Abstract
Hypertrophic cardiomyopathy (HCM) is characterized by a thickened but nondilated left ventricle in the absence of any other cardiac or systemic condition capable of producing the magnitude of hypertrophy evident (e.g., aortic valve stenosis, systemic hypertension, some expressions of athlete’s heart, infiltrative or storage disorders).
HCM is the most common genetic cardiovascular disease. HCM is transmitted as an autosomal dominant trait with variable expressivity and age-related (incomplete) penetrance. To date, more than 1500 disease-causing mutations have been identified in genes that encode proteins of the thick and thin contractile myofilament components of the cardiac sarcomere or the adjacent Z-disc.
HCM is a complex and clinically heterogeneous disease that demonstrates remarkable diversity in disease course, age of onset, severity of symptoms, left ventricular outflow obstruction, and risk for sudden cardiac death (SCD). HCM is the most common cause of SCD in the young and is the leading single cause of SCD in competitive athletes in the United States, accounting for approximately one-third of athletic field deaths. Myofibrillar disarray as well as diffuse interstitial myocardial fibrosis or replacement scar have been suggested as factors contributing to reentry and ventricular arrhythmogenesis in HCM.
No treatments to prevent or modify disease progression currently exist. Therefore current treatment focuses on symptom management, assessment for risk of SCD, and family screening. Implantable cardioverter-defibrillator insertion is recommended for secondary prevention in patients with prior cardiac arrest or sustained ventricular tachycardia, and for primary prevention in patients with certain risk factors.
Keywords
hypertrophic cardiomyopathy, genetic mutations, sarcomere, athlete’s heart, genetic screening, sudden cardiac death
Outline
Pathophysiology, 925
Ventricular Hypertrophy, 925
Diastolic Dysfunction, 925
Left Ventricular Outflow Obstruction, 925
Myocardial Ischemia, 926
Ventricular Arrhythmias, 926
Molecular Genetics, 926
Epidemiology and Natural History, 927
Clinical Presentation, 929
Heart Failure, 929
Left Ventricular Outflow Obstruction, 929
Myocardial Ischemia, 929
Syncope, 929
Atrial Fibrillation, 929
Ventricular Arrhythmias, 929
Initial Evaluation, 930
Electrocardiography, 930
Ambulatory Cardiac Monitoring, 930
Echocardiography, 930
Exercise Testing, 930
Electrophysiological Testing, 930
Cardiac Magnetic Resonance, 930
Genetic Testing, 931
Differential Diagnosis, 932
Risk Stratification, 935
Established Risk Markers, 935
Possible Risk Modifiers, 936
Conventional Risk Stratification Model, 937
“HCM Risk-SCD” Model, 937
Principles of Management, 938
Treatment of Heart Failure and Left Ventricular Outflow Obstruction, 938
Treatment of Atrial Fibrillation, 938
Treatment of Ventricular Arrhythmias, 938
Family Screening, 940
Genotype-Positive/Phenotype-Negative Patients, 940
Pathophysiology
Hypertrophic cardiomyopathy (HCM) is characterized by a thickened but nondilated left ventricle (LV) in the absence of any other cardiac or systemic condition capable of producing the magnitude of hypertrophy evident (e.g., aortic valve stenosis, systemic hypertension, some expressions of athlete’s heart, infiltrative or storage disorders), independent of whether obstruction to the left ventricular outflow tract (LVOT) is present.
Ventricular Hypertrophy
HCM is characterized by myocyte disarray. Cardiomyocytes in HCM become hypertrophied, enlarged, and distorted, which leads to disorientation of adjacent cells and arrangement in chaotic disorganized patterns (instead of the normal parallel cellular arrangement), forming circles or whorls around foci of connective tissue. Although the disorganized architecture is evident in the majority (95%) of patients dying from HCM, it is not specific to HCM, and it occurs in other syndromic causes of LV hypertrophy such as Noonan syndrome and Friedreich ataxia, congenital heart disease, hypertension, and aortic stenosis. Nevertheless, myocyte disarray in HCM is typically more extensive, occupying more than 5% of the total myocardium (including substantial portions of hypertrophied as well as nonhypertrophied LV myocardium), 33% of the ventricular septum, and 25% of the free wall.
Changes in myocyte architecture, myocyte hypertrophy, and expanded extracellular matrix composed of interstitial and replacement fibrosis lead to ventricular hypertrophy ( Fig. 28.1 ). The degree and distribution of LV hypertrophy vary markedly. LV hypertrophy can be asymmetrical or symmetrical. Asymmetrical septal hypertrophy is the most common variant and is associated with thickening of the basal anterior septum, which bulges beneath the aortic valve and causes narrowing of the LVOT region. However, isolated segmental hypertrophy can affect the LV apex (apical HCM) or any portion of the LV. The symmetrical form accounts for more than one-third of cases and is characterized by concentric thickening of the LV with small ventricular cavity dimensions. The morphological pattern of LV hypertrophy is not closely predictive of the severity of symptoms or prognosis.

Diastolic Dysfunction
Diastolic dysfunction is a major pathophysiological abnormality in HCM. Myocardial hypertrophy, LVOT obstruction, myocardial ischemia, replacement scarring, extracellular fibrosis, and disorganized cellular architecture, as well as abnormal cellular energetics and calcium handling, all contribute to increased LV stiffness, reduced compliance, impaired relaxation, and diastolic dysfunction.
Left Ventricular Outflow Obstruction
In the majority of patients, LVOT obstruction is precipitated by prolapse of the anterior leaflet of the mitral valve into the LVOT. This is related to systolic anterior motion (SAM) of the leaflet caused by systolic flow against the abnormally positioned mitral valve apparatus, independent of the presence of septal hypertrophy. Morphological abnormalities of the mitral valve (elongation of leaflets or accessory tissue), as well as papillary muscle abnormalities (hypertrophy, displacement, direct insertion into the anterior leaflet of the mitral valve), contribute to LVOT obstruction. In addition, septal hypertrophy can cause narrowing of the LVOT and promote obstruction caused by SAM. Almost always, SAM results in failure of normal leaflet coaptation and eccentric mitral regurgitation, with the jet directed laterally and posteriorly and predominating during mid and late systole. In addition, LVOT obstruction can result in reduced forward cardiac output, impaired LV relaxation, increased LV diastolic pressure, and myocardial ischemia.
In a minority of patients, obstruction occurs at the mid LV cavity level, caused by systolic apposition of hypertrophied (abnormally positioned) papillary muscle and LV wall. This form of obstruction may be associated with LV apical aneurysm (in up to 25% or patients).
LVOT obstruction is dynamic and is typically aggravated by maneuvers that decrease LV preload or afterload or increase contractility or heart rate. By convention, LVOT obstruction is defined as an instantaneous peak Doppler LVOT pressure gradient of 30 mm Hg or more at rest or during physiological provocation such as Valsalva maneuver, standing, and exercise. A gradient of 50 mm Hg or more is usually considered to be the threshold at which LVOT obstruction becomes hemodynamically important.
Myocardial Ischemia
Severe myocardial ischemia and even infarction can occur in HCM and is frequently unrelated to atherosclerotic epicardial coronary artery disease. Microvascular ischemia can be induced by a supply-demand mismatch. Increased demand is usually related to increased muscle mass, as well as increased wall stress due to elevated diastolic pressures. Reduced supply can be precipitated by impaired vasodilator reserve, myocardial bridging and compression of intramural vessels, LVOT obstruction, abnormalities in intramural coronary arterioles, reduced capillary density relative to the myocardial mass, and microvascular dysfunction. Bursts of focal myocardial ischemia result in repeated cycles of myocyte death with consequent replacement fibrosis and occasionally extensive scarring.
Ventricular Arrhythmias
The arrhythmogenic substrate responsible for ventricular tachycardia (VT) occurrence in HCM has not been completely defined. Myofibrillar disarray, as well as diffuse interstitial myocardial fibrosis or replacement scar (likely caused by focal ischemia), which potentially predispose to disordered conduction patterns and increased dispersion of electrical depolarization and repolarization, have been suggested as factors contributing to reentry and ventricular arrhythmogenesis.
Molecular Genetics
HCM is familial in approximately half of the cases and sporadic in the other half. The disease is transmitted as an autosomal dominant trait with variable expressivity and age-related (incomplete) penetrance. Sporadic cases can be due to de novo (new) mutations present in the proband (and absent in the parents), which then initiates a new familial disease. Furthermore, apparently sporadic cases can arise because of incomplete penetrance (absence of clinical expression despite the presence of a mutation) in a parent, inaccurate family history, or, less commonly, autosomal recessive inheritance.
There is a substantial diversity in the genetic causes of HCM. To date, more than 1500 disease-causing mutations have been identified in genes that encode proteins of the thick and thin contractile myofilament components of the cardiac sarcomere or the adjacent Z-disc ( Fig. 28.2 ). These mutations have been localized to 11 sarcomere genes, 6 Z-disc genes, and 2 calcium-handling genes. Among these genes, mutations in MYH7 (encoding beta-myosin heavy chain) and MYBPC3 (encoding cardiac myosin binding protein-C) are the most common, each accounting for one-quarter to one-third of all cases ( Fig. 28.3 ). Other sarcomeric mutations (including genes encoding troponin T, troponin I, alpha-tropomyosin, actin, regulatory light chain, and essential light chain) are less common. For each gene, several different mutations have been identified. Approximately 5% of patients with HCM have two or more mutations in the same gene or different genes. The vast majority of those mutations are missense (whereby a single normal amino acid is replaced for another). Frameshift mutations (insertion or deletion of one or more nucleic acids) are less common.


HCM is a complex and clinically heterogeneous disease that demonstrates remarkable diversity in disease course, age of onset, severity of symptoms, LVOT obstruction, and risk for sudden cardiac death (SCD). The characteristic diversity of the HCM phenotype is attributable to the intergenetic heterogeneity (with a variety of mutations encoding protein components of the cardiac sarcomere) and the intragenetic heterogeneity (with multiple different mutations identified in each gene), as well as the potential influence of modifier genes and environmental factors. Of note, no definitive genotype-phenotype relationships have been established between individual sarcomere mutations and the type or extent of morphological expression in HCM. The clinical outcome may not be predicted based on individual mutations, and the extent and distribution of LV hypertrophy can be dramatically different among relatives with the same disease-causing sarcomere mutation.
Epidemiology and Natural History
HCM is the most common genetic cardiovascular disease, with a prevalence of approximately 0.2% of the general population for the disease phenotype (i.e., LV hypertrophy recognized by echocardiography). Some investigators suggested that the combined prevalence of clinically expressed HCM and gene carriers (at risk for developing the disease phenotype) is likely higher and estimated that up to 0.6% of the population may carry disease-causing sarcomere mutations. However, this estimate has been debated.
The major causes of death in HCM are SCD, heart failure, and stroke. SCD accounts for approximately half of total mortality in patients with HCM. Progressive heart failure is responsible for the death of one-third of patients, usually after middle age (more than 55 years), and atrial fibrillation (AF)-associated stroke is responsible for the death of 13% of the patients at a higher age, usually older than 65 years.
Approximately 10% to 20% of individuals with HCM have a lifetime-increased risk for SCD, most likely resulting from VT and ventricular fibrillation (VF). SCD can be the first manifestation of the disease. HCM is the most common cause of SCD in the young and is the leading single cause of SCD in competitive athletes in the United States, accounting for approximately one-third of athletic field deaths in high school and college students engaged in organized sports. Although SCD occurs most often in adolescents or adults younger than 35 years, it can occur at any age. However, SCD is uncommon in young children and very uncommon in patients older than 60 years, even among those with established risk factors.
Individuals with HCM who are at the highest risk can have a greater than 3% to 5% annual rate of SCD. However, studies on broader-based populations suggest that the prognosis is typically more favorable and most affected individuals probably achieve a normal life expectancy with little functional disability. Individuals in the general HCM population have 0.5% to 1% annual risk of SCD and 1.4% annual total cardiovascular mortality.
Compared with patients with LVOT obstruction, HCM patients without the capacity to physiologically generate LVOT obstruction appear to have a more favorable prognosis, with lower risk for developing most HCM-related complications, including progressive heart failure, SCD, or embolic stroke, and with an HCM-related mortality rate of 0.5% per year.
Approximately 2% to 9% of HCM patients progress to end-stage (or “burned-out”) phase of the disease, marked by LV systolic dysfunction (ejection fraction less than 50%) and occasionally progressive LV dilatation and wall thinning. Progression to end-stage disease is likely related to extensive myocardial scarring, and it can occur at a young age (approximately 50% of the patients are less than 40 years of age). The only known predictor of end-stage HCM is a family history of end-stage disease. The transition to end-stage HCM carries an ominous prognosis; over a 3-year interval, approximately two-thirds of these patients die secondary to severe heart failure or SCD or require cardiac transplantation. Posttransplantation survival is favorable (75% at 5 years; 60% at 10 years).
Contemporary medical and invasive management strategies, including implantable cardioverter-defibrillator (ICD) implantation and septal reduction procedures, as well as heart transplantation, have significantly altered the natural history of HCM, currently resulting in an annual disease-related total mortality rate of only 0.5%.
Men are overrepresented in published HCM patient cohorts, suggesting a higher penetrance of HCM-causing mutations in male patients. Moreover, at the time of diagnosis, women were on average 9 years older than men. In addition, the extent of myocardial fibrosis is higher in male patients; nonetheless, diagnosed women tend to be more symptomatic, more likely to have LVOT obstruction, and fare worse than men. There appears to be no gender impact on the risk of SCD or the incidence of atrial or ventricular arrhythmias in patients with HCM.
Clinical Presentation
The clinical presentation of HCM is characterized by extreme variability in disease course, age of onset, severity of symptoms, and risk for SCD. Many patients are either asymptomatic or mildly symptomatic, and the diagnosis is frequently made as a result of family screening, detection of a murmur during routine examination, or the identification of an abnormal electrocardiogram (ECG). The majority of patients present during adolescence or young adulthood, but symptoms can develop at any age. Symptoms can be related to LV diastolic dysfunction, LVOT obstruction, myocardial ischemia, cardiac arrhythmias, or a combination of those mechanisms. Nonetheless, there is no predictable correlation between clinical symptoms and the presence or severity of LVOT obstruction or the extent of LV hypertrophy. The extent of LV hypertrophy does not typically change once early adulthood is reached.
Heart Failure
Variable degrees of heart failure occur in more than 50% of HCM patients. Shortness of breath, particularly exertional, is the most common symptom of HCM. The most common mechanism of heart failure is LVOT obstruction, but diastolic LV dysfunction can precipitate heart failure symptoms even in the absence of LVOT obstruction. Although left ventricular ejection fraction (LVEF) is typically preserved or even hyperdynamic, a minority of patients can progress to the end-stage phase of HCM, marked by LV systolic dysfunction and occasionally progressive LV dilatation and wall thinning. These patients can develop advanced heart failure symptoms of pulmonary and systemic venous congestion (orthopnea, paroxysmal nocturnal dyspnea, edema), which can become refractory to medical therapy and require cardiac transplantation.
Left Ventricular Outflow Obstruction
Approximately 20% to 30% of individuals with HCM demonstrate LVOT obstruction at rest, and a similar proportion exhibit LVOT obstruction only in response to maneuvers that decrease LV preload or afterload or increase contractility or heart rate (“dynamic” LVOT obstruction). LVOT obstruction is the most common cause of heart failure symptoms (dyspnea) in HCM patients but can also precipitate chest pain and syncope. LVOT gradients and related symptoms can be aggravated by exercise, dehydration, low blood volume, large meals, excess alcohol intake, and certain drugs (such as vasodilators, diuretics, digoxin). On physical examination, LVOT obstruction can manifest as a dynamic ejection systolic murmur (with increasing intensity in response to Valsalva maneuver, during or immediately after exercise, or on standing), mitral regurgitation murmur, and an arterial pulse with bisferiens contour. Squatting reduces the LVOT gradient and intensity of the systolic murmur.
Myocardial Ischemia
Exertional chest discomfort occurs in 25% to 30% of patients with HCM, usually in the setting of a normal coronary arteriogram, and likely is related to microvascular angina caused by supply/demand mismatch. Some patients also experience atypical chest pain, frequently precipitated or worsened by heavy meals. A subset of patients can also have obstructive epicardial coronary artery disease, which heralds an adverse outcome.
Syncope
Syncope occurs in 15% to 25% of patients with HCM. Another 20% complain of presyncope. Different mechanisms can precipitate syncope in HCM patients, including arrhythmias (VT or AF), neurocardiogenic syncope, and hypotension during exercise caused by LVOT obstruction or by abnormal vascular responses. Changes in LV loading conditions during exercise, heavy meals, and dehydration often provoke symptoms.
Syncope typically occurs in younger patients with small LV end-diastolic volume. Syncope during exertion is more common in patients with LVOT obstruction than in patients without obstruction, whereas unexplained syncope at rest and neurally mediated syncope do not appear to be related to LVOT obstruction.
Neurocardiogenic syncope is characterized by classic prodromal symptoms, including lightheadedness, warmth, nausea, and tunnel vision. Individuals tend to slump to the ground rather than drop suddenly. Syncope is brief, and patients awake nauseous and exhausted. On the other hand, syncope during exertion, or immediately following palpitation or chest pain, suggests a cardiac mechanism. Ventricular arrhythmias are an uncommon cause of syncope but should be suspected when loss of consciousness occurs suddenly, without a prodrome, particularly when it occurs at rest or on minimal effort. Occasionally, paroxysmal atrial arrhythmias with rapid ventricular rates can precipitate syncope, particularly in patients with preserved atrial function and high filling pressures.
Atrial Fibrillation
AF is the most common arrhythmia observed in HCM, with an annual incidence of approximately 1% to 2% and a prevalence of approximately 20% to 25%. The incidence of AF increases with age, occurring most frequently after the age of 55 years (but approximately 10 years earlier than in the general population), and is very uncommon in patients younger than 25 years. The high incidence of AF in HCM is likely related to increased left atrium (LA) atrial pressure and size, caused by LV diastolic dysfunction. In fact, LA size is one of the most important determinants of AF occurrence. In addition, the extent of LV myocardial fibrosis (as determined by cardiac magnetic resonance [CMR]) and interatrial conduction delay (as reflected by longer P wave duration) seem to correlate with higher incidence of AF. Furthermore, certain HCM mutations appear to predispose to AF, possibly by causing intrinsic atrial myopathy. Notably, the presence or severity of LVOT obstruction does not appear to be associated with an increased incidence of AF.
Due to the underlying ventricular hypertrophy and impaired relaxation, rapid ventricular rates and loss of atrial contribution to ventricular filling during AF are usually poorly tolerated. In fact, paroxysmal episodes of AF can precipitate acute clinical deterioration, resulting in syncope or acute heart failure, particularly in patients with severe diastolic dysfunction and LVOT obstruction. In addition, AF in HCM is associated with increased risk of stroke and thromboembolic complications (eightfold increase in risk compared with HCM patients without AF), with a prevalence and annual incidence of 27.1% and 3.8%, respectively. AF is associated with a fourfold increase in HCM-related mortality, predominantly driven by the higher occurrence of heart failure and stroke-related death. Of note, AF does not appear to be associated with an increased risk of SCD.
Ventricular Arrhythmias
The predominant arrhythmia syndrome associated with HCM is sudden cardiac arrest, presumably due to polymorphic VT or VF. SCD occurs with an annual rate of approximately 6% in referral-based populations and 1% in community-based studies. However, certain patient subgroups can have much higher rates, surpassing the American College of Cardiology/American Heart Association (ACC/AHA) guideline document definition of high risk for SCD (≥2% annual risk).
HCM is the most common cause of SCD in young people, including competitive athletes, in the United States. SCD occurs throughout life, with a peak in adolescence and young adulthood (less than 35 years of age), and can be the initial disease presentation. Although SCD occurs most commonly during mild exertion or sedentary activities, an important proportion of such events is associated with vigorous exertion. In HCM patients with ICDs, more than half of the ventricular arrhythmias identified and appropriate ICD therapies were associated with moderate or competitive physical activity.
Ambulatory cardiac monitoring frequently reveals premature ventricular complexes (PVCs) (in more than 80% of patients) and nonsustained VT (25% to 30%). Nonsustained VT is associated with severity of LV hypertrophy and symptom class. Stable sustained monomorphic VT is rare but has been observed particularly in patients with LV apical aneurysms. Among ICD recipients for primary or secondary prevention, appropriate ICD therapies in response to ventricular arrhythmias occur in approximately 5.5% of HCM patients per year.
Initial Evaluation
The initial evaluation is aimed at establishing the diagnosis of HCM, excluding other potential causes of LV hypertrophy, assessing the extent of the disease, and evaluating the underlying mechanisms of symptoms (e.g., chest pain, syncope), as well as risk stratification for SCD.
Clinically, HCM in adults is defined by a wall thickness ≥15 mm in one or more LV myocardial segments (as measured by echocardiograph or CMR), in the absence of an identifiable hemodynamic cause (such as coronary artery disease, valvular heart disease, hypertension, or congenital heart disease) capable of producing the observed myocardial abnormality ( Fig. 28.4 ). With borderline wall thickness (13 to 14 mm), the diagnosis of HCM requires evaluation of other features including family history, noncardiac symptoms and signs, ECG abnormalities, laboratory tests, and multimodality cardiac imaging.

It is important to understand that any degree of wall thickness is compatible with the presence of the HCM genetic substrate. Because of the age-dependent and incomplete penetrance, many mutation carriers may not exhibit phenotypic expression (i.e., LV hypertrophy). These individuals are usually referred to as being “genotype positive/phenotype negative” or as having “subclinical HCM.”
Electrocardiography
The ECG is abnormal in more than 90% of patients with HCM and in 75% of asymptomatic relatives. An abnormal ECG in the young is a sensitive—although nonspecific—marker of early disease expression.
The surface ECG in HCM patients can show a wide variety of abnormal patterns; however, ECG abnormalities do not correlate with severity or pattern of LV hypertrophy, and no particular ECG pattern is characteristic or predictive of future events. LA enlargement, repolarization abnormalities (ST-T changes including marked T wave inversion), and deep and narrow Q waves (mimicking myocardial infarction, most commonly in the inferolateral leads) are the most frequent ECG findings and may precede manifest evidence of LV hypertrophy ( Fig. 28.5 ). Voltage criteria for LV hypertrophy alone are nonspecific and are often seen in normal young adults. Giant negative T waves in the midprecordial leads are characteristic of hypertrophy confined to the LV apex.

Ambulatory Cardiac Monitoring
Ambulatory 24-hour (Holter) cardiac monitoring is recommended as part of the initial evaluation for asymptomatic and symptomatic HCM patients because the detection of ventricular tachyarrhythmias is important for risk stratification. PVCs occur during ambulatory ECG monitoring in the vast majority of patients, nonsustained VT in 25% to 30%, and paroxysmal supraventricular arrhythmias in 38%. It is reasonable to perform serial 24-hour Holter monitoring every 1 to 2 years in patients who are stable and had no VT on previous evaluation. Reevaluation with ambulatory cardiac monitoring is also recommended to evaluate patients with palpitations, lightheadedness, or syncope.
Echocardiography
Echocardiography is central to the diagnosis of HCM (see Fig. 28.4 ). Comprehensive transthoracic echocardiography should be performed in the initial evaluation of all patients with suspected HCM, as well as during follow-up, particularly when there is a change in the clinical status or symptoms. Echocardiography can help assess the nature, distribution, and extent of hypertrophy, systolic and diastolic function, LVOT obstruction, and identify apical aneurysms.
Exercise Testing
Exercise testing can help assess blood pressure responses to exercise. In addition, combining exercise testing with Doppler echocardiography is useful for determining the presence of dynamic LVOT obstruction. Metabolic stress testing (i.e., determination of maximum oxygen consumption) may be considered when a more precise assessment of functional capacity is clinically required.
Electrophysiological Testing
Invasive electrophysiological (EP) testing is indicated in patients with documented or suspected supraventricular or ventricular arrhythmias when catheter ablation is being considered. However, programmed ventricular stimulation to evaluate inducibility of ventricular arrhythmias has little predictive value for SCD in HCM and is not recommended for SCD risk stratification. Similarly, the routine use of EP study for evaluation of patients with syncope is not recommended.
Cardiac Magnetic Resonance
CMR should be considered when the echocardiogram is inconclusive or suboptimal. CMR also can provide valuable additional information in the diagnosis of HCM, including more precise LV wall thickness measurements, defining extent and location of myocardial scars, and identification of regional wall hypertrophy and LV apical aneurysms. In addition, CMR can help differentiate apical HCM from LV noncompaction. Furthermore, late gadolinium enhancement on contrast CMR can help to define the presence, severity, and distribution of myocardial fibrosis ( Fig. 28.6 ). Late gadolinium enhancement is present in 65% of HCM patients, typically in a patchy mid-wall pattern in areas of hypertrophy. More extensive late gadolinium enhancement can be associated with more severe disease and worse prognosis.

Genetic Testing
Given the lack of strong evidence of specific genotype-phenotype associations, the knowledge of the underlying gene and mutation has a limited role in risk stratification and management of the individual patient. Nonetheless, genetic testing is recommended for patients with established clinical diagnosis of HCM in whom mutation-specific confirmatory testing would help to confirm or exclude the diagnosis in at-risk family members. Similarly, postmortem genetic analysis should be considered in deceased patients with pathologically confirmed HCM, to enable cascade genetic screening of their relatives.
Genetic analysis should include the most commonly implicated sarcomere protein genes. Overall, the yield of genetic testing in probands with HCM is approximately 60% because some genes causing HCM have not yet been identified and are absent from testing panels. The likelihood of finding a causal mutation depends on patient selection, being highest in patients with familial disease and lowest in older patients and individuals with nonclassical features.
Genetic testing is not recommended as a diagnostic test of HCM in patients with nondiagnostic clinical features (e.g., athletes and those with hypertension) because the absence of a sarcomere mutation cannot rule out familial HCM. Furthermore, finding variants of uncertain significance are difficult to interpret.
Genetic counseling is recommended for all patients with HCM, to explain the potential risks and benefits and enhance understanding of the medical and familial implications of test results. Even when genetic testing is not undertaken, genetic counseling about the potential for familial transmission of HCM is medically important.
Differential Diagnosis
Phenocopies of Hypertrophic Cardiomyopathy
LV hypertrophy is a relatively nonspecific phenotype that may reflect the final common pathway for a number of different disease processes. It does not indicate etiology or reveal underlying pathophysiology. In infants, older children, and young adults, LV hypertrophy mimicking sarcomeric HCM is often associated with congenital malformations and syndromes (e.g., Noonan, LEOPARD, and Costello syndromes), inherited metabolic storage disorders (e.g., Anderson-Fabry disease, Danon disease, PRKAG2 syndrome, Pompe disease, Forbes disease), mitochondrial cytopathies (e.g., MELAS, MERRF, LHON), and neuromuscular diseases (e.g., Friedreich ataxia). These clinical entities are distinct from HCM caused by sarcomere protein mutations, despite the shared feature of LV hypertrophy ( Table 28.1 ).
Associated Phenotype | Gene | Protein | Inheritance | Frequency in Patients With HCM Phenocopy Diseases | Common Clinical Features | Diagnosis | Treatment |
---|---|---|---|---|---|---|---|
PRKAG2 syndrome | PRKAG2 | AMP-activated protein kinase γ2 regulatory subunit |
| <1% |
|
|
|
Anderson-Fabry disease | GLA | Alpha-galactosidase A |
| <5% |
|
|
|
Pompe disease (glycogen storage disease type IIa, acid maltase deficiency) | GAA | Acid alpha-1,4-glucosidase |
| Rare |
|
|
|
Danon disease | LAMP2 | Lysosomal-associated membrane protein 2 |
| Rare |
|
|
|
Mitochondrial cytopathies (MELAS, MERRF, LHON) | Various mitochondrial genes (e.g., MTTG, MTTI ) | Protein-encoding mitochondrial ribosomal and transfer RNA (respiratory chain protein complexes) |
| Rare |
|
|
|
Noonan syndrome |
| RAS/RAF/MEK/ERK signal transduction pathway |
| Rare |
|
|
|
Friedreich ataxia | FXN | Frataxin |
| Rare |
|
|
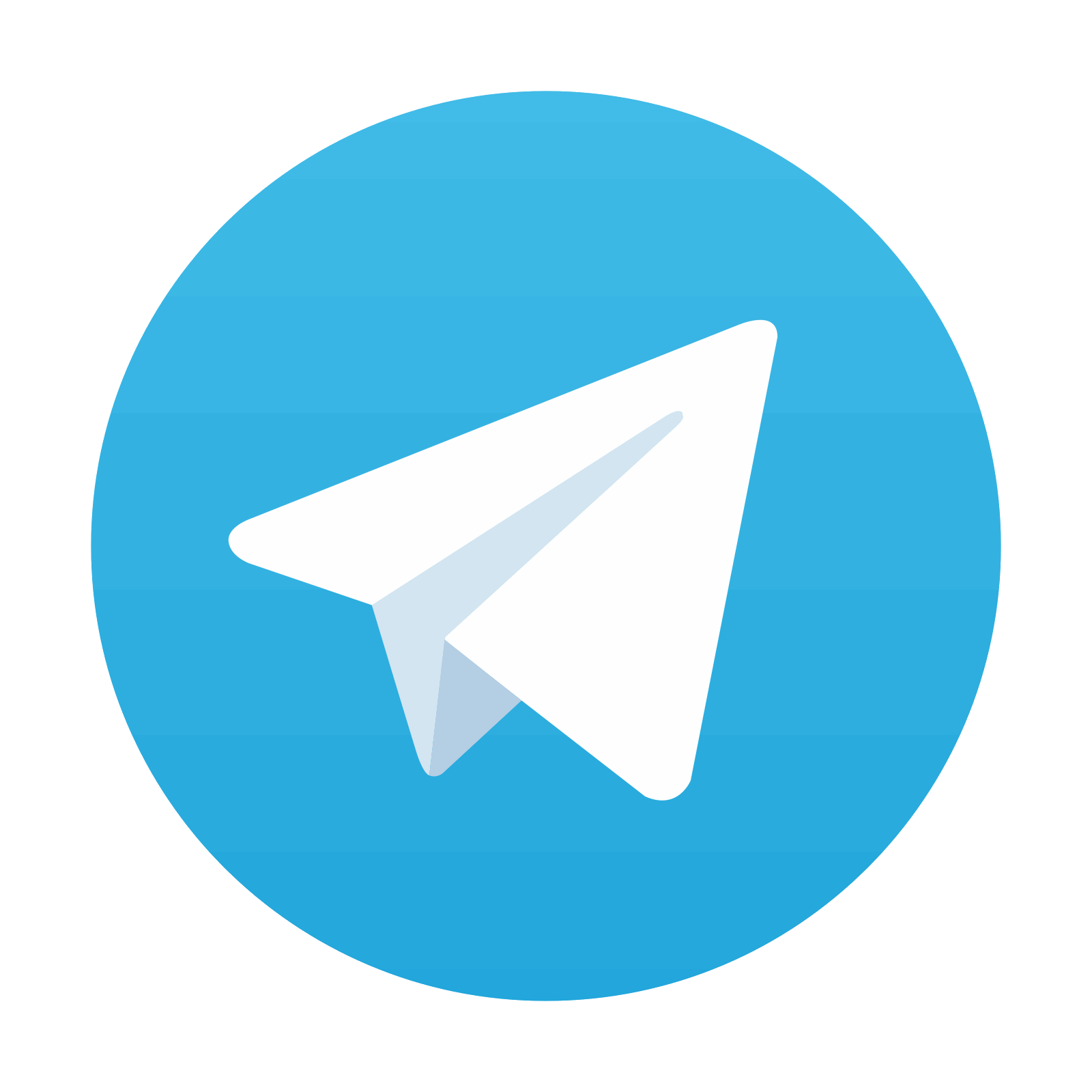
Stay updated, free articles. Join our Telegram channel

Full access? Get Clinical Tree
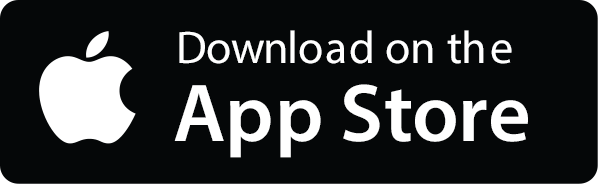
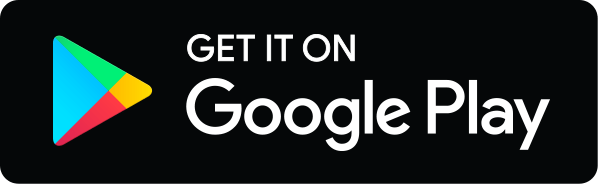
