Arteriosclerosis
Atherosclerosis
Stiffens aorta
Little effect
Dilates aorta
Occludes
Media
Intima
Diffuse
Focal
Age and blood pressure
Cholesterol and smoking
Cushion function
Conduit function
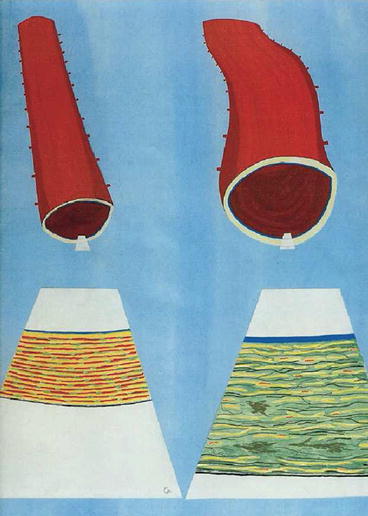
Fig. 4.1
Conceptual view of a young normal proximal aorta (left) and an elderly arteriosclerotic aorta (right) showing (above) dilation and wall thickening. Below is shown the orderly pattern of thick elastin fibres in the normal aorta with some muscular cells and collagenous fibres as wavy elements that do not bear any load at normal arterial pressure. In the thickened stretched arteriosclerotic wall at right, elastin fibres are fractured and fragmented. Collagenous fibres are stretched, and there is accumulation of amorphous material in the wall. The intima is thickened by hyperplasia of endothelial cells (Cartoon prepared by M O’Rourke and S Glagov. From Ref. [9])
In an editorial questioning the “comfort zone” of physicians [10], the Emeritus invasive cardiologist Spencer King referred to the future developments he expects to see from the use of engineering principles in this field. While concentrating on hydraulic factors and shear stresses which are involved in development of and damage to atheroscierotic plaques and which precipitate coronary acute events, he alluded to the predictability of other diseases, on the basis of engineering principles. One of them is arteriosclerosis, which Osler described as follows:
As an involution process arterio-sclerosis is an accompaniment of old age, and is the expression of the natural wear and tear to which the tubes are subjected. Longevity is a vascular question, which has been well expressed in the axiom that “a man is only as old as his arteries.” To a majority of men, death comes primarily or secondarily through this portal. The onset of what may be called physiological arterio-sclerosis depends, in the first place, upon the quality of arterial tissue (vital rubber) which the individual has inherited, and secondly upon the amount of wear and tear to which he has subjected it. [1]
The engineering principles that apply to development of arteriosclerosis from “wear and tear” (now quantifiable in terms of S/N (strain/cycle number) curves) are easier to understand than those that apply to rupture of an atherosclerotic plaque.
4.1 Cause of Arteriosclerosis
In seeking a cause for arteriosclerosis and an explanation for its development, one has to explain:
Why it predominantly affects the proximal thoracic aorta and not the peripheral muscular arteries
Why it affects the media of the aorta and not the intima or adventitia
Why it decreases distensibility of the aorta or (as put another way) why it increases aortic stiffness
Why it is associated with dilation of the aorta as well as increased stiffness
Why its major cause is aging
These issues are explicable and predictable on the basis of engineering principles of material fatigue, alluded to by Osler [1, 8] and King [10], as applied to inanimate objects, such as ships, bridges, aeroplane wing spars and even dance floors [6]. Each material object which is subjected to stretch or bending has a characteristic strain (S)/cycle number (N) or S/N curve. Natural rubber has properties similar to those of arterial elastin (indeed Osler referred to “vital rubber” as well as the effects of “wear and tear”) [1]. An S/N curve is shown in Fig. 4.2. The graph plots the properties of natural rubber in terms of the number of cycles of expansion to which it needs to be subjected in relation to the extent of strain, before fracture would be expected. The heart’s cycle is repeated some 30 million times per year and so some 1 billion times in 30 years. With the proximal thoracic aorta in youth stretching by some 15 % with each beat of the heart, one would expect that fracture of elastin fibres would begin to occur at age 30 years and progress steadily thereafter. One would also expect that peripheral muscular arteries (which dilate by <3 % at each cycle), or larger arteries such as the femoral, carotid or brachial which dilate by 3–5 % per cycle [11], would show little change in physical properties in a lifetime of 90 years.
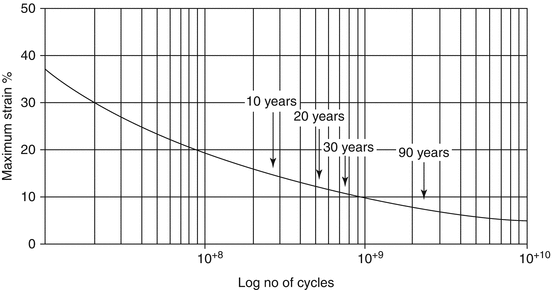
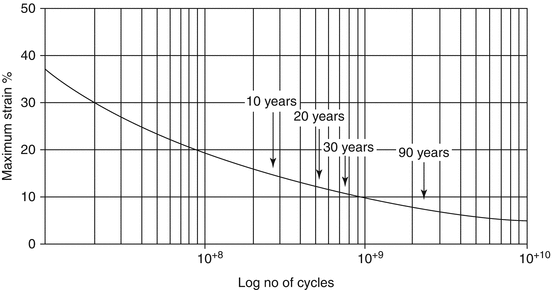
Fig. 4.2
Strain/cycle number (S/N) curve for natural rubber showing the relationship between distention and cycle number at which fracture of the rubber element is expected. The ascending aorta medial elastin fibres (if similar to natural rubber) which pulsate around 15 % with each beat would be expected to show evidence of fracture between 500 and 800 million cycles, corresponding to 20–30 years of life, whereas the distal muscular arteries which pulsate by <5 % with each beat would not be expected to show fracture in a lifetime of 100 years (From Ref. [6])
Such an explanation fits with the disorganisation of the elastin network in the aortic media with age (Fig. 4.1), and as seen in histology and pathology texts. This is regarded as a normal aging process “physiological arteriosclerosis”, unless extreme and unless associated with areas of medionecrosis, which is the substrate for aortic weakening and aortic dissection in the elderly [1, 6].
The explanation accounts for confining this process to the media since the intima and adventitia contain no elastin fibres (and do not oppose applied stress). It accounts for distension of the aorta since fracture of structural elements causes stretch. It accounts for increased stiffness of the aorta, since cycles of stretch are transferred to and borne by the collagenous fibres in the wall which have much higher modulus of elasticity (stiffness) than elastin. Physical damage to the proximal aorta is stressed here, whereas others such as Lakatta and colleagues tend to concentrate on cellular and molecular changes with aging [12]. I favour the physical (engineering) hypothesis since it explains the issues listed above. I see the physical damage to be primary and the chemical/cellular changes to be secondary and part of the attempt to repair the damaged aorta.
4.2 Effects of Aortic Change in Arteriosclerosis
Fracture of elastin elements in the aortic wall cause increase in elastic modulus. This appears to increase four- to tenfold over an average lifetime and so be greater than any other measurable effect of aging [6]. Stiffening of the aorta from change in elastic modulus increases aortic pulse wave velocity by an amount that depends on the square root of aortic modulus according to the classic Moens-Korteweg equation [6]:
where E is Young’s modulus of the wall, h is the wall thickness, r is the vessel radius and ρ is the blood density.
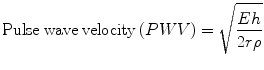
Hence, a ninefold increase in aortic modulus would increase aortic PWV threefold, and this explains the increase in aortic PWV with age from ~5 M/s to around 15 M/s at 90 years of age (Fig. 4.3).
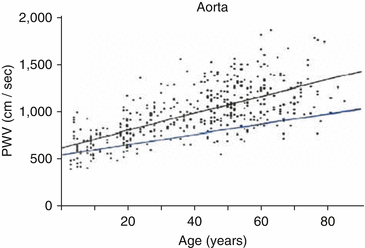
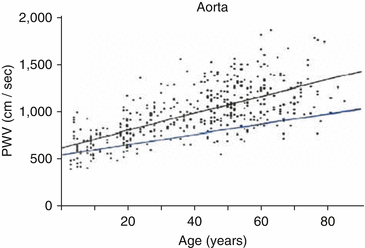
Fig. 4.3
Change in “aortic” carotid-femoral PWV with age in urban Beijing (individual data points and upper regression line), where hypertension is prevalent and salt intake high [13], and in rural Guangdong province where hypertension is less prevalent and salt intake is low (regression line at bottom). In Beijing, PWV increased approximately threefold between zero and 80 years. In rural Guangdong, PWV increased around twofold over the same period [13, 14] (From Ref. [9])
Increase in aortic PWV explains another characteristic change in the pulse waveform in older persons, with wave reflection returning early and augmenting the pressure wave in late systole. This is seen in Fig. 4.4 and is the cause of the change in radial artery pulse wave described by Mahomed, Broadbent, Osler and other physicians 100 years ago [6]: “The tidal wave is prolonged and too much sustained” [7]; “The pulse is slow in its ascent, enduring, subsides slowly” [8].
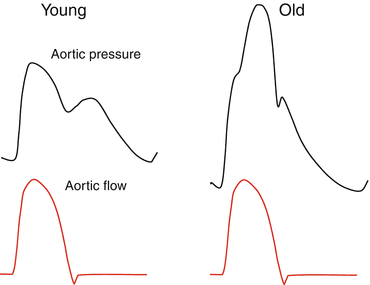
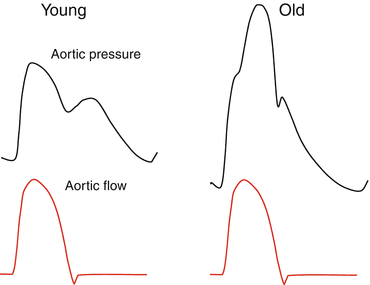
Fig. 4.4
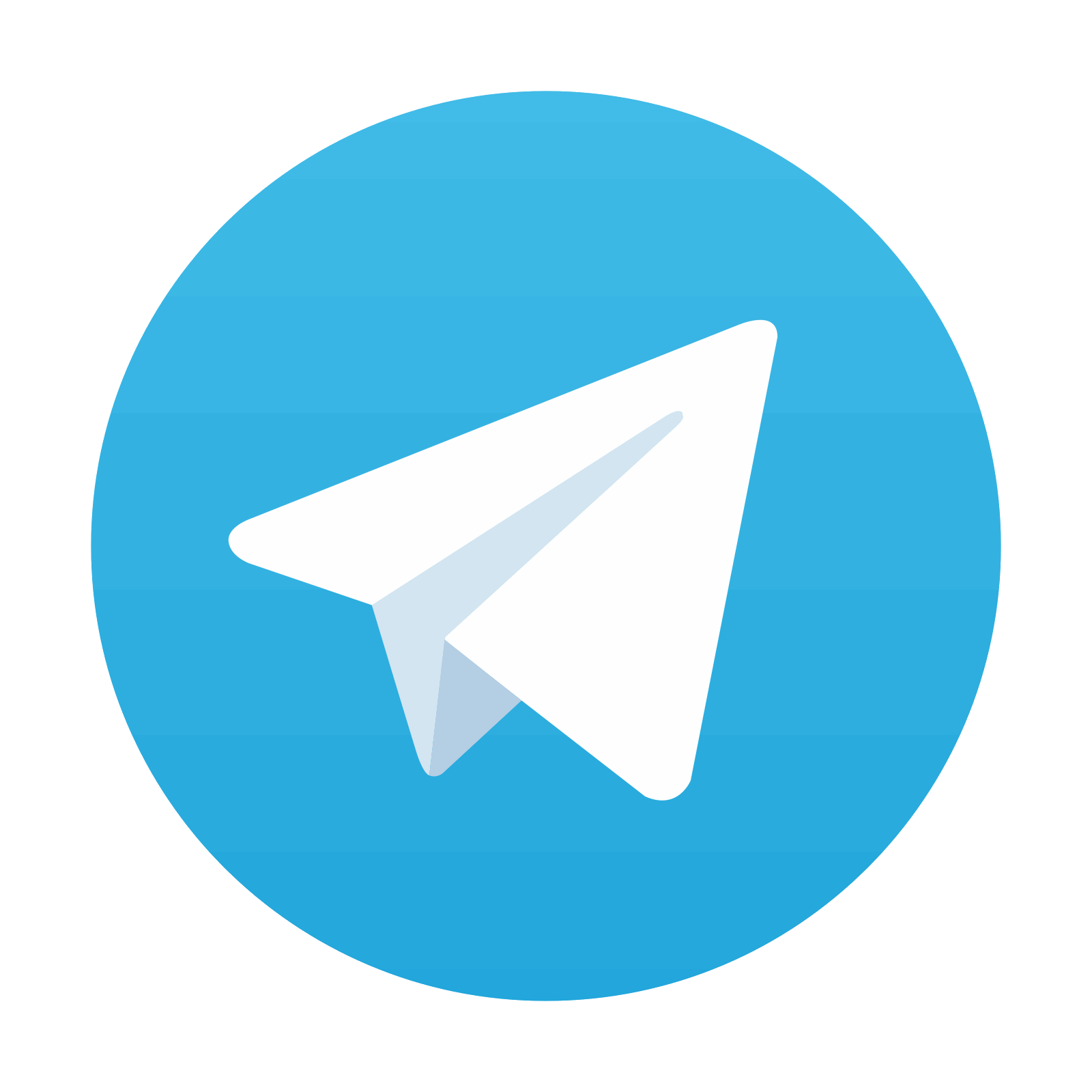
Schematic drawing of ascending aortic pressure waves (top) in a young (left) and old (right) subject for the same ejection (flow) wave from the heart (bottom, red). Increase in pressure from foot to initial peak or shoulder at ~100 msec later is higher in the old subject and attributable to high aortic characteristic impedance (Zc), while augmentation in late systole is due to early return of the reflected wave from peripheral sites, caused by increase in aortic PWV. In the younger subject, the initial pressure peak at ~100 msec forms the systolic peak, and the reflected wave returns at the end of systole and boosts coronary blood flow. In both young and old subjects, there is just one single spurt of blood from the LV into the aorta, but two distinct pressure pulses are created. The second pressure pulse must be due to wave reflection
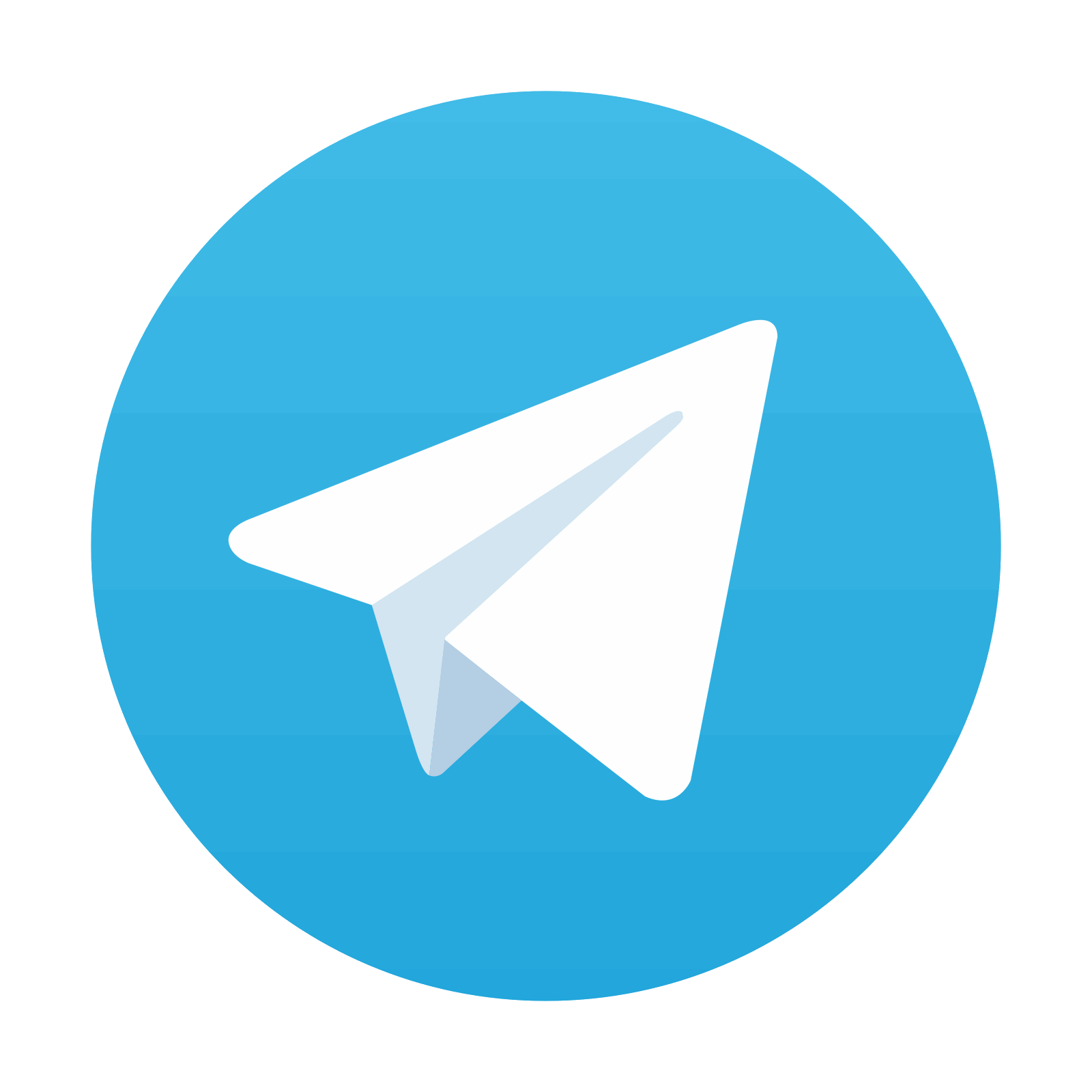
Stay updated, free articles. Join our Telegram channel

Full access? Get Clinical Tree
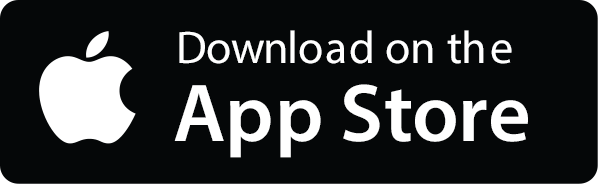
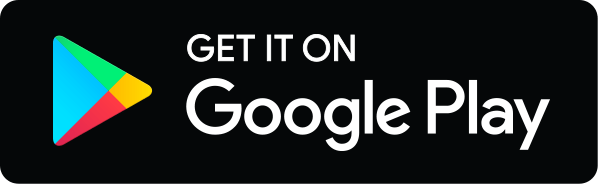
