out of the blood pool into the myocardium. There are two primary means of acquiring blood flow data. The most common technique for acquiring the dynamic data is using a list-mode acquisition, illustrated in Figure 4-1. These acquisitions record each true coincidence event in a file so that multiple virtual “acquisitions” can be performed post-acquisition over the entire acquisition. This type of acquisition allows for rebinning of the gated, perfusion, and dynamic datasets from the single list-mode acquisition (Figure 4-1).
A second approach uses a short dynamic framed acquisition at the beginning of the study. This technique then is followed by a longer perfusion and gated study (Figure 4-2). Both techniques have been validated and can produce accurate results; however, different flow models must be employed depending on the type of acquisition used.19,20 This method has the advantage of being less susceptible to the effects of tracer washout and patient motion, and it can be implemented on list-mode and non-list-mode PET systems.
The dynamic PET study is a set of short, continuous tomographic acquisitions starting prior to the start of the infusion (or injection) of the radiotracer and concluding in a final frame representing the cumulative uptake and washout of tracer. This dynamic study can be produced in real time as a series of sinograms or from a virtual rebinning of list-mode data. The dynamic scan typically uses shorter acquisition times near the peak of the blood pool bolus activity and then longer acquisition times during the uptake phase of the study.9–11 For accurate calculation of the myocardial blood flow, it is necessary to measure the entire uptake process of the tracer into the myocardium; from prior to the start of the infusion to the final uptake frame. Technologists should allow for sufficient time between the start of the acquisition and start of the injection of the radiotracer to establish that no part of the blood pool activity is missed.
During the dynamic acquisition, technologists should ensure that the patient avoids motion. Patient motion, either position movement or respiratory, can lead to inaccurate flow values that can be difficult to detect.16,21 For some flow models, it is recommended that patient motion be corrected prior to evaluating myocardial blood flow. This motion correction differs from misregistration correction and artifact-producing patient motion. Motion correction of the dynamic study must be performed to ensure that the blood pool region of interest tracks is correctly registered over the myocardium and not the blood pool or outside the cardiac region.
The processing of myocardial blood flow PET studies is similar to the processing of conventional myocardial perfusion PET studies. It requires that the sinogram data be corrected for misregistration of transmission and emission datasets, and using an appropriate iterative algorithm. It is important that in the processing myocardial blood flow PET studies to use image-filtering parameters as are required by the specific quantitative software. Altering these values can change the quantitative accuracy of blood flow estimates. Once the dynamic volumes are created by reconstruction software, the myocardial blood flow study must be reoriented onto conventional cardiac oblique angles and the myocardial boundary and blood pool region of interest (ROI) identified.17
Last, it is essential that sufficient counts are obtained throughout the dynamic series. Technologists and clinicians should inspect entire dynamic series to ensure the myocardium and blood pool images are of sufficient quality to obtain accurate measurements.
Calculating myocardial blood flow from measurements of tracer uptake and blood pool concentration requires a model for transport of tracer activity into the myocytes. The most common model for tracer transport is a multicompartment model. Each compartment of the model represents a location the tracer can be in during the acquisition. In the simplest model, the tracer begins in the blood (compartment one) and then is transported into the cell (compartment two). More complicated models include parameters for washout from the cell, compartments for the interstitial layer, metabolism of the tracer, and different subcomponents within the cell.
The choice of a compartmental model for calculating myocardial blood flow is based on an understanding of the underlying kinetics of the tracer and the parameters of the acquisition. In addition, consideration should be given common imaging artifacts, such as image noise, patient motion, and noncardiac uptake. These practical considerations become essential as the complexity of the model increases.
The general form of a three compartment model is12:
where Cm is the myocardial uptake, K1 is the uptake into the first tissue compartment, k2 is the washout from the first compartment, k3 is the uptake into the second tissue compartment, t is the timepoint the uptake is measured and Cm (t) is the arterial concentration at timepoint t. This model is also often referred to as a two-tissue compartment model (2TCM) where one compartment represents the blood and the two-tissue compartments represent the cell.
To calculate the myocardial blood flow from the compartmental model, a model for the tracer extraction is needed. The extraction of activity from the blood is often modeled using the Renkin-Crone formula22,23:
where MBF is the myocardial blood flow and a and b are fit parameters. A list of common a and b parameters is given in Table 4-1.11,23–28
A | B | k2 (Rest/Stress) | k3 (Rest/Stress) | |
---|---|---|---|---|
Rb-82 (10) | 0.77 | 0.63 | 0.12/0.21 | NA |
N-13 ammonia (10) | 0.607 | 1.25 | 0.23/0.89 | 0.14/0.13 |
C-11 acetate (23) | 0.64 | 1.20 | 3.0 | 1.0 |
F-18 flurpiridaz (20,27) | 0.06 | 0.0 | NA | NA |
O-15 water (25) | 0 | 0 | Freely diffusible | NA |
Tc-99m sestamibi (19) | 0.86 | 0.267 | NA | NA |
Early studies of the 2TCM for 13N-ammonia demonstrated a high degree of accuracy compared to microspheres. Choi et al in 1999 examined the quantitative myocardial blood flow of 13N-ammonia using four different quantitative models.24 In that study, the 2TCM was demonstrated to have low dispersion (0.15) and correlated well with microspheres results. The authors also noted the importance of the correction of washed out metabolite. In a separate study comparing blood flow models using 13N-ammonia, it was demonstrated that the single-tissue compartment model did not accurately represent the myocardial blood flow as well as the two-tissue compartment model.
82Rb is a potassium analog and thus is not metabolized by the myocytes. Because of this 82Rb can be modeled using a single-tissue compartment model (1TCM). Lortie et al in 2007 presented a simplified single-tissue compartment model for calculating the myocardial blood flow using 82Rb.11 This model uses a nonlinear fit to a series of dynamic measurements of uptake arterial blood pool to estimate the K1 (uptake), k2 (washout), and partial volume corrections (f).
The 1TCM can be derived by setting k3 = 0:10
1TCM has been shown to accurately represent the estimate of myocardial blood flow for 82Rb myocardial blood flow11 and is highly repeatable.29
This model can be further simplified by setting k2 = 0. This model is referred to as the net retention model.9 Comparison of the net retention model with the full 1TCM demonstrates a high degree of correlation between blood flow calculations in the first 2 minutes of a study. Following the first 2 minutes, the influence of the washout reduces the accuracy of this model. One of the advantages of this simple model is that it can be performed on non-list-mode, frame-mode PET systems. Another advantage of this technique is that the myocardial uptake is calculated from a single late frame, making it less susceptible to motion artifacts. One of the limitations of this technique is that the partial volume correction cannot be determined from the data and must be supplied as a parameter.
A major concern of clinicians is the robustness and interchangeability of myocardial blood flow measurements. In a large study comparing myocardial blood flow calculation techniques in 82Rb, net retention, 1TCM and 2TCM models produced similar results for the mean rest and stress values for flow; however, there were differences observed on a patient-by-patient basis depending on the kinetic model that was used19 (Table 4-2). These results confirm the overall robustness of these methods and demonstrates their interchangeability.30 In addition, software packages using the same compartmental modeling algorithm produced interchangeable results. This further validates the consistency of these software packages, despite varying implementation strategies. The key limitation of this study is that no gold standard was available and, therefore, the overall accuracy of particular techniques cannot be assessed relative to true myocardial blood flow.
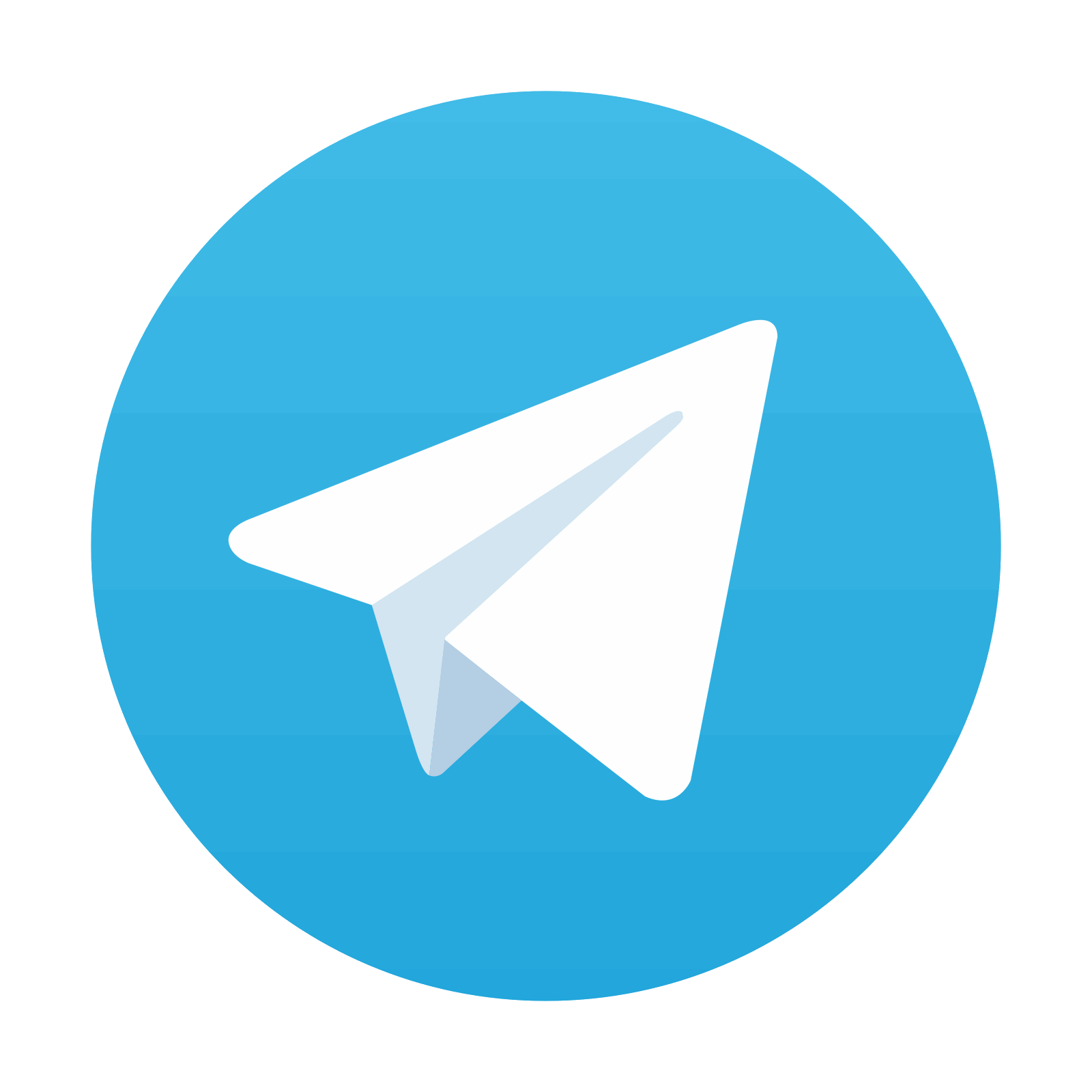
Stay updated, free articles. Join our Telegram channel

Full access? Get Clinical Tree
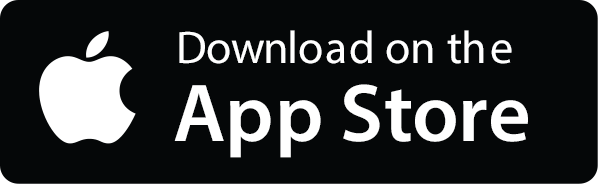
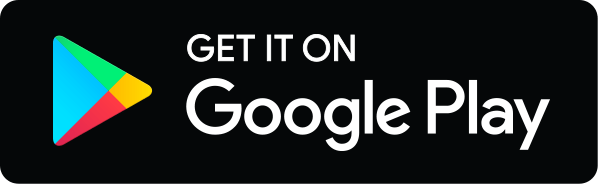