Fig. 26.1
Central aortic pressure-flow relations. Volumetric flow into the aorta is assessed by multiplying left ventricular outflow tract flow velocity (a) and area computed from diameter (b). The resulting volumetric flow waveform is paired with carotid artery tonometry and used to compute input impedance (c). Characteristic impedance (Z c) is estimated by taking the ratio of change in pressure and change in flow prior to flow reaching 95 % of the peak value (t Q95), which is prior to the inflection point (t i) indicating arrival of the global reflected wave. Once Z c is known, it is possible to separate forward and reflected pressure and flow waves (d)
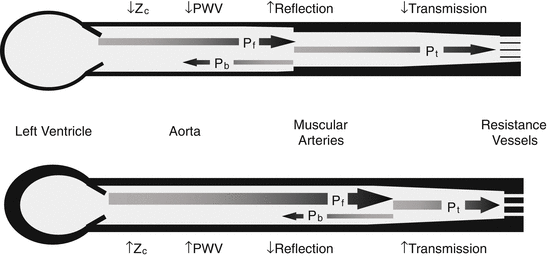
Fig. 26.2
Protective effect of wave reflection. Normal aortic stiffness is much lower than muscular artery stiffness (portrayed as differences in wall thickness). The resulting impedance mismatch produces proximal wave reflection, resulting in reflection of a component of waveform power (P b), which limits the proportion of forward pulsatile power (P f) that is transmitted (P t) into the periphery, where it may cause damage (a). When aortic stiffness exceeds muscular artery stiffness, impedance mismatch is reduced, and a greater proportion of the pulsatile power is transmitted into the periphery (b)
With advancing age, the aorta stiffens to a highly variable degree for reasons that are only partially elucidated but may be related to mechanical fatigue of elastic fibers [4], inflammation [5], fibrosis, cross-linking of structural proteins within the aortic wall [6], and smooth muscle cell hypertrophy [7] or stiffening [8]. As a result, Z c and forward wave amplitude (P f) increase after midlife [9], resulting in an increase in the pulsatile energy content of the advancing waveform. In addition, stiffening of the aorta with age greatly exceeds stiffening of the muscular arteries, which reduces impedance mismatch and wave reflection at this critical interface [10]. Loss of wave reflection means that a greater percentage of pulsatile energy is transmitted into the periphery (Fig. 26.2), which is particularly deleterious for obligate high-flow organs such as the brain and kidney [1]. High-flow organs are necessarily of low impedance, meaning that a greater proportion of the pulsatility that passes through the critical first stage barrier at the aorta-muscular artery interface will be transmitted across the second stage barrier provided by the resistance vessels and may damage the microcirculation [2].
A number of measures of arterial function have been proposed to characterize the hemodynamic abnormalities summarized above. Aortic wall stiffness is readily assessed by measuring carotid-femoral pulse wave velocity, which is strongly related to incident hypertension [11] and cardiovascular disease (CVD) events [12]. The balance between aortic diameter, stiffness, and flow can be assessed by measuring forward pressure wave amplitude and Z c. The amount of flow pulsatility penetrating into peripheral organs can be assessed by analysis of flow using Doppler ultrasound or magnetic resonance imaging [2, 3]. Wave reflection can be assessed by examining the central pressure waveform to determine augmented pressure and augmentation index (AI) or more precisely by measuring central pressure and flow waveforms [13]. Measured central aortic pressure and flow allows for separation of forward (P f) and backward (P b) pressure waves in order to determine relative wave reflection, for example, by calculating the global reflection coefficient, RC = P b/P f [14]. In addition, one can measure carotid intima-media thickness (CIMT) and ankle-brachial index (ABI) as measures of large artery wall hypertrophy and atherosclerotic disease. Finally, one can use multidetector computerized tomography (MDCT) to evaluate calcification in the aorta and coronary arteries as measures of structural damage and atherosclerosis.
26.2 Measures of Aortic Stiffness and Pressure Pulsatility
26.2.1 Carotid-Femoral Pulse Wave Velocity
CFPWV has been associated with CVD risk and risk reclassification in various studies and in a recent individual participant meta-analysis and has emerged as the reference standard measure of aortic stiffness [12]. In part, the ability of CFPWV to reclassify risk stems from the observation that CFPWV is only modestly associated with standard risk factors [15], although there are modest associations of higher CFPWV with greater age, mean arterial pressure, heart rate, inflammation, and components of the metabolic syndrome. In addition, CFPWV is moderately heritable and various genetic loci may contribute to increased aortic stiffness [16]. In the Framingham Offspring Study, having CFPWV in the highest (>12 m/s) as compared to the lowest (<8 m/s) quartile was associated with markedly increased risk for a first major CVD event (HR = 3.4; 95 % CI, 1.4–8.3; P < 0.008) in a model that adjusted for standard risk factors [17]. Prevalence of CFPWV >12 m/s is low prior to 50 years of age but increases to >60 % after 70 years of age [18], suggesting that a substantial and growing burden of disease may be attributable to excessive aortic stiffness in our aging society.
CFPWV is readily measured in a clinical setting in just a few minutes with relatively modest requirements for specialized equipment and expertise. Transit time is measured by performing tonometry of the carotid and femoral arteries. The distance between recording sites is measured and CFPWV is computed as transit distance divided by transit time. Transit distance must account for parallel transmission of the advancing wavefront around the aortic arch and up the brachiocephalic and carotid arteries. The linear distance from carotid to femoral artery overestimates true transit distance. Compensation for parallel transmission is commonly achieved by using the suprasternal notch as a fiducial point for the waveform separation site and measuring upward to the carotid site and downward to the femoral site. The difference between these distances represents the corrected transit distance. An alternative approach measures the linear distance from carotid to femoral site and then rescales the distance by an empirically derived factor of 0.8 [19]. The latter approach has the potential limitation of not accounting for variability in individual anthropomorphic factors and operator positioning of the tonometer on the neck.
26.2.2 Pressure and Flow Pulsatility
European guidelines recommend consideration of pulse pressure (PP, the difference between systolic and diastolic blood pressure) when evaluating risk for CVD and when managing CVD and hypertension; PP ≥ 60 mmHg is considered evidence of target organ damage in older patients [20]. PP and CFPWV are related but distinct measures of aortic function that contribute separately to risk. CFPWV is closely related to aortic wall stiffness but is relatively insensitive to the lumen area. In contrast, determinants of PP, such as Z c and P f, are strongly dependent on the aortic lumen area and matching between the aortic lumen area and ambient flow. Individuals with higher PP have higher aortic flow and ventricular dimensions and yet a smaller aortic lumen area, resulting in mismatch between the resting peak systolic flow and lumen area of the aorta, increased P f amplitude, and increased PP [21].
Central as compared to peripheral PP has been recommended as a potentially superior tool for risk stratification. However, although absolute values for central and peripheral PP may differ because of the effects of variable timing of wave reflection, differences are modest from midlife onward, and the two values are highly correlated [22]. As a result, it is difficult to demonstrate that one or the other provides superior risk prediction. Placing the focus on increased awareness of the clinical implications of wide PP and other independent measures of aortic stiffness, such as CFPWV, rather than minor potential differences between central and peripheral PP, seems warranted.
26.2.3 Global Wave Reflection, AI, and Augmented Pressure
Measures of wave reflection are among the most frequently misunderstood and misinterpreted indicators of arterial system function. Relative wave reflection is often assessed from pressure alone by evaluating AI from a central aortic pressure waveform (Fig. 26.1c). Higher AI was originally attributed to premature arrival of the reflected pressure wave because of increased aortic PWV. However, subsequent studies in large community-based samples have demonstrated modest relations between AI and CFPWV. In particular, after 50 years of age, when CFPWV increases dramatically, AI plateaus or falls [10, 13]. These seemingly counterintuitive relations between AI and aortic stiffness are attributable in part to a reduction in impedance mismatch and amount of wave reflection after midlife, when aortic stiffness increases to the point that CFPWV reaches and then exceeds muscular artery PWV, resulting in reversal of the arterial stiffness gradient that normally gives rise to proximal wave reflection (Fig. 26.2).
In contrast to AI, augmented pressure continues to rise after midlife [13]. However, augmented pressure (or the separated P b) amplitude depends strongly on P f amplitude. If one rearranges the expression for global RC, one finds that P b = P f * RC. Given that P f increases dramatically after midlife, whereas RC falls modestly, it is clear that the increase in P b after midlife is primarily attributable to larger P f rather than increased wave reflection per se, as assessed by the RC. Thus, a late life increase in augmented pressure represents ratiometric rescaling of the central pressure waveform, with a constant percentage of PP attributable to augmentation. In addition, recent studies have shown that AI and augmented pressure amplitude are related to left ventricular function [23, 24]. P b augments pressure and decelerates flow. The net effect on augmented pressure depends on left ventricular function and the left ventricular ejection pattern [13, 23]. Factors that limit ventricular ejection in late systole can therefore have a major effect on augmented pressure and AI even in the absence of alterations in P b. Relations between ventricular function and augmentation may offer a mechanism for reducing central pressure that is not dependent on transferring the excess pulsatile energy into the microcirculation, as occurs with peripheral vasodilator drugs.
Measures of wave reflection have similarly complex relations with CVD risk. Early studies demonstrated relations between AI and clinical events in select samples comprised of patients on dialysis [25] or with known coronary artery disease [26]. In addition, some recent community-based samples have found relations between measures of wave reflection and CVD outcomes [27–29], whereas others have not [17]. However, studies that found relations focused on P b rather than a measure of relative wave reflection such as AI or the RC. As a result, the relation between P b and events may be heavily dependent on P f. Others used an imputed or estimated flow waveform rather than measured flow [27, 29], which may contribute to misclassification of wave reflection because of the dependence of the flow waveform shape on left ventricular function [30].
In contrast to the question of risk stratification, when evaluating the effects of various interventions on blood pressure and clinical events, consideration of the differential effects of therapy on central as compared to peripheral blood pressure may be important. Drugs that reduce the amount of wave reflection or the ventricular response to a given reflected wave may have a greater effect on central as compared to peripheral systolic and PP. However, no study performed to date has demonstrated that differential lowering of central as compared to peripheral blood pressure is associated with a differential response to therapy that can be attributed to a more favorable effect of therapy on central as compared to peripheral blood pressure.
26.3 Measures of Atherosclerosis and Arterial Remodeling
26.3.1 Carotid Intima-Media Thickness (CIMT)
Carotid artery imaging provides a direct assessment of arterial wall remodeling and hypertrophy and the presence and severity of atherosclerosis. CIMT is measured in regions of the artery that are free of identifiable focal plaque, most often in the common carotid artery. CIMT represents a variable combination of medial hypertrophy and intimal thickening. Focal plaque provides clear evidence of atherosclerosis [31]. Plaque volume and characteristics may contribute separate information regarding risk. Echolucent plaques are lipid-rich and confer higher risk, whereas echogenic, fibrous plaques tend to be more stable [32, 33]. A recent individual participant data meta-analysis evaluated CVD risk reclassification offered by IMT of the common carotid based on data from 14 cohorts and 45,828 individuals with 4,007 events [34]. CIMT was associated with modestly higher risk for myocardial infarction and stroke. However, reclassification analysis demonstrated minimal net reclassification improvement in 10-year risk classification. Even in the subgroup at intermediate risk, net reclassification was small (3.6 %, 95 % CI, 2.7–4.6 %) and not likely to be clinically relevant [34].
26.3.2 Coronary Artery and Aortic Calcification
Calcification in the coronary arteries is located mostly within complex atherosclerotic plaques and associated with increased risk for adverse atherosclerotic events. Importantly, lack of calcification does not rule out the presence of disease because some plaques are not calcified; thus, coronary calcification should be used for risk stratification in the appropriate patient group (asymptomatic, intermediate risk) [35, 36]. Recent guidelines have included evaluation of coronary calcification as an option that may upstage CVD risk in those at intermediate risk [37]. However, evaluation of calcification requires exposure to radiation and therefore carries a risk that must be considered in the context of potential benefits of screening. To date, no prospective, randomized study has shown that a strategy of therapy guided by the presence of coronary calcium improves clinical outcomes.
Calcification in the aorta can occur in the intimal plaque or the media. Medial calcification is a complex, regulated process that is dysfunctional in various disease states and conditions, such as diabetes and chronic kidney disease [38]. Medial calcification may represent a cause and a consequence of arterial stiffness, leading to a potential vicious cycle that could contribute to the accelerated rate of aortic stiffening and calcification after midlife. Aortic stiffening and calcification may contribute to difficulty treating systolic hypertension in older people [39].
26.3.3 Ankle-Brachial Index (ABI)
The presence of flow-limiting lower extremity peripheral artery disease (PAD) can be confirmed noninvasively by an ABI <0.9. ABI is calculated by taking the maximum of systolic pressures in the posterior tibialis and dorsalis pedis arteries of each leg and dividing by the maximum systolic pressure in the arms, which is used as a common denominator in order to minimize confounding of ABI by upper extremity stenoses. Since PAD is often asymptomatic or associated with atypical symptoms, using ABI to screen for disease seems logical. However, studies performed to date have failed to demonstrate that ABI screening substantively reclassifies CVD risk [40–42]. Failure of ABI to fulfill criteria for a new risk biomarker is likely a consequence of strong relations between standard risk factors and abnormal ABI, i.e., rather than identifying a novel causal mechanism that contributes to CVD pathogenesis, abnormal ABI signifies rather advanced downstream consequence of risk factor exposure. For example, in the PARTNERS (PAD Awareness, Risk, and Treatment: New Resources for Survival) Study, screening of 6,979 adults >70 years of age or 50–69 years of age with diabetes identified 1,865 (29 %) with an ABI <0.9 [43]. However, the majority of participants with ABI <0.9 had clinically evident CVD or diabetes, indicating that they were already known to be high risk. The Aspirin for Asymptomatic Atherosclerosis trial used ABI to screen nearly 30,000 community-dwelling adults aged 50–75 years with no history of CVD and identified 3,350 participants with ABI <0.9 who were randomly assigned to receive aspirin or placebo [44]. There was no difference in CVD outcomes, indicating that screening was not an effective means for identifying risk and directing preventive therapy.
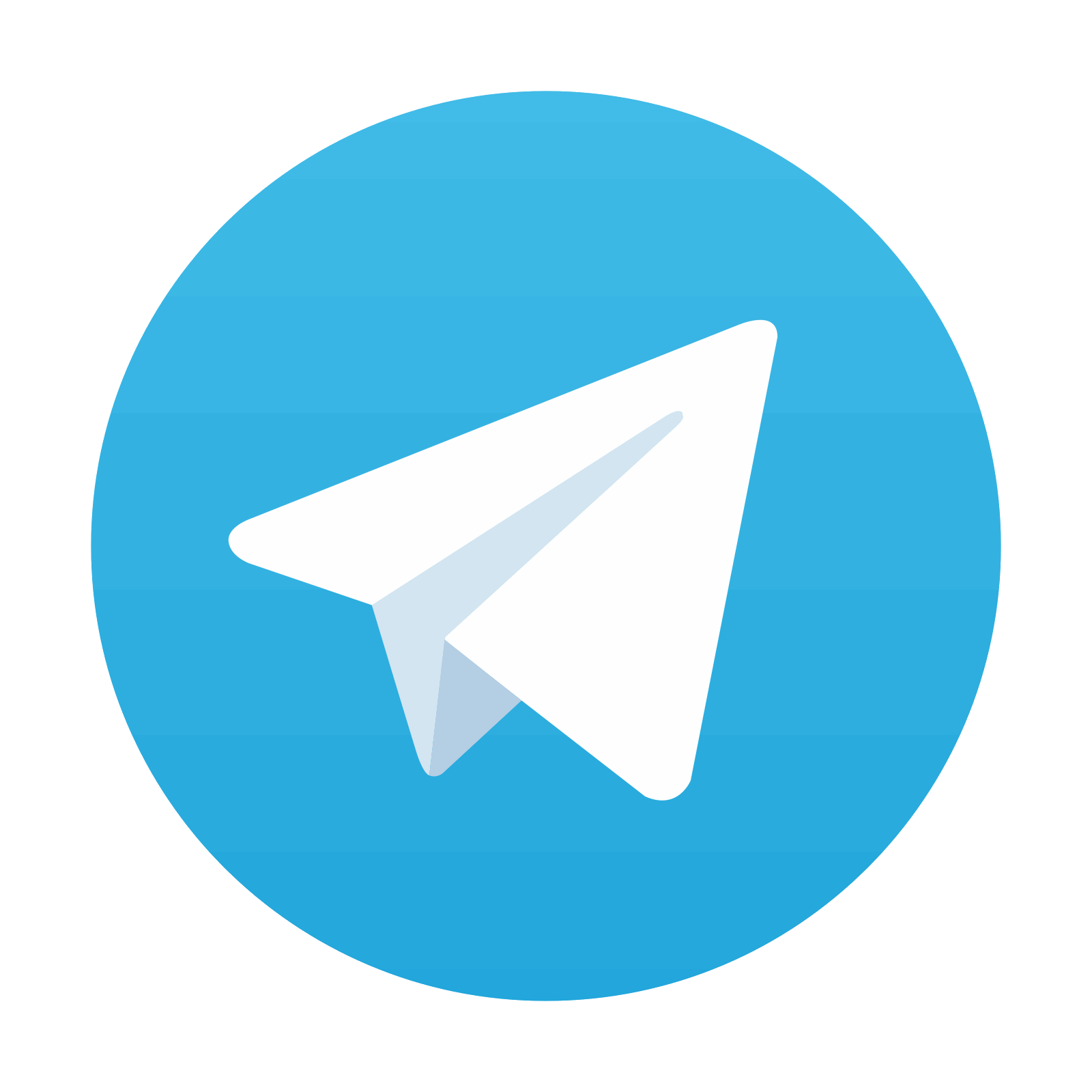
Stay updated, free articles. Join our Telegram channel

Full access? Get Clinical Tree
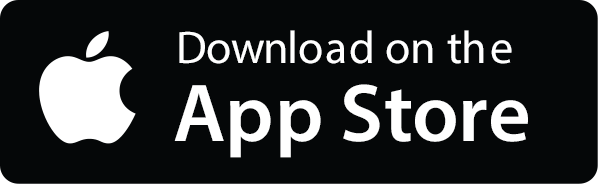
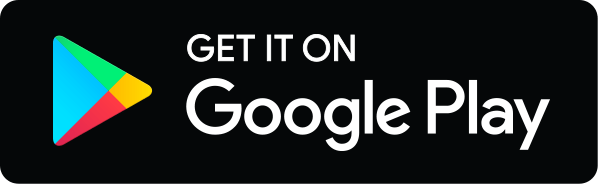