Fig. 11.1
Osteochondrogenic BMP- Msx2-Wnt signaling in arterial calcifications
Molecular imaging in vivo has demonstrated inflammation-associated osteogenesis in early stages of atherosclerosis [38], confirming the role of inflammation in triggering the metabolic cascade leading to the transformation of VSMC into an osteogenic phenotype. Macrophage activation releases proinflammatory cytokines (such as IL-6 and TNFα) and proteolytic enzymes (metalloproteinases MMP 2, MMP 9, and cathepsin S) whose release is associated with osteochondrocytic VSMC transdifferentiation [23, 38]. IL-6 and TNFα are the first steps for the activation of BMP2:BMP4 and Msx2 which promote calcification by activating paracrine Wnt signals and nuclear activation and localization of β-catenin, an indispensable coregulator of expression of Runx2, osterix, and Sox9 which are all transcription factors associated with the osteochondrogenic phenotype conversion of VSMC and pericytes [23, 38–40]. The second aspect of inflammation-related calcification is the proteolytic activation of elastolysis and degradation of extracellular matrix. The fragmentation of elastic lamellae and release of biologically active elastin-derived peptides also promote VSMC dedifferentiation and calcium deposition [41].
In the presence of normal serum, VSMC do not calcify. Serum inhibits spontaneous calcium and phosphate precipitation in solution [42], indicating that systemic calcification inhibitors are present in the serum. VSMC which constitutively express potent local or systemic inhibitors of calcification [21], such as matrix GLA protein [43], may limit AC by binding to bone morphogenic proteins [44]. Osteopontin and osteoprotegerin are potent inhibitors of AC in vivo, and inactivation of their genes enhances the calcification process [45, 46]. Fetuin-A (AHSG or α2-HS glycoprotein) is a potent circulating AC inhibitor that is abundant in the plasma [47]. Pyrophosphate is another potent inhibitor. In vitro, phosphate-stimulated apatite production can be completely prevented by adding pyrophosphates that antagonize the cellular sodium–phosphate cotransport system [48].
While in the general populations the presence of cardiovascular calcifications could be observed in the absence of overt mineral metabolism disorders in several clinical conditions such as CKD/ESRD, the associations between vascular calcifications are associated with deterioration of mineral and bone metabolism caused by changes in serum phosphorus and calcium and disruption of endocrine and humoral pathways including parathyroid hormone (PTH), calcitriol, FGF-23/Klotho, and vitamin D. In vitro, calcium and phosphate promote both synergistically and independently VSMC calcification [28, 49]. Recent findings indicate that hyperphosphatemia, through activation of mitochondrial respiration, stimulates the production of ROS with final activation of NF-κB, enhancing Runx2 (Cbfa1) activation [50].
Hyperphosphatemia is tightly related to disruption of Klothe-FGF23 axis [51, 52]. Several experimental models and clinical conditions such as CKD are characterized by increased FGF23 and decreased Klotho activity [53, 54]. In animal models, Klotho and FGF23 knockout animals are characterized by short life span, accelerated aging phenotype, extensive arterial calcifications, osteoporosis, and hyperphosphatemia [52, 55, 56]. Suppression of phosphate from the diet or knockout of gene for Pit1 and sodium-dependent phosphate uptake restores normal life span and phenotype [52, 55]. Klotho deficiency increases expression of Pit1 and expression of Runx2 associated with secretory osteogenic phenotype of VSMC [53]. In animal models, FGF23 is not directly associated with vascular calcifications since neutralization of FGF23 with specific antibodies results in extensive arterial calcifications and premature death of animals [57].
Decreased Klotho expression and increased FGF23 precede the elevation of parathormone (PTH) secretion also responsible for mineral and bone homeostasis [58]. Chronic elevation of PTH upregulates RANKL and downregulates OPG gene expression and enhances the RANKL–OPG ratio, RANK–RANKL–OPG (receptor activator of nuclear factor (NF)-κB–receptor activator of NF-κB ligand–osteoprotegerin) signaling pathway, and the RANKL–OPG ratio [59–61]. Once bound to RANK, RANKL activates the alternative NF-κB pathway and initiates production of inflammatory cytokines and activates MSx2/Runx2 pathway. Results of a recent study demonstrated that RANKL increased VSMC calcification directly through BMP4 upregulation, providing autocrine stimulus and activation of Wnt signaling [62]. The arterial calcifications observed with increased PTH secretion are attributed to RANKL-mediated bone resorption by osteoclast-associated excessive calcium and phosphate releases.
11.2 Bone–Vascular Cross-Talk
There is growing clinical and experimental evidence linking bone pathology and different functional and structural characteristics of cardiovascular system. Several population-based longitudinal studies demonstrated associations between osteoporosis and AC or arterial stiffness, as well as an association between the progression of aortic calcifications and decreased bone mineral density [14–17, 63–65]. The mechanisms accounting for these associations are not well understood. Several possibilities should be considered: (1) common risk factors for osteoporosis or bone remodeling and vascular calcifications, (2) role of primary vascular pathologies on bone function and remodeling, and/or (3) the participation of bone cells in vascular remodeling.
11.2.1 Common Factors
Clinical data show that osteoporosis and vascular calcifications are influenced by several common risk factors, such as age, menopausal status, diabetes, dyslipidemia with inflammation, and oxidative stress as the final common mechanisms [27, 66–69].
Aging is associated with bone loss and with development of vascular calcifications especially in postmenopausal women [66, 67]. Nevertheless, this bone–vascular association remained significant after adjustment for age suggesting a biologically linked phenomenon [14, 16, 65]. Aging is the most typical condition associated with the development of vascular calcifications. VSMC senescence is associated with the switch to a secretory phenotype (senescence-associated secretory phenotype, SASP) linked to low-grade arterial inflammation with production of proinflammatory cytokines associated with AC by activation of BMP 2/4 and Wnt/β-catenin signals [32, 33]. Direct evidence that inflammation was the factor linking bone remodeling and arterial calcifications was recently provided by Hjortnaes et al. who, using near-infrared fluorescence molecular imaging, showed that arterial and aortic valve calcifications inversely were correlated with osteoporotic bone remodeling [69]. Chronic inflammation is also associated with unbalanced bone formation and bone resorption [70].
Normal bone remodeling is characterized by a balance between osteoclast bone resorption and osteoblast bone matrix deposition. This balance is disrupted in osteoporosis and is influenced by the RANKL–OPG equilibrium. The discovery that OPG-deficient mice develop severe arterial calcifications concomitantly with severe osteoporosis, cortical and trabecular bone porosity, and their high fracture rate provided robust evidence pointing to the RANKL–OPG disequilibrium as a possible common factor linking osteoporosis and arterial calcifications [71]. RANKL activates the alternative NF-κB pathway and initiates production of inflammatory cytokines and arterial calcifications in parallel with RANKL-mediated bone resorption by osteoclasts whose apoptosis is suppressed osteoclast apoptosis. The association between arterial calcification and osteoporosis is most typically observed in postmenopausal women. It could largely reflect estrogen deficiency since estrogen inhibits RANKL-signaling and induces osteoblast OPG expression [72].
Osteopenia, poor fracture healing, arterial calcifications, and higher risk of hip fractures are frequently found simultaneously in patients with diabetes mellitus [73]. Increased AGE accumulation could be the common factor linking bone and arterial pathologies in diabetes. Endogenous ligands for AGE receptors (RAGE) trigger activation of transcription factor NF-κB and ROS signaling, leading to the production of proinflammatory cytokines and activation of VSMC osteogenic BMP/Wnt signaling through Runx2 upregulation inducing AC [74]. In vitro, through their accumulation in diabetes, AGEs stimulate osteoblast apoptosis and modify osteoclast activity by delaying bone regeneration and contributing to defective bone formation [75, 76].
11.2.2 Arterial Disorders and Bone Alterations
Atherosclerosis also affects bone circulation and impaired bone perfusion. With aging, bone arteries and arterioles are subjected to arteriosclerosis, with reduced bone marrow blood supply that renders the marrow ischemic and diminishes the cortex blood supply, which is replaced by the periosteal circulation [77]. That a link between compromised intraosseous circulation and consequent osteoporosis might exist is also suggested by the observation associating decreased bone mineral density and peripheral artery disease [78]. Intraosseous angiogenesis and bone remodeling are regulated by similar cytokines and growth factors, and bone formation–resorption and blood supply are tightly associated [79]. Some results showed that, in healthy women, bone perfusion indices were lower in subjects with osteoporosis than those with osteopenia or normal bone–mineral density [79, 80].
11.2.3 Bone Functions and Arterial Alterations
Several experimental findings support that bone and osteoblast physiology are involved in the control of fat-tissue metabolism and adipokine release, energy expenditure, and insulin secretion and sensitivity, all factors directly affecting cardiovascular function and health. Lee et al. [81] showed that osteoblasts exert endocrine regulation on energy metabolism, with osteocalcin (OCN) playing an important role. Uncarboxylated osteocalcin can regulate the expression of insulin genes and β-cell proliferation and adiponectin (ADPN) release and expression by adipocytes [81, 82]. In CKD patients, serum OCN was positively associated with serum ADPN [83]. ADPN is anti-inflammatory, suppresses atherosclerosis, increases insulin secretion and sensitivity, and activates osteoblastogenesis [84]. Moreover, ADPN regulates arterial calcifications [85], and an inverse relationship was observed between ADPN and the progression of coronary calcifications [86]. In ESRD patients, cardiovascular calcifications are frequently observed in the presence of low bone volume and adynamic bone disease, characterized by decreased osteoblast numbers/activity [15, 87] suggesting that bone cells could influence vascular function and calcification. In a recent study in ESRD patients, it has been shown that peripheral artery disease with extensive calcifications is associated with low osteoblastic activity characterized by pronounced osteoblast resistance to PTH [87].
In conclusion, the results of cross-sectional studies on general populations and several clinical conditions showed an association between atherosclerosis/arteriosclerosis and arterial calcifications. The two types of calcifications, i.e., intimal and medial, have a different impact on arterial functions. The intimal calcification as a part of advanced atherosclerosis results in the development of plaques and arterial lumen decrease or occlusion and ischemic lesions downstream. Medial calcifications result in the stiffening of arterial walls with increased systolic and decreased diastolic pressures. Arterial changes occur in relation with mineral and bone disorders, including osteoporosis, low bone volume, and high or low bone activity. The pathophysiology and biological links between bone and arterial abnormalities suggest the existence of bone-vascular cross-talk and common regulatory factors shared by vascular and bone systems.
This leads to cardiac pressure overload, left ventricular hypertrophy, and decreased myocardial perfusion. The two types of calcifications are associated with increased mortality.
References
1.
Briet M, Boutouyrie P, Laurent S, London GM (2012) Arterial stiffness and pulse pressure in CKD and ESRD. Kidney Int 82:383–400CrossRef
2.
Hayden MR, Tyagi SC, Kolb L et al (2005) Vascular ossification – calcification in metabolic syndrome, type 2 diabetes mellitus, chronic kidney disease, and calciphylaxis – calcific uremic arteriolopathy: the emerging role of sodium thiosulfate. Cardiovasc Diabetol 4:1–22CrossRefPubMedCentralPubMed
3.
4.
5.
7.
8.
9.
10.
11.
12.
13.
14.
Hak AE, Pols HA, van Hemert AM et al (2000) Progression of aortic calcification is associated with metacarpal bone loss during menopause: a population-based longitudinal study. Arterioscler Thromb Vasc Biol 20:1926–1931CrossRefPubMed
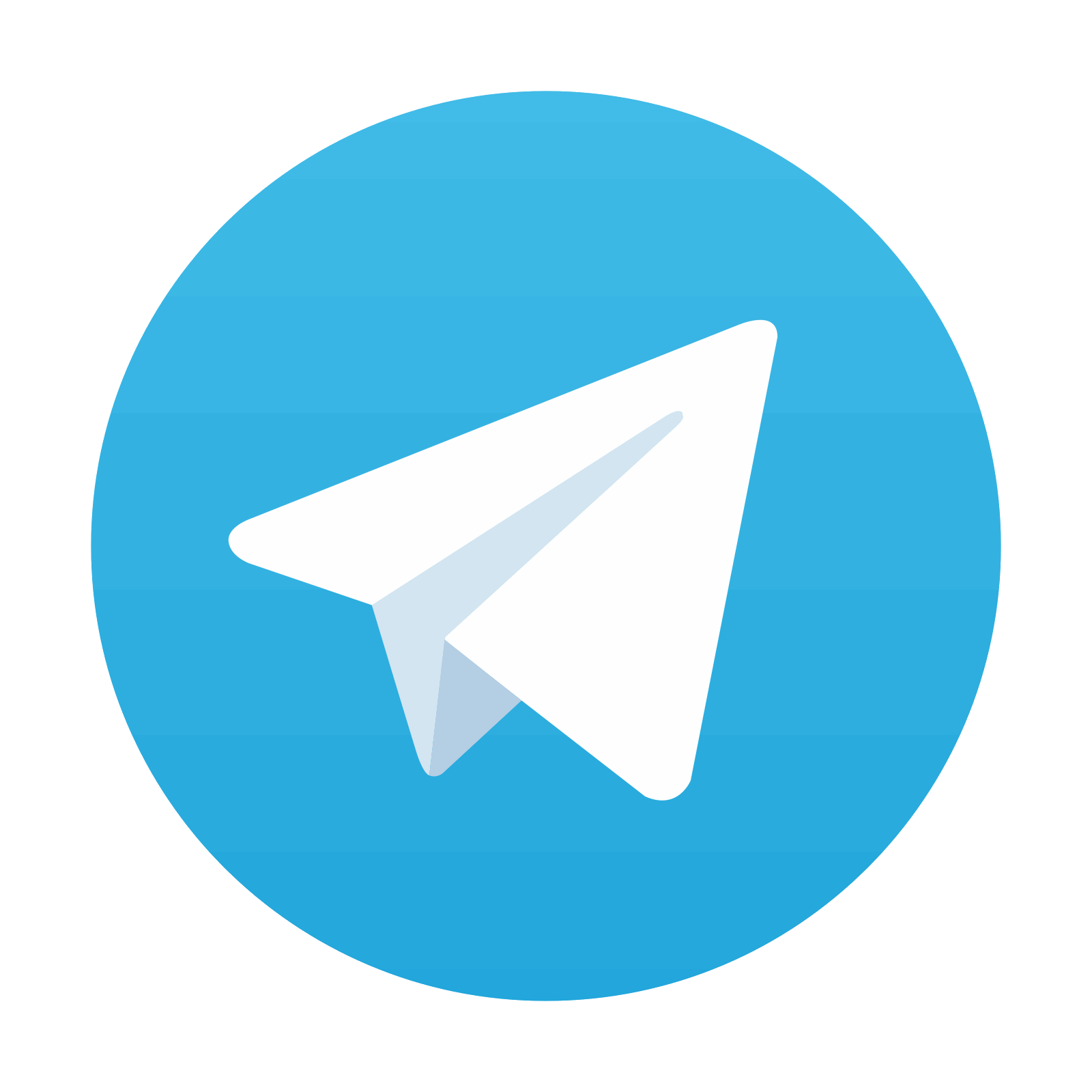
Stay updated, free articles. Join our Telegram channel

Full access? Get Clinical Tree
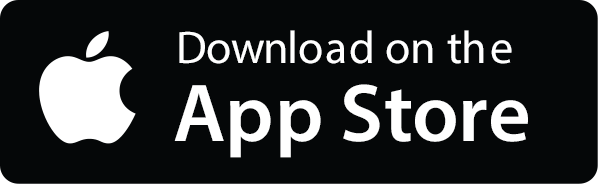
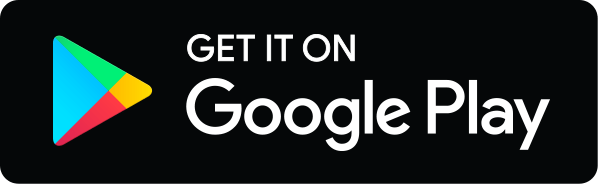