Fig. 13.1
Left panel: Representative plateau pattern of log-log plot of mPAP vs. VO2 in eiPAH. Right: Representative “take-off” pattern of log-log plot of mPAP vs. VO2 in eiPAH
EiPAH has been described as an intermediate clinical entity by two other groups. Condliffe et al. [43] noted that 19 % of 42 systemic scleroderma (SSc- eiPAH) patients developed resting PAH when a repeat right-heart catheterization was performed. Saggar et al. [44] also noted that 3 of the 24 SSc-eiPAH patients developed resting PAH when followed serially over time. This finding was reported as part of an open label clinical trial of ambrisentan. In the same study using invasive exercise testing they also demonstrated the positive therapeutic effect of Ambrisentan on exercise hemodynamics in these subjects. In summary, iCPET can be used to diagnose disease at an earlier, milder phase. ICPET can also be used for the accurate assessment of treatment effect on pulmonary artery hemodynamics.
In Chronic thromboembolic pulmonary hypertension (CTEPH) patients, invasive exercise hemodynamics has been utilized to define the cause of persistent exertional dyspnea in post pulmonary endarterectomy patients. These subjects were found to have an abnormal pulmonary vascular response to exercise based on an abnormal PVR and pulmonary arterial compliance when the hemodynamics in spite of normal in resting hemodynamics. The increase in PVR was mild, relative to the alteration in compliance, and exercise arterial compliance was the only independent predictor of decreased exercise capacity in this group of subjects [45]. In addition to what has already been discussed, iCPET can also be used to include or exclude other causes of exercise intolerance like preload-dependent limitations to stroke volume and cardiac output, oxidative myopathies, and left to right shunting.
The limitations of exercise hemodynamic testing are significant because of its invasive nature that limits its use for serial measurements. Although rare, other potential complications include infection, vascular thrombosis, and pain at the site of catheter placement. ICPET can be costly, labor intensive, and requires technical expertise not only for accurate performance but also for recording and interpretation of the results. Consistent invasive measurements during exercise are challenging to perform and must be interpreted carefully because of the potential for large intra-thoracic pressure swings associated with increased minute ventilation during exercise. Boerrigter, et.al studied the worst possible scenario for this problem when they studied iCPET in a COPD population with esophageal balloons in place. They suggested that central hemodynamics measured using conventional end‐expiration method might lead to an overestimation of intravascular pressures during exercise. To obtain exercise hemodynamic data more reliably by averaging pressures over the respiratory cycle or using the RAP waveform to correct for intra-thoracic pressure (Fig. 13.2a, b) [4].
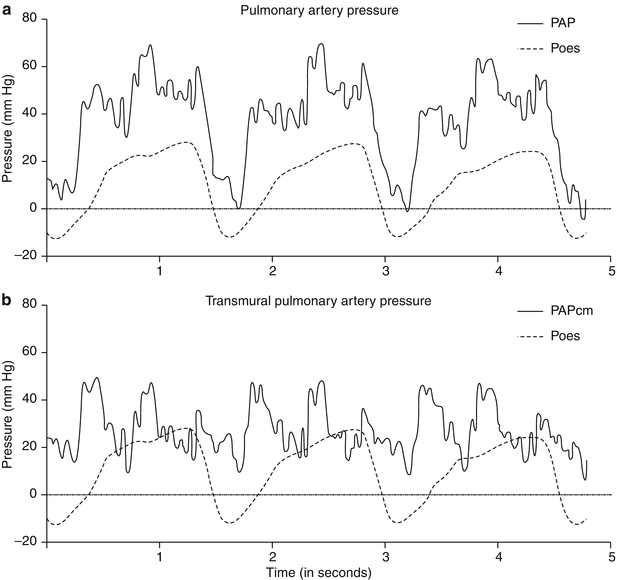
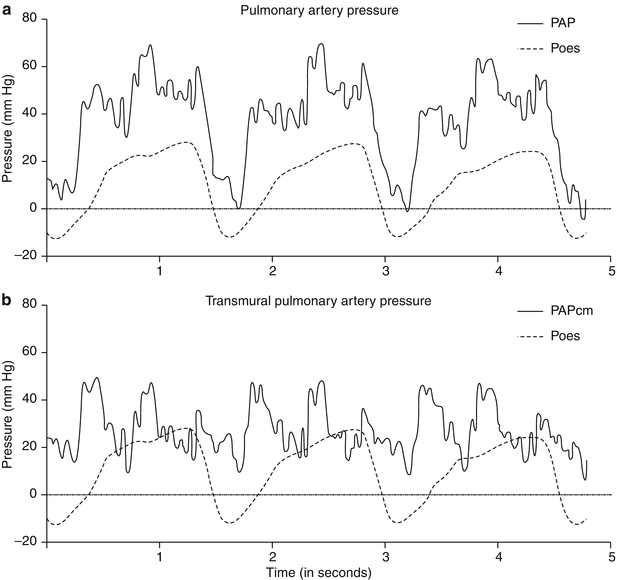
Fig. 13.2
(a). Example of pulmonary artery pressure before (PAP) and after (PAPtm) continuous correction for esophageal pressure (Peso) at maximal exercise in a patient with severe COPD (FEV1: 30 % of predicted). (b) Simultaneous measurement of RAP and esophageal pressure (Peso) at maximal exercise in the same patient as shown in Fig. 2.1. RAPnadir is the lowest point in RAP during expiration, which represents RAP during relaxation. Note that RAP falls towards Peso during relaxation. RAPswing was determined as the difference between inspiratory RAP and expiratory RAP
Imaging of the Right Heart System
Functional status and mortality in patients with PAH is related primarily to RV structure and function. Direct assessment of RV function using noninvasive techniques may provide a more informative way of determining response to therapy and monitoring disease progression in PH. With constant advances in the field of imaging, we now has access to a number of non invasive imaging tools to more clearly define the interaction between the RV and pulmonary circulation. Magnetic resonance imaging (MRI) and Computed Tomographic (CT) scanning are becoming increasingly important to study the RV-PA coupling and provide detailed quantitative data with extreme quality. Prognostic markers have been identified with independent predictive value for identification of treatment failure.
Computed Tomographic Imaging
Over the past decade, cardiac CT has evolved rapidly from the introduction of 4-detector row systems to those with 64 detectors, dual-source CT and, more recently, 320-detector systems. This has been accompanied by improvements in spatial and temporal resolution, extended longitudinal coverage and decreased scan times and radiation exposure. Current generation ECG gated multidetector CT (MDCT) scanners have a spatial resolution that ranges from 0.3 to 0.5 mm, which permits accurate and consistent assessment of the RV and PA. In addition use of the contrast agents, gives us additional information about ventricular volumes, ejection fraction, and vascular structures.
Studies have evaluated the specificity and sensitivity of CT defined dilatation of the main pulmonary artery as a marker for pulmonary hypertension. A value ≥29 mm had a sensitivity and specificity for the detection of PH of 77.4 % and 89.6 % respectively. The ratio between pulmonary artery and abdominal aorta when greater than 1, was shown to be 86.8 % sensitive and 79.2 % specific for a diagnosis of PH. This ratio correlated with RHC derived mean PA pressures and in combination with ECHO derived RV systolic pressures. The correlation was (R2 = 0.45, p < 0.001) alone, and (R2 = 0.55, p < 0.001) in combination, giving a 96 % specificity and 59 % sensitivity for PH diagnosis [46–48].
Kuriyama et al. have reported the use of cross-sectional areas of the main and inter-lobar pulmonary arteries for estimating the pulmonary artery pressures. In this study the upper limit of normal diameter for the main pulmonary artery was found to be 28.6 mm. In the PH patient group, the diameters were correlated with data from cardiac catheterization and a value greater than 28.6 mm readily predicted the presence of pulmonary hypertension. The calculated cross-sectional areas of the main and inter-lobar pulmonary arteries were also found to give the best mPAP estimates (r = 0.89, P < 0.001 and r = 0.66, P <0.001) [49].
Groves et al. demonstrated that contrast reflux into the inferior vena cava and hepatic veins on first-pass contrast-enhanced CT was a strong marker for tricuspid regurgitation (TR), having 90.4 % sensitivity and 100 % specificity when compared to echocardiography. They further demonstrated excellent correlation between semi-quantitative grading of TR with systolic PA pressure measured by RHC (r = 0.69, p < 0.001). Subsequently, others have shown that high-grade contrast reflux was associated with a diagnosis of PH (OR 5.41, 2.95–9.94 95 % CI, p < 0.001) in a large retrospective cohort [50, 51].
When CT-measured PA volumetric analyses are adjusted with body surface area, they correlate highly with mean PA pressure (r =0.89, p < 0.05) [52] A study in patients awaiting lung transplantation demonstrated that mPAP correlated strongly with the combination of cross-sectional areas of the main and left main PA indexed to body surface area (r = 0.81, p = 0.0001) [53].
Simon et al. have also shown the utility of ECG gated MDCT to detect the regional changes in RV structure and function, which may be early markers of patients at risk for developing RV failure. The increased pulmonary vascular resistance exposes the right side of the heart to chronic pressure and volume overload. The morphology of the heart compensates in the form of septal bowing into LV. When the heart contracts, the LV faces the consequences of pressure overload while during the relaxation phase, the volume changes interfere with its normal functioning. These manifest as RV hypertrophy and RV strain. This study emphasized that patients with different pulmonary and RV filling pressures display significantly different phenotypes. Even in the presence of normal RV filling pressures, RV infundibular changes might be useful to characterize regional RV remodeling and function in PH and direct therapeutic decision making early in the disease process [54].
In group 4 PH patients, pulmonary thromboendarterectomy (PTE) is the recommended treatment but the prediction of post surgical outcome remains challenging. In CTEPH patients Heinrich et al. have reported how pre operative CT scan findings can help predict hemodynamic improvement after PTE. They also concluded that absence of central thrombi is a significant risk factor for inadequate hemodynamic improvement. In a more recent study Scholzel et al. found pre-operative PA diameter indexed for body surface area as the only independent predictor for hemodynamic improvement after PTE in CTEPH [55, 56].
Although CT scan has been shown to give us important data about pulmonary vessels and morphological changes in heart structure limitations of radiation exposure, use of contrast agents, and little experience in pulmonary vascular diseases, makes its use limited.
Cardiac Magnetic Resonance Imaging
Noninvasive cMRI provides a comprehensive picture of RV structure and function with excellent resolution. It reports on markers of RV structure and function including right ventricular end-diastolic volume index (RVEDVI), left ventricular end diastolic volume index (LVEDVI), right ventricular ejection fraction (RVEF), and relative area change of the pulmonary trunk. The use of cMR in PAH is increasingly attractive as cMR is considered the gold standard for assessment of RV volume, mass, and function. In a recent pan-European study investigating the relationship between RV structure and function, and survival, a large RV end-diastolic volume, low left ventricular end-diastolic volume, and a low SV at baseline were associated with a poor prognosis. Progressive dilatation of the RV, a further decrease of LV diastolic volume, and a further decrease in SV at follow-up predicted treatment failure and a poor long-term outcome [57] MRI is ideally suited to longitudinal follow-up of patients with PAH due to its non-invasive nature, high reproducibility and has the advantage over other biomarkers in PAH due to its high sensitivity to detect changes in morphological, functional and flow related parameters.
CMRI provides details about the morphological changes in the anatomy of right ventricle, septum and related structures, which correlate with invasive pulmonary hemodynamic parameters. Saba et al. showed that Ventricular Mass Index (VMI) (ratio of right ventricular mass over left ventricular mass) had a strong correlation with mean pulmonary artery pressures (r = 0.81) Also the sensitivity and specificity for detecting PH were 84 and 71 % respectively for the VMI when compared with conventional commonly practiced technique of echocardiography [58]. Similarly septal curvature metrics have been used to judge the RV afterload and to assess the acute vaso-reactivity of pulmonary vasculature during vasodilator testing.
The normally cross sectional circular shape of the LV is the result of the positive pressure gradient between the LV and RV throughout the cardiac cycle. However, in PH, the LV–RV pressure gradient is less than that observed in normal population or can even be negative causing the septum to become either flattened or bow into the LV. Panda et al. have shown this septal curvature using the real time cMRI has a very strong correlation between the PAP and PVRi at baseline and during vasodilator challenge. Also others have shown the presence of a strong correlation by comparing the septal curvature parameters with the hemodynamic parameters of PASP, RVSP measured on RHC [59–61].
With the progression of the disease, the changes in the RV functioning and structure lead to the changes of LV contour. This LV deformation can be quantified using MRI, which helps to calculate echocardiographic indices such as the LV systolic and diastolic eccentricity indices (sEI, dEI). These indices correlate with mPAP and can identify the prognosis of PAH patients. Elevated RV pressures cause the inter-ventricular septum to bow to the left in patients with PH, this leftward motion of the inter ventricular septum causes the deformation of the LV, “D shape”, as a result of the pressure differential between LV and RV chambers. Several investigators have quantified the paradoxical inter-ventricular septal position by measuring the curvature of the septum and have shown strong correlations with the severity of PH [61–63].
Cardiac MR can also be used to track reverse remodeling. In patients with non operable CTEPH, treated with balloon pulmonary angioplasty there was significant improvement in RV dilatation (RVEDVI and RVESVI), RV systolic function (RVEF), RV hypertrophy (RVMI and VMI), and inter ventricular septal bowing assessed by cMR. There were strong correlations between observed changes in RV remodeling, measured with cMR, and changes in invasive hemodynamic parameters. cMR has been used to demonstrate RV reverse remodeling after successful pulmonary thromboendartectomy in CTEPH patients in both early and later phases of recovery [64, 65].
Also MR angiography of the pulmonary arteries and a time resolved contrast enhanced lung perfusion scan can help in identification of CTEPH. The high signal to noise from blood flow provides good contrast within the vessel lumen and thus serves as a non-contrast enhanced angiogram, which can be useful in the delineation of adherent material in the central pulmonary arteries in CTEPH [66]. Contrast enhanced MR angiograms can provide an overview of vessel pruning in PH and the delineation of thromboembolic material in CTEPH.
While cardiac MR is considered the gold standard for measurement of cardiac function, and it offers very high spatial resolution, clinicians have been slow to utilize cardiac MRI for a number or reasons. Data on the use of cMR as an outcome measure is limited. While certain subjects cannot tolerate the claustrophobia they feel inside the magnet, some patients are too large to fit in the MRI chambers. We do not generally image patients who have implanted defibrillators or pacemakers because of heating of the tip of leads. Compared with other non-invasive approaches, cMR is more time consuming. Cardiac MR techniques are also dependent on image acquisition during repeated breath hold maneuvers of 5–10 s. If patients are unable to perform this maneuver, an important consideration in PH patients, image quality can be significantly degraded. Cardiac volumetric parameters vary with age, gender and body surface area. Hence there is also a need to adjust these measurements accordingly as they may be critical to recognize the clinical abnormalities in individual patient [67–69].
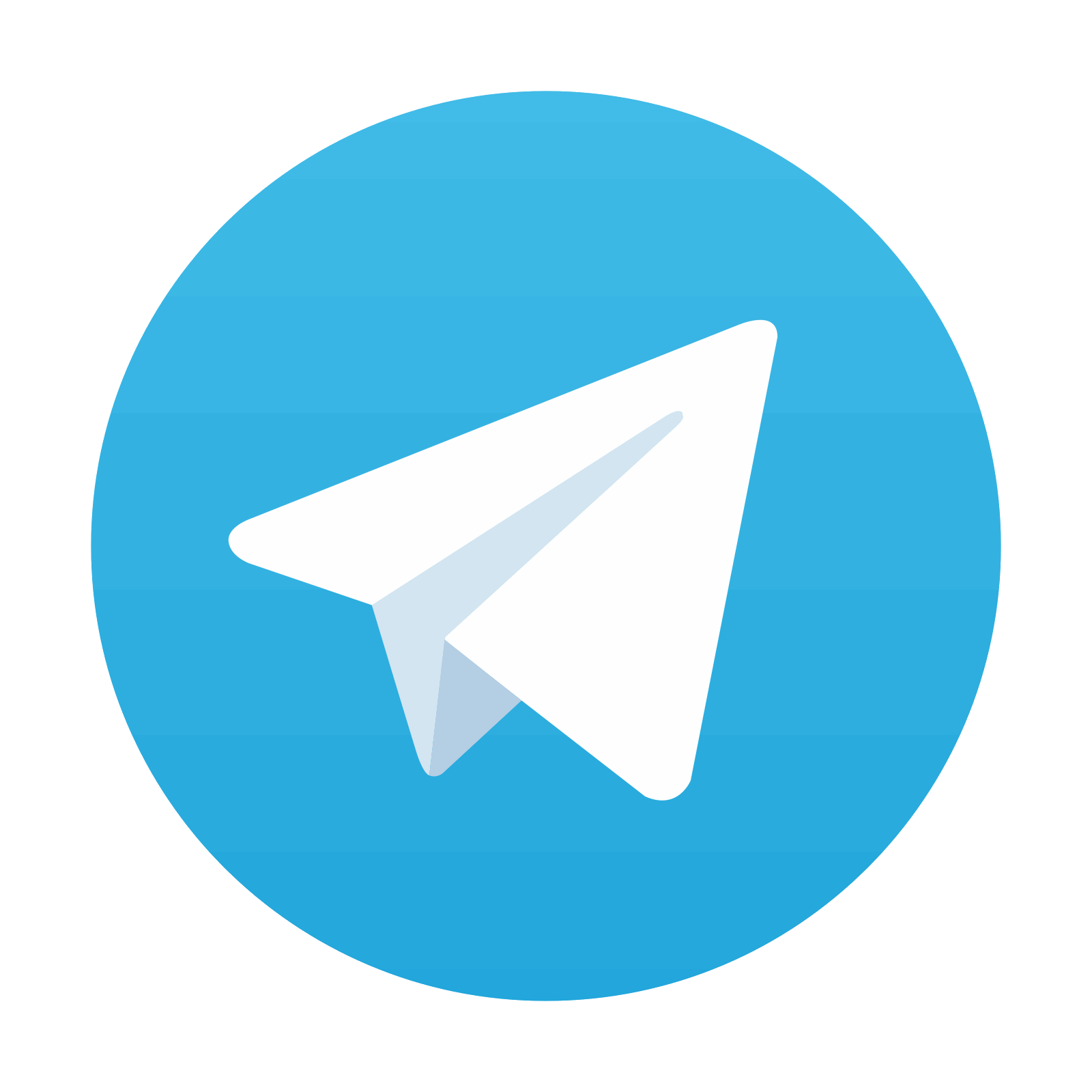
Stay updated, free articles. Join our Telegram channel

Full access? Get Clinical Tree
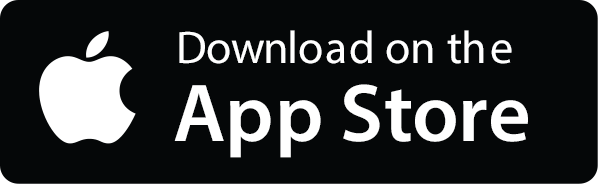
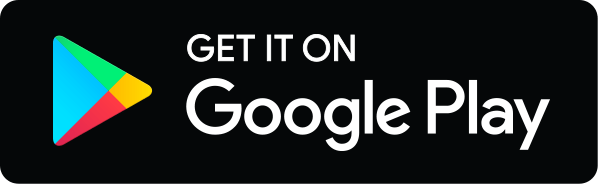