There has been dramatic progress in the field of vascular anomalies in recent years, including improved understanding of the classification of these lesions, their natural history, and optimal management strategies. This section discusses only vascular anomalies that have the potential to pose cardiovascular ramifications, either due to abundant arteriovenous shunting presenting with congestive heart failure (CHF) or pulmonary hypertension or due to right-to-left shunting (at the intracardiac or intrapulmonary level) presenting with cyanosis. Because of the highly varied and imprecise terminologies used in the past to describe a host of vascular anomalies, older publications are difficult to rely on for accurate diagnoses and are predominantly useful to provide historical context regarding the early descriptions of such conditions. One of the earliest reports of “congenital hemangioendothelioma” (a term that should no longer be used because it does not convey with clarity the precise nature of the lesion according to modern standards) with cardiovascular symptoms in infancy was by Kunstadter in 1933, in which he describes a 5-month-old infant with dyspnea and cyanosis. Levick and Rubie described a similar case of “hemangioendothelioma” simulating congenital heart disease in an infant with dyspnea and cyanosis; pathologic findings showed multiple nodules measuring approximately 1 cm distributed throughout both lobes of the liver; the heart was considerably enlarged with biventricular hypertrophy, and an interatrial communication was seen together with a tiny arterial duct. Right-to-left interatrial shunting is presumed to have been the cause of cyanosis. With this historical context in mind, we will discuss specific vascular anomalies according to currently accepted terminology.
Hepatic Hemangioma
Clinical Features, Investigations, and Management
Hepatic hemangiomas are technically not arteriovenous malformations (AVMs), but rather neoplasms representing benign endothelial tumors. Hepatic hemangiomas can be broadly classified into two varieties: congenital and infantile. Both types of hemangiomas can exist in extrahepatic locations, but the cardiovascular ramifications exist only in cases of hepatic hemangioma; hence our discussion will be limited to hemangiomas presenting in the liver. Patients with hepatic hemangiomas commonly have associated skin hemangiomas, frequently multiple, and their presence may be the initial presenting feature and should prompt a search for liver hemangioma. Older literature often used the term hemangioendothelioma , but this term was imprecise and could represent different entities, including congenital or infantile hemangiomas and even malignant angiosarcomas; hence the term hemangioendothelioma is currently discouraged.
Congenital hemangioma is a benign neoplasm of endothelial origin that begins proliferating in utero and is fully formed at, or just before, birth and then follows one of three patterns of natural evolution depending on the subtype: rapidly involuting (until 15 to 24 months of age), partially involuting, or noninvoluting. Affected infants may present with liver manifestations (variceal bleeding, hepatomegaly, splenomegaly), hematologic manifestations (anemia and thrombocytopenia), and high-output CHF due to massive arteriovenous shunting. In addition, cyanosis may occur due to right-to-left shunting across intracardiac communications such as an atrial septal defect or patent arterial duct. Because congenital hemangiomas have reached their peak size at birth, these various manifestations (e.g., CHF) are usually present immediately after birth if the tumors are large enough to cause these sequelae.
The diagnosis of congenital hepatic hemangioma is usually made using noninvasive imaging. The study of choice is two-dimensional ultrasound with Doppler. The ultrasound appearance can be variable, ranging from a hypoechoic to hyperechoic mass, and larger hemangiomas can have a more complex appearance that can include calcifications, thrombosis, and fibrosis. If the diagnosis is unclear, a liver magnetic resonance imaging (MRI) study with contrast and dynamic acquisition pattern can be performed and typically reveals a hypointense lesion on T1-weighted and hyperintense lesion on T2-weighted images. Congenital hemangiomas are most commonly single tumors. The differential diagnosis of a solitary liver mass present at birth or shortly thereafter includes hepatoblastoma, metastatic neuroblastoma, or hamartoma. However, typically, hepatoblastoma would lack the rim enhancement seen in hemangiomas and would be associated with elevated α-fetoprotein levels. Although congenital hemangioma can usually be diagnosed based solely on noninvasive imaging, a biopsy may be occasionally required; it is therefore important to note that congenital hemangioma does not express glucose transporter-1 or lymphatic markers.
Congenital hemangioma can follow one of three natural patterns of evolution: rapidly involuting congenital hemangioma will typically involute within the first 24 months of life, but most regress by 3 months of age, whereas noninvoluting congenital hemangioma will generally not persist, and a third intermediate form is considered to be partially involuting.
Infantile hemangiomas are the most common of all childhood tumors and represent an entity that is distinct from congenital hemangiomas. Infantile hemangiomas continue to proliferate after birth and may reach peak size by 6 to 12 months of age, whether these are cutaneous, hepatic, or in other locations. Although infantile hemangiomas can present with very similar hematologic, hepatic, and cardiovascular manifestations as congenital hemangiomas, an important distinction is that in infantile hemangiomas these features will not be present at birth but may develop during infancy if the tumor mass reaches a sufficient size. Another distinguishing feature is that infantile hemangiomas may express high levels of type 3 iodothyronine deiodinase, leading to inactivation of circulating thyroid hormone and clinical hypothyroidism. It is important to note that this form of infantile hypothyroidism would not be detected by newborn screening because it is expected to develop only after several months of tumor growth.
The diagnosis of infantile liver hemangiomas is usually also made based on noninvasive imaging, usually ultrasound with Doppler, although liver MRI also plays an important role. Unlike congenital hepatic hemangiomas, which are most commonly solitary lesions, infantile hemangiomas are more often multifocal or diffuse. Although biopsy is not typically required to make the diagnosis, infantile hemangiomas characteristically express glucose transporter-1, an important and very specific distinguishing feature. The clinical course of infantile hemangioma is one of universal growth of the tumor up to 12 months of age, followed by gradual regression until approximately 9 years or age.
Infantile liver hemangiomas and congenital liver hemangiomas of involuting types may be observed without treatment in asymptomatic individuals. Although asymptomatic infants may be observed, the prognosis is poor in untreated infants presenting with heart failure, with an estimated 80% to 90% mortality. For patients with significant clinical manifestations, such as CHF and hypothyroidism, propranolol 1 to 3 mg/kg per day has become the mainstay of therapy and has been reported to be effective for hepatic infantile hemangiomas. There is no evidence that any pharmacotherapy accelerates the regression of rapidly involuting congenital hemangioma lesions. In the past, corticosteroids and interferon-α were recommended, but their use has been supplanted by propranolol due to its greater efficacy and lower side-effect profile. Transcatheter embolization (TCE) may be occasionally required to control severe symptoms until propranolol or time result in sufficient regression. Anatomically, these liver neoplasms may possess hepatic arterial supply, as well as extrahepatic arterial supply via the superior mesenteric, phrenic, renal, or intercostal arteries. In addition, portal venous supply may also exist and has been correlated with increased likelihood of CHF. A clear understanding of the vascular anatomy is necessary to carry out an effective embolization. Surgical hepatic artery ligation is now rarely used. Similarly, surgical resection is only rarely required, and liver transplantation is reserved for patients with abdominal compartment syndrome for whom there may not be time to allow for spontaneous or pharmacotherapy-assisted regression.
Systemic Arteriovenous Malformations and Arteriovenous Fistulas
Vascular malformations represent congenital abnormalities of vascular development and are therefore not tumors; hence they neither have disproportionate growth potential nor do they involute over time, but rather tend to grow commensurate with the patient’s growth. Vascular malformations include capillary, venous, lymphatic, arteriovenous, and mixed types. Of these, the only lesions that pose significant cardiovascular ramifications are AVMs and arteriovenous fistulas (AVFs), due to their high-flow state. These fast-flow vascular malformations are characterized by rapid flow from a feeding artery to a draining vein without an intervening capillary bed. An important distinguishing feature is that AVMs contain an intervening nidus of dysmorphic vessels, whereas AVFs consist of a feeding artery draining directly into an arterialized draining vein. Examples of congenital AVFs include vein of Galen malformation and arterioportal fistula (APF). AVMs and AVFs can be sporadic or exist as part of an AVM syndrome, such as hereditary hemorrhagic telangiectasia (HHT). Imaging modalities include Doppler ultrasound and MRI; in addition, computerized tomography (CT) angiography may be useful to define the feeding arteries and draining veins. These fast-flow lesions are the only vascular anomalies that require conventional arterial angiography for optimal definition.
Hepatic Arteriovenous Malformations
Hepatic AVMs are much rarer than hepatic hemangiomas and have no potential for spontaneous regression. Congenital hepatic AVMs are typically localized in a single lobe of the liver and present in neonates with hepatomegaly, anemia, CHF, or portal hypertension. Hepatic AVMs are differentiated from hepatic hemangiomas by the usual presence of multiple skin hemangiomas in the latter. In hepatic AVMs the presence of pulsatile venous tracing on Doppler ultrasound is typical. Mortality from untreated hepatic AVMs is approximately 50%. Pharmacologic management of hepatic AVMs is limited to therapies aimed at supporting adequate cardiac output and anticongestive therapy using diuretics. Percutaneous embolization of congenital hepatic AVMs has become the mainstay of therapy. Embolic agents such as alcohol and N-butyl cyanoacrylate should be aimed at the central nidus to maximize effectiveness; coils and vascular plugs cannot reach the nidus and thus occlude only the feeding arteries, which predisposes to rerecruitment and recurrence of the AVM.
Hepatic AVMs associated with a predisposing syndrome such as HHT (also known as Osler-Weber-Rendu syndrome) are usually asymptomatic in children but become more commonly symptomatic in adulthood. HHT will be discussed further in the section on pulmonary AVMs.
Arterioportal Fistula
APF represents an abnormal communication between a systemic artery and a splanchnic vein. APF was first reported by Goodhart in 1889 and Sachs in 1892. Acquired APF are most common; they can occur due to tumor invasion or cirrhosis or as a result of penetrating liver trauma and can also be iatrogenic following liver biopsies and percutaneous transhepatic vascular access. Most acquired APFs have been reported in adults, and treatment by transcatheter arterial embolization is performed to reverse portal hypertension, ascites, and variceal bleeding.
Germane to this textbook are the rarer congenital APFs. These may present with sequelae of portal hypertension and/or CHF and occasionally with cyanosis. Some APFs may have an underlying congenital cause and yet not be present at birth; these include syndromes that predispose to arterial aneurysms (e.g., Ehlers-Danlos syndrome) with subsequent aneurysmal rupture into an adjacent portal vein or syndromes associated with the development of liver AVFs (Osler-Weber-Rendu syndrome, also known as HHT). These cases, despite a congenital predisposition, are better classified as acquired APFs. Truly congenital (i.e., present at birth) APFs are very rare and can be classified according to their location: intrahepatic or extrahepatic. Congenital intrahepatic APF may exist in a single lobe of the liver or in both lobes and may receive exclusive hepatic arterial supply or additional arterial supply from other arteries. In addition, a venous varix may exist at the site of the draining portal vein or umbilical vein. It has been suggested that the occurrence of CHF in congenital APF is linked to the presence of a patent ductus venosus: it is presumed that the ductus venosus provides a low-resistance path for blood flow into the right heart, whereas in the absence of a ductus venosus flow through the APF must necessarily flow subsequently through the hepatic parenchyma which may serve to limit shunting by posing an additional resistance to flow. In neonates and infant presenting with severe heart failure, the presence of an atrial communication or arterial duct may allow right-to-left shunting resulting in cyanosis. Congenital APFs presenting in adulthood with symptoms related to portal hypertension have also been reported.
TCE has been advocated as the treatment of choice given that selective surgical ligation of the feeder artery may be difficult, especially in deep intraparenchymal lesions. However, reports of successful treatment of congenital APF are rare.
Arteriovenous Fistula Involving the Umbilical Vein or Ductus Venosus
Hartung et al. reported on two cases of AVFs presenting in utero with fetal hydrops, one consisting of a tortuous artery arising from the aorta and the other consisted of a branch of the hepatic artery, both entering the umbilical vein directly. Both cases had trisomy 21, and both suffered in utero demise. Postnatal descriptions of similar fistulas are rare but illustrate the ability to successful ligate these vessels to control the shunt. Rarer still are direct communications between and umbilical artery and the umbilical vein. Such a case was described by Berg in a fetus with trisomy 18 and tetralogy of Fallot, and the AVF was associated with the development of a calcified aneurysm of the umbilical vein. A rare case of umbilical AVF detected in an infant was successfully ligated with good long-term outcome.
Vein of Galen Aneurysmal Malformations
Vein of Galen aneurysmal malformations (VGAMs) are rare and fascinating lesions that have long piqued the interest of pediatric neurosurgeons, neurologists, radiologists, and cardiologists. In the severest form or if left untreated, VGAMs can prove to be fatal. The types of malformations that are most commonly referred to as VGAMs are, in fact, a misnomer: In these malformations the arteriovenous connections actually drain into the precursor of the vein of Galen, known as the median prosencephalic vein of Markowski, and this precursor is actually the dilated channel. The vein of Galen is the dilated channel when the arteriovenous connections drain directly into the vein of Galen, which is considered a separate entity. A number of different classification schemes have been used to describe these lesions. VGAMs may consist of a network of vessels or direct arterial feeders into the median prosencephalic vein of Markowski. Associations of VGAMs with RASA1 and ACVRL1 gene mutations have been reported. In addition, a familial association of VGAMs has been described.
The clinical manifestations are variable and depend on the age at presentation, whether in the neonatal period, infancy, childhood, or adulthood (less common). Due to increasing sophistication of prenatal imaging, fetal presentation is being reported more commonly. It is not uncommon for neonates and infants with VGAMs to be misdiagnosed or diagnosed late due to the rarity of this lesion. Neonates generally present with the severest form of the disease, with tachypnea, fatigue, poor feeding, and poor growth. Systemic organ hypoperfusion can manifest if there is significant runoff in the aorta and can lead to signs of intestinal, renal, or hepatic ischemia. In severe cases, cardiorespiratory compromise may ensue if left untreated. Neurologic compromise can manifest as seizures, developmental delay, and headaches in patients who present late. Physical examination reveals a dynamic cardiac precordium and bounding pulses from runoff in the aorta due to the arteriovenous shunting. Signs of pulmonary hypertension with a loud pulmonary component of the second heart sound may be present. Macrocephaly from hydrocephalus can be present. A characteristic cranial bruit may be heard on auscultation.
Chest radiographs reveal cardiomegaly ( Fig. 50.1 ) in symptomatic patients, particularly neonates. Cardiac chamber enlargement or hypertrophy can be seen on electrocardiograms. Transthoracic echocardiography confirms cardiac chamber enlargement, which is usually of the right side of the heart ( Fig. 50.2 ) but may progress to enlargement of all chambers and, if left untreated, eventually impacts ventricular function. Signs of pulmonary hypertension may be apparent by transthoracic echocardiography. Doppler ultrasound is a useful screening tool in neonates and can detect the dilated venous sac ( Fig. 50.3 ) and hydrocephalus. However, CT and MR with angiography ( Fig. 50.4 ) are needed to evaluate for cerebral atrophy, abnormalities in venous drainage pathways (e.g., thromboses), intracranial hemorrhage, and the details of the arteriovenous connections. Conventional angiography via the carotid arteries is generally reserved for interventional purposes. Fetal cardiac diagnostic features of VGAMs by ultrasound consist of an enlarged cardiothoracic ratio, reversal of diastolic flow across the aortic isthmus, and significant tricuspid regurgitation velocity and severity; significant tricuspid regurgitation in the fetus with VGAM is considered a poor prognostic indicator.




The natural history of VGAMs is clouded by the fact that many historical reports include malformations that result in dilation of both the median prosencephalic vein of Markowski and the vein of Galen. Due to the rarity of the lesion, meta-analyses have proven helpful in understanding outcomes in patients with VGAMs. In one meta-analysis, nearly 77% of patients who did not undergo treatment were found to have died, whereas in another meta-analysis, the risk of preoperative (including endovascular techniques) sudden death was found to be 6%. Spontaneous thrombosis of VGAMs has also been reported and is not uncommon. Medical treatment includes supportive therapy, antiepileptic therapy for seizures, management of hydrocephalus/raised intracranial pressure, management of heart failure symptoms, and respiratory support with mechanical ventilation if needed. Ultimately, direct treatment of the lesion is required. Surgery was historically performed but was associated with high mortality. However, surgery is needed in some cases to manage intracranial hemorrhages and hydrocephalus.
The treatment of choice in the current era is endovascular embolization, which is most commonly performed via the arterial approach (carotid artery cannulation via the femoral arterial route) and less commonly from a transvenous or transtorcular (directly through the occipital bone) approach. The goal of endovascular therapy is not to obliterate the shunt completely at the first setting ( Fig. 50.5 ) but rather to control symptoms. Often, more than one procedure is needed to result in complete occlusion or control of the shunt or shunts. Smaller-bore sheaths, catheters, and microcatheters enable the procedure to be safely performed in even small neonates. Most often the arterial feeding vessels are occluded with embolic liquid agents (e.g., N -butyl cyanoacrylate) to ensure that the very distal small feeding vessels are occluded. Coils are generally used as an adjunct to liquid embolization agents. Recently, use of a microvascular plug has been reported. In a large single-center experience from France, the mortality from endovascular treatment was nearly 11%, similar to the 12% found in a large meta-analysis. These procedures can be technically demanding, and complications of cerebral hemorrhage, cerebral ischemia, leg ischemia and cerebral vessel perforation can occur. All patients with VGAMs should be followed for life to monitor their neurodevelopment and cognitive function.

Pulmonary Arteriovenous Malformations
Background
Pulmonary arteriovenous malformations (PAVMs) are structurally abnormal vessels that bypass the pulmonary capillary bed through direct communication between the pulmonary artery and pulmonary vein and thus create an anatomic right-to-left shunt. These malformations result in a low-resistance, high-flow state causing impairment in gas exchange and impaired filtration of the systemic venous blood ( Fig. 50.6 ). The degree of right-to-left shunting causes varying degrees of hypoxemia, from asymptomatic to profoundly symptomatic. PAVMs predispose to complications such as paradoxical systemic embolization, stroke, and cerebral abscess. Treatment of asymptomatic PAVMs is recommended to prevent long-term complications, TCE is currently the accepted standard of care, and this can be achieved using low doses of x-ray radiation. PAVMs were historically considered to be rare based on early autopsy studies, but modern sophisticated diagnostic tools have suggested a prevalence of 2 to 3 per 100,000, with a male to female ratio ranging from 1 : 1.5 to 1.8. A population-based cancer screening program suggests the incidence to be 1 in 2630 of PAVMs that are sufficiently large to be detected by CT. PAVMs may be single or multiple, simple or complex, or diffuse, which are mostly confined to the lower lobes. The simple PAVMs receive blood from a single artery involving a single sac ( Figs. 50.7 and 50.8 ), whereas complex PAVMs can involve two or three arteries creating a plexiform mass of dilated vascular channels or tortuous communications between artery and vein (see Fig. 50.7 ). A small subset of patients have a more diffuse and severe type of PAVMs involving single or several segmental pulmonary arteries. The diffuse PAVMs are more common in both lungs (72%).



Most PAVMs are hereditary, occurring in 90% of patients with HHT, and the remaining are sporadic or idiopathic. HHT is the most common cause of PAVMs. It is a genetic disorder with an autosomal dominant trait, affecting 1 in 50,000 to 80,000 people. It is characterized by dermal, mucosal, and visceral telangiectasias and visceral AVMs (pulmonary, hepatic, gastrointestinal, and cerebrovascular). Gene coding mutations are responsible for HHT, and 85% of the cases are caused by one of three gene mutations: ENG coding for endoglin (HHT 1) on chromosome 9, ACVLR1/ALK 1 (HHT2) (Activin A Receptor, Type II-Like Kinase 1) mutation on chromosome 12, or SMAD4 (HTJP) gene coding on chromosome 18 that is associated with juvenile polyposis. There are currently two further unidentified genes causing HHT, mapped to chromosome 5q (HHT 3) and chromosome 7p (HHT4). The prevalence of PAVMs is related to the genotype of HHT. In one large study, PAVM was commonly associated with ENG mutation in 58% compared with only 18% with ACVL1/ALK 1 . The recognition of PAVMs has increased due to awareness of HHT, such that 59% of patients with PAVMs who were previously unaware were diagnosed with HHT. The HHT Foundation international guidelines working group established the Curacao criteria as the basis of diagnosis and an indication for subsequent genetic testing to confirm the diagnosis. The diagnosis of definite HHT is accepted if at least three of the following characteristics of the disease are met: (1) spontaneous and recurrent epistaxis, (2) family history of a first-degree relative with HHT, (3) cutaneous-mucous telangiectasia, and (4) visceral AVMs. Possible hypotheses for the pathogenesis of vascular malformations include impairment of blood vessel formation or imbalance in multiple proangiogenic and antiangiogenic factors, and delayed vascular remodeling with inappropriate proliferative response of the endothelial cells due to endoglin depletion or absence. This leads to the formation of blood vessels between an artery and a vein without intervening capillaries; these segments are fragile with high propensity for rupture and bleeding. These connecting blood vessels are termed telangiectasia for small-caliber and AVMs for large-caliber vessels.
Outside of HHT, PAVMs can also occur in patients with single ventricle variants who undergo superior cavopulmonary anastomosis in the form of a “classic” Glenn or bidirectional Glenn. In addition, PAVMs are particularly common in patients with single ventricle variants with interrupted inferior caval vein with azygous or hemiazygous continuation to the superior caval vein (typically in the setting of left isomerism of the atrial appendages) following the Kawashima procedure ( Fig. 50.9 ). In these patients, PAVMs can develop due to absence or paucity of hepatic venous effluent into the lungs. After inclusion of hepatic venous flow into the pulmonary circulation to complete the Fontan circulation, PAVMs may regress completely in some cases, whereas in others the anatomic pathway and flow dynamics can lead to streaming of hepatic venous flow primarily to a single lung, predisposing the opposite lung to PAVM formation (see Fig. 50.9 ). Unilateral PAVMs in patients who have already undergone Fontan completion may be correctable by reconfiguration of the pathway between the hepatic veins and the pulmonary arteries. Various approaches have been described, but the direct hepatic vein–azygous vein connection off-pump surgically through a lateral thoracotomy is probably the most reliable method of distribution of hepatic venous blood to both lungs.

PAVMs with dyspnea and hypoxemia are also seen in patients with hepatopulmonary syndrome (HPS). HPS is a complication of liver cirrhosis and portal hypertension and is seen rarely in acute and chronic hepatitis. The vascular pathology involves localized dilated pulmonary capillaries and less commonly discrete macroscopic PAVMs. The clinical triad that defines HPS comprises of portal hypertension with and without cirrhosis, hypoxemia, and intrapulmonary vascular dilations. The hypoxemia is defined by most as PaO 2 less than 70 mm Hg and an alveolar-arterial oxygen gradient greater than 20 mm Hg on room air. The precise pathogenesis causing HPS is unknown, and several vascular mediators, including nitric oxide, endothelin-1, and tumor necrosis factor-α, have been postulated to cause intrapulmonary vascular dilations resulting in right-to-left shunting and hypoxemia ( Fig. 50.10 ). The degree of hypoxemia does not correlate with the severity of liver disease. However, cirrhotic patients with HPS have higher mortality than patients without HPS. There are no definitive therapeutic options for HPS except for liver transplantation. Other causes of PAVMs include infections involving schistosomiasis, tuberculosis, actinomycosis, and Fanconi syndrome, and it is very rarely acquired due to trauma.
The clinical features seen in PAVM vary widely based on the size, number, and degree of shunting. Asymptomatic hypoxemia due to right-to-left shunting is the most characteristic clinical finding. Only a few patients demonstrate dyspnea or dyspnea with exertion. Commonly orthodeoxia (worsening hypoxemia when upright) and rarely platypnea (dyspnea relieved by lying down) are attributed to PAVMs because they are predominantly in the lower lung fields, with 17% in the upper and 83% in the lower lung zones. The classic triad of dyspnea, cyanosis, and clubbing is uncommon. Clinical examination can reveal cyanosis, clubbing, and telangiectasia involving the nails, skin, and mucous membranes of the mouth. A systolic or continuous murmur may be heard at the site of a superficially located PAVM or in the anterior or posterior basilar bilateral lung segments.
PAVM-associated neurologic complications are common and are grossly underrecognized. Several series have reported incidence of cerebral abscess to range from 7.8 % to 19%, with ischemic stroke rates of 9% to 18% and projected clinical stroke in at least 25% of patients with untreated PAVM by the age of 65 years; the stroke risk was reduced after embolization of the PAVMs. Interestingly, most of PAVM-related ischemic strokes or cerebral abscess occurred in patients who had not received the diagnosis of PAVMs. The median delay from a cerebral event to PAVM diagnosis was 2 years. Migraine headaches are relatively common in patients with associated PAVMs and can improve following treatment. The risk of ischemic stroke correlated directly with the severity of PAVM shunting. Paradoxical embolic events were more common with higher-grade contrast echocardiography (CE) shunts (grade 3 shunts) in patients with visible PAVMs seen on CT. These features were associated with a 10.4-fold increase in stroke/abscess compared with patients with grade 1 shunts who had no enhanced risk. The strongest stroke risk factors identified were: low serum iron due to inadequate iron intake from hemorrhagic losses, low pulmonary artery pressure likely facilitating paradoxical embolic process, and high serum fibrinogen, which promotes platelet addition. The organisms involved in PAVM-associated cerebral abscesses were anaerobic facultative organisms of periodontal origin. Risk factors identified for PAVM-associated cerebral abscess were male gender, poor dental hygiene and interventions, and lower SaO 2 . A relatively rare but potentially fatal complication is PAVM hemorrhage, leading to hemoptysis or hemothorax due to lack of structural integrity in the thin-walled layer of endothelium and weak connective tissue support. The highest risks for hemorrhage were in the setting of PAVM perfused at systemic pressures due to pulmonary hypertension or systemic arterial supply from bronchial or nonbronchial systemic arteries into the PAVM sac and during pregnancy. Other less common presentations or complications of PAVMs include pleuritic chest pain in 10% of patients with PAVMs, CHF secondary to high output from large extrapulmonary AVMs (primarily intrahepatic) and polycythemia secondary to hypoxemia. Chronic hypoxemia stimulates secondary erythrocytosis (polycythemia) to maintain arterial oxygen content. After embolization of PAVM, the erythrocytosis response abates with a fall in hemoglobin level within months. In addition, PAVM patients have impaired CO 2 clearance resulting in abnormally high ventilatory drive and increased minute ventilation on exercise. PAVMs patients have high cardiac output at rest and with exercise. During exercise, these patients use increased stroke volume, improved oxygen delivery secondary to increased hemoglobin and red cell mass, modified iron handling, and exuberant postural tachycardia as plausible mechanisms for adaptive exercise tolerance.
Investigations
The evaluation of PAVM is often sought for the investigation of respiratory symptoms such as dyspnea or hemoptysis, suspected right-to-left shunting in patients with cyanosis or HHT, or cerebral abscess and/or unexplained embolic stroke. A round or oval-shaped mass or nodule may occasionally be seen on chest radiograph; however, the PAVM needs to be fairly large to be detected in this manner, and many (10% to 40%) are not detected, making routine screening by chest radiograph insensitive. Several noninvasive methods to assess and quantify right-to-left shunting across the PAVMs include measuring arterial PaO 2 on 100% oxygen and perfusion scans using technetium-labeled albumin macroaggregates. Transthoracic CE is recommended by the international HHT guidelines as initial screening tool for PAVMs, with a sensitivity of 100% and the specificity of 67% to 91%. CE with agitated saline detects intrapulmonary shunting with the use of noninvasive ultrasound to visualize delayed appearance of microbubbles in the left side of the heart after three to four cardiac cycles. In contrast, the microbubbles are visualized within one to two cardiac cycles in patients with intracardiac shunt such as patent foramen ovale or an atrial septal defect. Chest CT scan, although not part of the PAVMs screening process, is widely considered the gold standard diagnostic tool for further evaluation of patients with a high suspicion of PAVMs to demonstrate size, location, and extent of PAVM prior to therapy. The higher resolution of CT is preferred to MRI. Pulmonary angiography is no longer considered necessary for diagnostic purposes alone and is solely reserved for therapeutic purposes after diagnosis is established.
Management
Percutaneous TCE is the gold standard for the treatment of PAVMs due to its effectiveness in reducing paradoxical embolism and its associated complications. Several series have demonstrated reduction in right-to-left shunting, improvement in oxygenation, reduction in strokes, migraines, reduced erythrocytosis, improved exercise tolerance, and prevention of lung hemorrhage. Therefore, regardless of symptoms, any PAVMs with feeding artery greater than 3 mm in diameter as detected by CT are generally recommended for TCE. During the procedure, if additional smaller PAVMs are found, the goal is to occlude as many feeding arteries as technically feasible, even those that do not conform to the “3 mm rule.” After obtaining femoral venous access, antibiotic prophylaxis is administered prior to PAVM embolization. The catheter is advanced to both pulmonary arteries, and baseline angiograms provide an overview of the number and distribution of PAVMs. Embolization of PAVMs is performed by targeting the supplying artery just proximal to the aneurysmal sac (see Fig. 50.8 ). A coaxial catheter system involving an outer guide catheter for stabilization and an inner catheter for deployment of the device is commonly used (see Figs. 50.8 and 50.10 ). Postdeployment angiograms are necessary to ensure all possible source arteries to the aneurysmal sac are occluded (see Figs. 50.10 and 50.11 ). Technical advances with the use of microcatheters, coils, and microvascular plugs have allowed occlusion of even small feeder arteries successfully (see Fig. 50.11 ).


Many devices have been successfully used to embolize PAVMs. Conventionally, coils were used for feeding arteries measuring 3 to 9 mm (see Fig. 50.8 ). However, PAVM sac persistence in up to 25%, recanalization, and collateralization rates of 5% to 19% can occur. Several series have indicated higher incidence of recanalization with coils when used singly or placed greater than 1 cm from the PAVM sac. In recent years, the Amplatzer vascular plug has become the preferred device for embolization due to its ability to occlude large-caliber vessels with a single plug ( Fig. 50.12 ), with precise occlusion at or proximal to the neck of the sac, thereby reducing procedure time and radiation exposure. A combination of embolization with vascular plugs and coils has demonstrated no recanalization 6 to 40 months after TCE. An alternate technique to occlude PAVMs and reduce risk of reperfusion is to block the draining venous sac. A recent study using this technique demonstrated no significant differences in PAVM characteristics, follow-up duration, and complications between the two techniques. However, the reperfusion of the PAVMs in the arterial group was 50%, and no reperfusion was encountered in the venous sac embolization group ( P < .1). Complications of TCE are low in experienced centers. Postembolization transient pleurisy is reported in 10%. Paradoxical embolism of devices, thrombi, or air bubbles is rare with current techniques. A rare postembolization complication includes massive hemoptysis from bronchial collaterals requiring systemic and pulmonary angiographic approaches for embolization. Pulmonary hypertension remains a relative contraindication for an elective TCE because it can lead to acute increase in pulmonary artery pressure. However, it can be carefully considered in select patients because it can reduce risk of hemoptysis due to rupture of the PAVM. Life-threatening complications, including PAVM bleed and stroke, are experienced in a small percentage of pregnant women, and 1% of pregnancies resulted in maternal and fetal death. For significant PAVM, third-trimester TCE is considered safe and feasible. Surgical resection of the PAVMs is uncommon nowadays because catheter embolization therapy has become the standard practice. Surgery is reserved for rare PAVMs deemed not suitable for embolization and is also used emergently to control hemorrhage. The surgical techniques include local excision, ligation, segmental resection, lobectomy, and rarely pneumonectomy.

Despite treatment with embolization or surgery of large PAVMs, small PAVMs persist and new PAVMs may be formed in patients with HHT. Therefore the use of antibiotic prophylaxis for procedural risks for bacteremia (dental procedures) is recommended. Other precautions that are warranted for patients with PAVMs include avoiding air bubbles during intravenous access and avoiding SCUBA diving due to risk of paradoxical emboli from decompression sickness. The follow-up evaluation of PAVMs could include CT scans at 6 to 12 months and every 3 years thereafter according to certain guidelines; however, in common practice, routine CT scans are not generally advocated due to the risks of radiation exposure. Alternative approaches include a modified management flow chart ( Fig. 50.13 ), use of MRI, and limiting use of CT scans for patients with symptoms, low oxygen saturation, or failure to obliterate a PAVM sac.

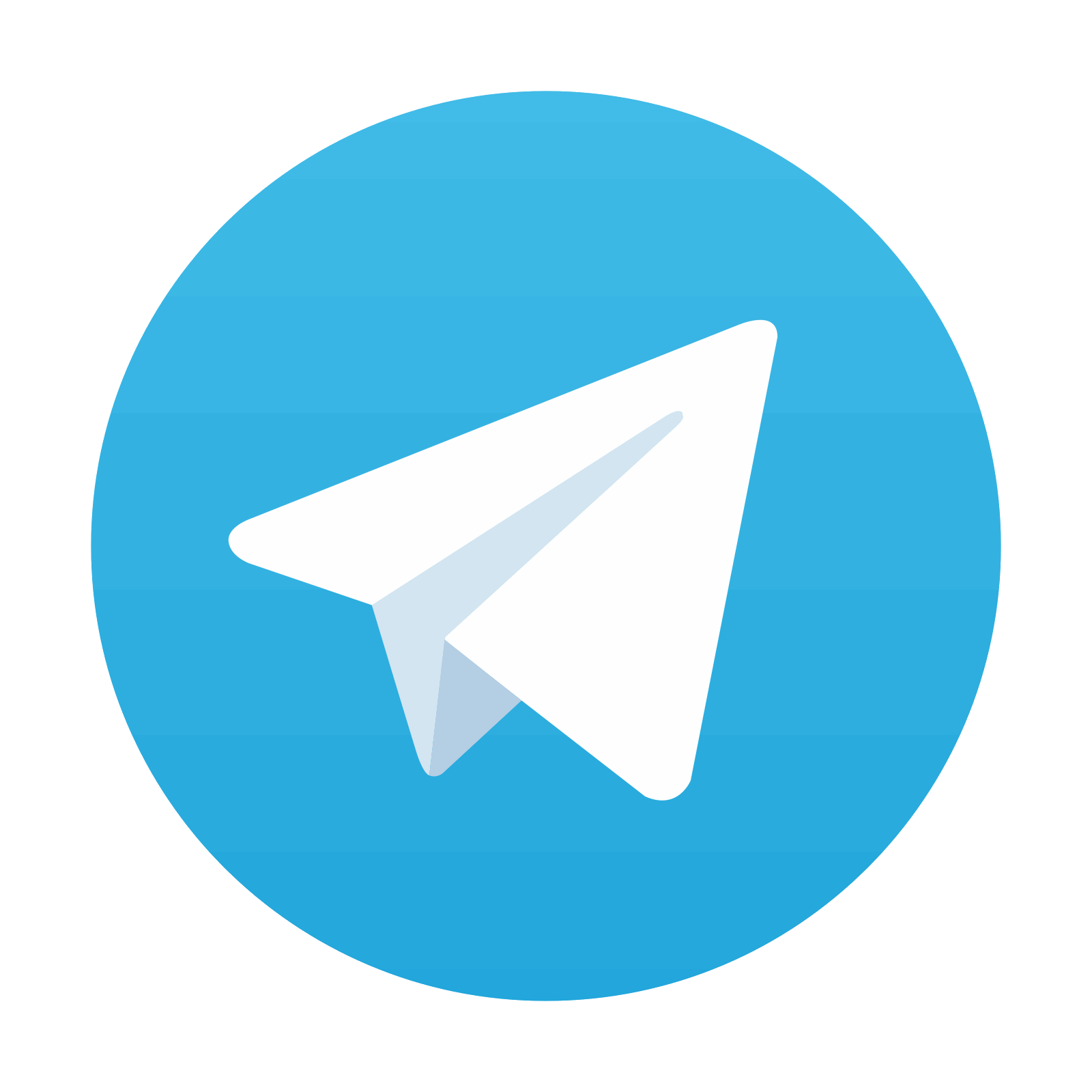
Stay updated, free articles. Join our Telegram channel

Full access? Get Clinical Tree
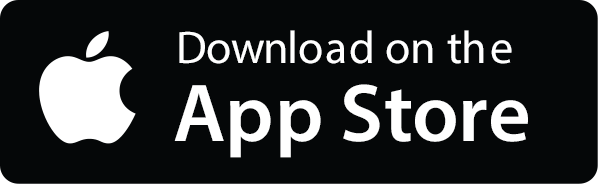
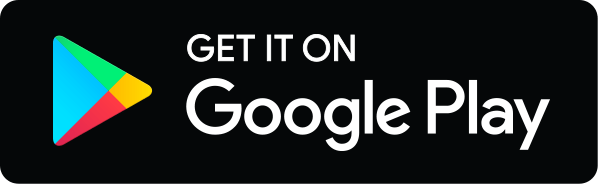
