Fig. 19.1
(a) ECG exhibiting polymorphous VT that was determined to be torsades de pointes, (b) ECG showing sustained scar-related monomorphic VT. ECG electrocardiogram, VT ventricular tachycardia
Apart from acute ischemia, ventricular tachyarrhythmias may also occur as a result of structural heart disease that causes localized disturbances of electrical activation in the myocardium or conduction system. In severe disease, left ventricular function may be markedly impaired resulting in an ischemic cardiomyopathy.
The most common structural disturbance leading to tachyarrhythmia susceptibility is scar remaining after a prior myocardial infarction due to obstructive coronary artery disease. However, ventricular scars leading to reentrant VT also occur in nonischemic cardiomyopathies, including idiopathic dilated cardiomyopathy, hypertrophic cardiomyopathy, infiltrative heart disease (e.g., sarcoidosis), right ventricular dysplasia (also now known as right ventricular cardiomyopathy), and postrepair of congenital heart disease or valvular heart disease.
Reentry involving the regions of myocardial scar noted above is the basis for most instances of sustained monomorphic VT in patients with structural heart disease. In such cases, the scar zone contains viable fibers that provide for the slow conduction that is a necessary requirement for sustained reentry. These slow conduction zones and sometimes the coexisting conduction pathways sustaining the arrhythmia can be identified by “electro-anatomic mapping” in the electrophysiology laboratory and subsequently modified by radiofrequency ablation to diminish VT susceptibility.
Bundle branch reentry is an important but less common form of reentry mostly seen in nonischemic dilated cardiomyopathy, but can be occasionally seen in ischemic cardiomyopathy. Typically, bundle branch reentry (◘ Fig. 19.2) occurs in the setting of severe cardiomyopathy in which there is usually a combination of both conduction system disease and marked ventricular dilatation. The reentry in these cases uses the bundle branches. Most often anterograde ventricular activation occurs over the right bundle branch, with retrograde conduction back over the usually diseased left-sided conduction system. The conduction system disease in conjunction with a dilated ventricle allows for the necessary electrical circuit size that permits sustained reentry. Recognition of this arrhythmia is important because it is amenable to catheter ablation therapy by transecting the bundle branches (usually the right bundle as it is a more discrete target) [38, 39].


Fig. 19.2
ECG showing bundle branch reentry VT in a patient with conduction system disease. VT ventricular tachycardia
Management of VT includes antiarrhythmic therapy and ablation therapy . In the era of defibrillators, VT manifests the most with ICD shocks. Patients who present with an ICD shock should be first evaluated to rule out obvious reversible causes: electrolyte abnormalities, device interrogation to make sure it is an appropriate shock, decomposition of heart failure, ischemic workup especially in patients with ischemic cardiomyopathy, and polymorphic VT. It should be mentioned that ischemia can manifest with monomorphic VT, challenging the old dogma just mentioned. Moreover, beta-blocker therapy needs to be optimized. Once a reversible cause is ruled out and/or treated, medical antiarrhythmic therapy is usually the first-line therapy and needs to be tailored to the type of cardiomyopathy. Antiarrhythmic therapy is detailed in a separate section. Of note, if a patient presents with a single ICD shock with no recurrence, it is reasonable to defer therapy until a significant recurrence since VT burden tends to sometimes wax and wane without intervention.
Ablation therapy for VT related to cardiomyopathy is slowly gaining momentum and is currently considered in patients with recurrent VT resistant to antiarrhythmic medical therapy [40]. Although the occurrence of VT increases mortality in patients with structural heart disease, there is still debate whether VT suppression, especially with VT ablation, affects mortality.
There are two main approaches to VT ablation with ramifications of each. The first is based on activation mapping, which includes inducing the clinical VT and using 3D and conventional mapping techniques to define the circuit and ablate the area responsible for sustaining the tachycardia. This is limited by the fact that only about 10 % of the VTs are hemodynamically stable enough to have time for mapping while in VT. The percentage of VTs amenable to this approach increased with the advent of hemodynamic support such as an intra-aortic balloon pump, the CARDIOHELP system (Maquet Cardiopulmonary AG, Hirrlingen, Germany), and IMPELLA (Abiomed, Danvers, MA). This method is limited by the potential vascular complications with hemodynamic support and the potential of stunning the myocardium with subsequent severe decompensation of heart failure from prolonged times in VT during the ablation. The second method consists of substrate modification, with the advantage of performing ablation in sinus rhythm without prolonged time in VT. The goal is to homogenize the scarred area in order to eliminate potential slow conduction channels responsible for sustaining VT. There are a multitude of approaches within substrate modification, which is out of the scope of this chapter. Overall, most operators use a hybrid approach between the two methods that permits targeting of the clinical VT and prevents future potential circuits.
We will review the efficacy of VT ablation with each of the most common clinical scenarios.
Ablation Therapy for VT in Ischemic Cardiomyopathy (ICM)
Two main relatively large prospective randomized clinical trials examine the outcomes after VT ablation in patients with ICM [41, 42].
SMASH-VT included 128 patients with recently implanted ICDs for secondary prevention and with primary prevention ICDs who had received a single appropriate therapy [41]. Freedom from recurrent VT/VF resulting in appropriate ICD therapy after 2 years of follow-up was significantly higher in the ablation arm (88 % versus 67 %; HR 0.35; 95 % CI 0.15–0.78; P = 0.007) compared with controls.
The second landmark trial was the Ventricular Tachycardia Ablation in Coronary Heart Disease (VTACH) study that enrolled 110 patients with hemodynamically stable VT, prior MI, and reduced left ventricular ejection fraction (LVEF), who were randomly assigned to catheter ablation and ICD versus ICD alone [42]. After 2 years, the ablation arm had less VT/VF (47 % versus 29 %; HR 0.61; 95 % CI 0.37–0.99; P = 0.045) and fewer appropriate ICD shocks per patient year (mean 0.6 ± 2.1 versus 3.4 ± 9.2 shocks; P = 0.018).
There are a multitude of retrospective studies with various success rates and effects on mortality. The main limitation is the largely heterogeneous ablation techniques adopted. There are two ongoing VT ablation studies for ICM patients, the VANISH and BERLIN trials, that will also study mortality [43, 44]. Two other trials, STAR-VT and PARTITA, will include both ICM and NICM patients [45, 46].
Ablation Therapy for VT in Nonischemic Cardiomyopathy (NICM)
Unlike ICM, where the underlying substrate is relatively well defined based on the affected coronary artery, patients with NICM have heterogeneous substrates that include different degrees of involvement of the mid-myocardium and epicardium, usually in the perivalvular regions.
To date, there are no prospective randomized trials describing outcomes of VT ablation in patients with NICM. In general, patients with NICM have higher rates of acute procedural failure and long-term VT recurrence after ablation therapy compared to patients with ICM. The HELP-VT study was a prospective observational European single-center study that enrolled 63 patients with NICM and 164 patients with ICM treated with VT ablation [47]. VT-free survival rates at 1-year follow-up were 40.5 % for NICM and 57 % for ICM. One large single-center retrospective observational study included 226 patients with NICM treated with VT ablation. The composite endpoint of death, heart transplantation, or hospitalization for VT recurrence at 1 year (after the last ablation) was 31 % [48].
Role of the Implantable Cardioverter Defibrillator
Patients with either ischemic or nonischemic cardiomyopathy have an increased risk of SCD due to ventricular tachyarrhythmias. SCD is also the leading cause of mortality in patients with heart failure (HF) and occurs at a rate six to nine times that seen in the general population.
The Role of Implantable Cardioverter Defibrillator (ICD) Therapy in Cardiomyopathy
In general, a systolic dysfunction worsens (i.e., the left ventricular [LV] ejection fraction becomes lower), and the severity of HF becomes more marked. In LV dysfunction of NYHA Class I and II and moderate III severity, SCD is most often due to VF, and ICD therapy has proved highly effective. However, as LV function deteriorates further (severe NYHA Class III and Class IV), the propensity for bradyarrhythmic deaths increases (particularly pulseless electrical activity). Inasmuch as the most prominent bradyarrhythmias in this setting are associated with pulseless electrical activity (PEA), they are not amenable to ICD therapy. Prevention of the latter scenario requires that both medical therapy to slow deterioration of LV function and ICD therapy to terminate VF events be employed in concert at an early stage of patient care.
Given the worrisome susceptibility to SCD in patients with diminished LV ejection fraction (particularly <35 %) and heart failure, the ICD has emerged as an important lifesaving treatment option. Randomized trials have consistently shown that ICD implantation reduces mortality in HF patients with reduced left ventricular function, as well as in patients who have suffered a cardiac arrest [49–51] (◘ Tables 19.1 and 19.2). Further, ICD therapy has always proved superior to antiarrhythmic drug therapy. Two broad categories of patients are candidates for ICD therapy: (a) secondary SCD prevention and (b) primary SCD prevention.
Table 19.1
Major randomized clinical ICD trials (secondary prevention)
Study | Year | Inclusion criteria | Patients, n | ICD, n | Mean follow-up (month) | Main result |
---|---|---|---|---|---|---|
AVID [5] | 1997 | Any of (1) VF, (2) VT with syncope, or (3) VT with severe symptoms and EF ≤ 40 % | 1016 | 507 | 18 | ICD therapy resulted in 31 % RR reduction in mortality (CI 10–52 %), P = 0.002 |
CASH [7] | 2000 | Cardiac arrest secondary to ventricular arrhythmia | 288 | 99 | 57 | ICD therapy resulted in nonsignificant 23 % RR reduction in mortality (CI lower bound–11 %), P = 0.08 |
CIDS [8] | 2000 | Any of (1) VF, (2) out-of-hospital cardiac arrest requiring defibrillation or cardioversion, (3) VT with syncope, (4) VT ≥ 150 bpm with symptoms and EF ≤ 35 %, or (5) unmonitored syncope with subsequent VT | 659 | 328 | 36 | ICD therapy resulted in nonsignificant 20 % RR reduction in mortality (CI—8–40 %) P = 0.142 |
Table 19.2
Major randomized clinical ICD trials
Primary prevention in ischemic cardiomyopathy | ||||||
Study | Year | Inclusion criteria | Patients, n | ICD, n | Follow-up (mo) | Main result |
MADIT [10] | 1996 | EF ≤ 35 %, MI ≥ 3 weeks before entry, NSVT, inducible sustained VT on EPS, NYHA I–III | 196 | 95 | 27 | ICD therapy resulted in 54 % RR reduction in mortality (CI 18–74 %), P = 0.009 |
CABG [12] Patch | 1997 | EF ≤ 35 %, abnormal SAECG, epicardial ICD during CABG | 900 | 446 | 32 | ICD therapy did not reduce mortality, P = 0.64 |
MUSTT [11] | 1999 | EF ≤ 40 %, MI 1 month before entry, asymptomatic NSVT | 704 | 161 | 39 (median) | ICD therapy resulted in 55 % RR reduction in mortality (CI 37–68 %), P = 0.001 |
MADIT-II [4] | 2002 | EF ≤ 30 %, MI 1 month before entry, NYHA I–III | 1232 | 742 | 20 | ICD therapy resulted in 31 % RR reduction in mortality (CI 7–49 %), P = 0.016 |
SCD-HeFT [3] | 2005 | EF ≤ 35 %, 3 months of optimal medical therapy, NYHA II–III | 2521 total, 1310 ischemic | 829 total, 431 ischemic | 45.5 (median) | Overall, ICD therapy resulted in 23 % RR reduction in mortality (CI 4–38 %), P = 0.007; in ischemic patients, ICD therapy resulted in nonsignificant 21 % RR reduction in mortality (CI—4–40 %), P = 0.05. No evidence of effect modification by etiology |
Primary prevention in ischemic cardiomyopathy: early after MI | ||||||
DINAMIT [16] | 2004 | EF ≤ 35 %, within 6–40 days of MI, depressed HRV, or average Holter HR ≥ 80 bpm, NYHA I–III | 674 | 332 | 33 | ICD group had a significant decrease in risk of death due to arrhythmia, P = 0.009 but a significant increase in risk of non-arrhythmic death, P = 0.02. ICD therapy did not reduce all-cause mortality, P = 0.66 |
IRIS [17] | 2009 | EF ≤ 40 %, within 5–31, HR—90 bpm or NSVT ≥ 150 bpm, NYHA I–III | 898 | 445 | 37 | ICD group had a significant decrease in sudden cardiac death, P = 0.049 but a significant increase in risk of non-sudden cardiac death, P = 0.001. ICD therapy did not reduce mortality, P = 0.78 |
Primary prevention in nonischemic cardiomyopathy | ||||||
CAT [13] | 2002 | EF ≤ 30 %, new-onset DCM, NYHA II–III | 104 | 50 | 66 | ICD therapy did not reduce mortality, P = 0.55 |
AMIOVIRT [14] | 2003 | EF ≤ 35 %, DCM, asymptomatic NSVT, NYHA I–III | 103 | 51 | 36 | ICD therapy did not reduce mortality, P = 0.80 |
DEFINITE [15] | 2004 | EF ≤ 35 %, NSVT, NYHA I–III | 458 | 229 | 29 | ICD therapy resulted in nonsignificant 35 % RR reduction in mortality (CI—6–60 %), P = 0.08 |
SCD-HeFT [3] | 2005 | EF ≤ 35 %, 3 months optimal medical therapy, NYHA II–III | 2521 total, 1310 ischemic | 829 total, 431 ischemic | 45.5 (median) | Overall, ICD therapy resulted in 23 % RR reduction in mortality (CI 4–38 %), P = 0.007; in ischemic patients, ICD therapy resulted in nonsignificant 21 % RR reduction in mortality (CI—4–40 %), P = 0.05. No evidence of effect modification by etiology |
Secondary SCD Prevention
Secondary prevention refers to the prevention of SCD in patients who have survived a prior cardiac arrest or sustained VT. If the initial arrhythmic event was not due to a clearly reversible or temporary cause (such as an electrolyte disturbance, a transient hypoxia due to respiratory failure, or an acute coronary ischemia episode that can be addressed), then there is a high risk (>40 %) of experiencing a recurrent episode of VT or VF in the next 2 years [52]. In such cases, several clinical trials have shown that ICD use results in improved survival compared with antiarrhythmic agents. By way of summarizing these observations, a meta-analysis of secondary prevention trials (AVID [Antiarrhythmics Versus Implantable Defibrillators], CASH [Cardiac Arrest Study Hamburg], and CIDS [Canadian Implantable Defibrillator Study] [51, 53, 54]) demonstrated that ICD use was associated with a 50 % relative risk reduction for arrhythmic death and a 25 % relative risk reduction for all-cause mortality [55] (◘ Table 19.1).
Primary SCD Prevention
Primary SCD prevention refers to the use of ICDs in individuals who are at risk for, but have not yet experienced, an episode of sustained VT, VF, or resuscitated cardiac arrest. Early primary prevention trials focused on patients with ischemic cardiomyopathy (MADIT–I [Multicenter Automatic Defibrillator Implantation Trial], MUSTT [Multicenter Unsustained Tachycardia Trial], MADIT–II [Multicenter Automatic Defibrillator Implantation Trial-II], CABG–Patch [Coronary Artery Bypass Graft Patch Trial]) [49, 56–58]. These prospective, randomized, multicenter studies showed benefit of ICD therapy for primary SCD prevention and improved total survival in patients with ischemic cardiomyopathy (◘ Table 19.2). Initial trials of ICD therapy for primary prevention in patients with nonischemic cardiomyopathy (CAT, the Cardiomyopathy Trial) and AMIOVIRT (amiodarone versus implantable cardioverter defibrillator) showed no survival benefit, but were limited by small sample size [59, 60]. However, subsequent larger trials (DEFINITE, Defibrillators in Non-Ischemic Cardiomyopathy Treatment Evaluation, and SCD–HeFT, Sudden Cardiac Death in Heart Failure Trial) have extended the evidence of ICD benefit to patients with nonischemic cardiomyopathy and have demonstrated decreased mortality from prophylactic ICD implantation in this patient group [50, 61] (◘ Table 19.2).
Given the high risk of SCD in the early post-myocardial infarction (MI) period (e.g., in the Valsartan in Acute Myocardial Infarction Trial [VALIANT], the risk of sudden death was highest in the first 30 days after an MI) and the benefits of ICD therapy in patients with cardiac dysfunction due to MI, the consideration arose that ICD implantation would be beneficial early after MI [52]. However, two separate randomized trials have failed to show the benefit of ICD implantation within 30–40 days after MI (DINAMIT, IRIS) [62, 63] (◘ Table 19.2). Subsequent analysis of VALIANT and DINAMIT has demonstrated a possible pathophysiologic mechanism for the absence of benefit of ICD implantation in the early period after MI [52, 62]. In DINAMIT, only 50 % of the sudden deaths were attributable to arrhythmia, whereas mechanical causes of SCD (e.g., LV rupture, acute mitral regurgitation) were common in the other half of patients. Similarly in VALIANT, in the first month after MI, 80 % of sudden cardiac deaths appeared to be due to recurrent MI or myocardial rupture, and presumed arrhythmia-induced SCD only accounted for 20 %. By 1 year, the proportions of sudden deaths due to non-arrhythmia versus arrhythmia causes were equal, and over time there appeared to be a very gradual increase in the proportion of sudden deaths due to arrhythmia (approximately 60 % at 30 months). Therefore, early implantation of an ICD in this patient population would not be expected to significantly impact deaths. These observations have led to specific recommendations regarding “waiting periods” between the occurrence of an acute event and the placement of an ICD. In fact, after these studies, CMS (Center for Medicare & Medicaid Services) ruled that there should be at least 40 days of waiting period after MI before ICD implantation. In addition, due to the possibility of EF improvement in patients who underwent revascularization or in whom there were reversible causes of NICM (myocarditis, postpartum cardiomyopathy, etc.), CMS requirements demand at least 90 days waiting period after revascularization and/or newly diagnosed and medically treated NICM before ICD implantation [64].
Not infrequently, the waiting time rules and exposure to risk that they necessitate cause patients and physicians to be very uncomfortable. The introduction of more widespread use of wearable ICDs (WCD) (◘ Fig. 19.3) has substantially reduced risk of SCD in these waiting periods. Recently Epstein et al. reported findings in 8453 patients who had a WCD prescribed in the first 3 months post-MI [65]. A total of 133 patients (1.6 %) received 309 appropriate shocks from their WCD. Of these patients, 91 % were resuscitated from a ventricular arrhythmia. With 40-day and 3-month waiting periods in patients post-MI, the WCD successfully treated SCD in 1.4 %, and the risk was highest in the first month of WCD use.


Fig. 19.3
Life Vest, wearable cardiac defibrillator (WCD) , ZOLL Medical Corporation, Pittsburgh, PA, USA
Recommendations for Implantable Cardioverter Defibrillators (◘ Tables 19.3 and 19.4)
Table 19.3
Recommendations for implantable cardioverter defibrillators (2012 ACCF/AHA/HRS Focused Update Incorporated into the ACCF/AHA/HRS 2008 Guidelines for Device-Based Therapy of Cardiac Rhythm Abnormalities) [24]
Class I | Level of evidence |
• ICD therapy is indicated in patients who are survivors of cardiac arrest due to VF or hemodynamically unstable sustained VT after evaluation to define the cause of the event and to exclude any completely reversible causes. | A |
• ICD therapy is indicated in patients with structural heart disease and spontaneous sustained VT, whether hemodynamically stable or unstable. | B |
• ICD therapy is indicated in patients with syncope of undetermined origin with clinically relevant, hemodynamically significant sustained VT or VF induced at electrophysiological study. | B |
• ICD therapy is indicated in patients with LVEF less than or equal to 35 % due to prior MI who are at least 40 days post-MI and are in NYHA functional Class II or III. | A |
• ICD therapy is indicated in patients with nonischemic DCM who have an LVEF less than or equal to 35 % and who are in NYHA functional Class II or III. | B |
• ICD therapy is indicated in patients with LV dysfunction due to prior MI who are at least 40 days post-MI, have an LVEF less than or equal to 30 %, and are in NYHA functional Class I. | A |
• ICD therapy is indicated in patients with non-sustained VT due to prior MI, LVEF less than or equal to 40 %, and inducible VF or sustained VT at electrophysiological study. | B |
Class IIa | Level of evidence |
• ICD implantation is reasonable for patients with unexplained syncope, significant LV dysfunction, and nonischemic DCM. | C |
• ICD implantation is reasonable for patients with sustained VT and normal or near-normal ventricular function. | C |
• ICD implantation is reasonable for patients with HCM who have 1 or more major† risk factors for SCD. | C |
• ICD implantation is reasonable for the prevention of SCD in patients with ARVD/C who have 1 or more risk factors for SCD. | C |
• ICD implantation is reasonable to reduce SCD in patients with long-QT syndrome who are experiencing syncope and/or VT while receiving beta-blockers. | B |
• ICD implantation is reasonable for non-hospitalized patients awaiting transplantation. | C |
• ICD implantation is reasonable for patients with Brugada syndrome who have had syncope. | C |
• ICD implantation is reasonable for patients with Brugada syndrome who have documented VT that has not resulted in cardiac arrest. | C |
• ICD implantation is reasonable for patients with catecholaminergic polymorphic VT who have syncope and/or documented sustained VT while receiving beta-blockers. | C |
• ICD implantation is reasonable for patients with cardiac sarcoidosis, giant cell myocarditis, or Chagas disease . | C |
Class IIb | Level of evidence |
• ICD therapy may be considered in patients with nonischemic heart disease who have an LVEF of less than or equal to 35 % and who are in NYHA functional Class I. | C |
• ICD therapy may be considered for patients with long-QT syndrome and risk factors for SCD. | B |
• ICD therapy may be considered in patients with syncope and advanced structural heart disease in whom thorough invasive and non-invasive investigations have failed to define a cause. | C |
• ICD therapy may be considered in patients with a familial cardiomyopathy associated with sudden death. | C |
• ICD therapy may be considered in patients with LV non-compaction cardiomyopathy. | C |
Class III | Level of evidence |
• ICD therapy is not indicated for patients who do not have a reasonable expectation of survival with an acceptable functional status for at least 1 year, even if they meet ICD implantation criteria specified in the Class I, IIa, and IIb recommendations above . | C |
• ICD therapy is not indicated for patients with incessant VT or VF. | C |
• ICD therapy is not indicated in patients with significant psychiatric illnesses that may be aggravated by device implantation or that may preclude systematic follow-up. | C |
• ICD therapy is not indicated for NYHA Class IV patients with drug-refractory congestive heart failure who are not candidates for cardiac transplantation or CRT-D. | C |
• ICD therapy is not indicated for syncope of undetermined cause in a patient without inducible ventricular tachyarrhythmias and without structural heart disease. | C |
• ICD therapy is not indicated when VF or VT is amenable to surgical or catheter ablation (e.g., atrial arrhythmias associated with the Wolff-Parkinson-White syndrome, RV or LV outflow tract VT, idiopathic VT, or fascicular VT in the absence of structural heart disease ). | C |
• ICD therapy is not indicated for patients with ventricular tachyarrhythmias due to a completely reversible disorder in the absence of structural heart disease (e.g., electrolyte imbalance, drugs, or trauma). | B |
Table 19.4
HRS/ACC/AHA expert consensus statement on the use of implantable cardioverter defibrillator therapy in patients who are not included or not well represented in clinical trials (2013) [25]
• In patients with abnormal cardiac biomarkers that are not thought to be due to an MI and who otherwise would be candidates for implantation on the basis of primary prevention or secondary prevention criteria, implantation of an ICD is recommended. |
• Implantation of an ICD within the first 40 days following acute MI in patients with preexisting systolic ventricular dysfunction (who would have qualified for a primary prevention ICD) is not recommended. |
• In patients who, within 40 days of an MI, develop sustained (or hemodynamically significant) ventricular tachyarrhythmias >48 h after an MI and in the absence of ongoing ischemia, implantation of an ICD is recommended. |
• Implantation of an ICD for primary prevention is not recommended with in the first 3 months after initial diagnosis of NICM. |
• If recovery of left ventricular function is unlikely, implantation of an ICD for primary prevention can be useful between 3 and 9 months after initial diagnosis of NICM. |
Recommendations on the use of the ICD in clinical practice have been provided in four important guideline documents sponsored by the American College of Cardiology (ACC), the American Heart Association (AHA), Heart Rhythm Society (HRS), and the European Society of Cardiology (ESC) [66–68]. Current ICD indications and recommendations are summarized in ◘ Tables 19.3 and 19.4 [69].
Recommendations for Wearable ICD (WCD)
WCDs are recommended for patients with accepted indications for ICD implantation but who also have (usually temporary) contraindications for such a procedure [65, 70]. The most common are those in the CMS mandated “waiting periods” after acute MI or revascularization. Other temporary contraindications include an infected ICD system that requires explanation with the need for long-term antibiotic treatment. A second group of WCD candidates comprises patients under investigation for a disease with a high risk of arrhythmic death or pending definitive diagnosis (e.g., those with inheritable arrhythmic disorder who are awaiting results of confirmatory testing or survivors of a cardiac arrest of unclear (and potentially treatable or reversible) origin). The third group consists of patients with severe heart failure awaiting cardiac transplantation and, finally, patients having a condition that temporarily places them at high risk of an arrhythmic death (e.g., patients with a low LVEF resulting from potentially reversible condition such as a newly diagnosed dilated cardiomyopathy (that could be due to transient myocarditis) or an ischemic cardiomyopathy in the early period after revascularization or in the early period after a MI ).
Antiarrhythmic Drug Therapy for Atrial and Ventricular Tachyarrhythmias in Cardiomyopathy
The apparent antiarrhythmic effects of certain compounds have been recognized for well over 250 years. Cardiac glycosides and quinine are perhaps the earliest examples. However, most of the antiarrhythmic drugs (AADs ) currently in use have been introduced in only the last 35–40 years. Currently, AADs remain a mainstay for termination and prevention of atrial fibrillation (AF) and provide useful adjunctive therapy for certain forms of ventricular tachycardia (VT). Few, however, have proved effective for ventricular fibrillation prevention (with the possible exception of amiodarone and perhaps bretylium).
The primary goals of current AAD therapy have been the reduction of frequency, duration, and severity of arrhythmia episodes. Unfortunately, on the negative side, most AADs have cardiac and noncardiac adverse effects that limit their clinical utility in important segments of the patient population, particularly those with more than minimal left ventricular (LV) dysfunction and/or heart failure.
Overview of Current Antiarrhythmic Drugs
◘ Table 19.5 summarizes the most widely available antiarrhythmic drugs using the Vaughan Williams classification which focuses on each drug’s principal cardiac channel effects. However, it is recognized that drug actions are much more complex than the Vaughan Williams approach allows and that actual drug effects on arrhythmias are not readily predicted by the classification. Given this important limitation, an attempt has been made to provide a more comprehensive and precise classification of drug effects [71, 72]. This effort (the so-called Sicilian gambit ), while scientifically robust, is necessarily complex and as a result has largely been neglected in recent years.
Table 19.5
Vaughan Williams classification
Class | Drugs | Channel(s) blocked | Mechanism(s) |
---|---|---|---|
Ia | Quinidine | INa, IKr, acetylcholine | (Na+) channel block (intermediate association/dissociation) and K+ channel-blocking effect |
Procainamide | |||
Disopyramide | |||
Ib | Lidocaine | INa | (Na+) channel block (fast association/dissociation) |
Phenytoin | |||
Mexiletine | |||
Tocainide | |||
Ic | Encainide | INa, β | (Na+) channel block (slow association/dissociation) |
Flecainide | |||
Propafenone | |||
Moricizine | |||
II | Carvedilol | Beta-adrenergic blockers | |
Propranolol | |||
Esmolol | |||
Timolol | |||
Metoprolol | |||
Atenolol | |||
Bisoprolol | |||
III | Amiodarone | IKr, INa, ICa, β, α, acetylcholine | K+ channel blocker |
Sotalol | IKr, β | Sotalol is also a beta-blocker | |
Ibutilide | IKr, INa agonist | Amiodarone has Class I, II, III, and IV activity | |
Dofetilide | IKr | ||
Dronedarone | IKr, INa, ICa, β, α, acetylcholine | ||
IV | Verapamil | ||
Diltiazem | |||
Ivabradine | I f | Inhibition of the funny channel |
Excluding beta-adrenergic blockers, calcium channel blockers, and cardiac glycosides, the majority of available “membrane-active” antiarrhythmic drugs exert predominant effects on cardiac sodium or potassium currents. The first of the orally available membrane-active agents to have a prominent place in therapeutics was quinidine and its congeners (derived from quinine). Procainamide became available in the early 1950s. Thereafter, at least in the USA, there was a long delay before the emergence of disopyramide in the late 1970s. Flecainide, encainide (the latter now withdrawn from the US market), ethmozine, mexiletine, tocainide (also withdrawn), imipramine, bretylium/bethanidine (not used to any extent), and amiodarone appeared in the 1980s. Propafenone, sotalol, dofetilide, dronedarone, and ivabradine followed.
Encainide , like flecainide, is a Vaughan Williams Class 1C agent (◘ Table 19.5), but its use was discontinued in many countries (but not all) after CAST (Cardiac Arrhythmia Suppression Trial) revealed increased death rate in the treatment group [73]. Flecainide remains available due to additional studies showing benefit in supraventricular tachycardias, particularly atrial fibrillation (AF).
Tocainide is an orally available lidocaine “look-alike,” but its use was undermined by an excessive number of adverse effects (especially hematologic). Imipramine (a long-used QT-prolonging tricyclic antidepressant) was, surprisingly to many, incorporated in the pre-CAST pilot study (CAPS), but was never a serious antiarrhythmic drug contender, and reasonably dropped by the wayside as far as cardiac arrhythmia therapy was concerned in the mid-1980s [74]. Bretylium was developed in the 1970s and proved to be an interesting antifibrillatory drug and was commercially available for parenteral use in the USA. The principal indication for bretylium was termination of refractory VF and VF prevention during acute care scenarios. However, while bretylium has an excellent inotropic profile, it induced profound postural hypotension and was difficult to use; in addition, it was not available for oral administration. Bethanidine (which had been widely used as an antihypertensive drug for many years and may still be used in some countries) has bretylium-like antifibrillatory properties [75]. However, although orally absorbable, very cheap, and widely available in the world, it is also difficult to use due to induction of postural hypotension. Techniques were devised to minimize the postural hypotension limitation, but given the emergence of amiodarone, the impetus to use bethanidine largely evaporated.
Sodium channel-blocking drugs (◘ Table 19.5) are often called membrane-stabilizing agents because they decrease the excitability of cardiac tissue. Typically, these drugs exhibit use dependence. This means that the predominant effect on conduction (sodium channel blockade) is seen at rapid heart rates. On the ECG, QRS widening due to conduction slowing is often observed.
Drugs with major effects on blocking potassium currents prolong the action potential duration and refractory periods. Quinidine, procainamide, disopyramide, sotalol, and dofetilide are primarily potassium channel-blocking drugs that display reverse use dependence such that repolarization is prolonged at slow heart rates. The latter effect predisposes these drugs to lengthen the QT interval most dramatically during bradyarrhythmias and increase susceptibility to triggering torsade-de-pointes (TdP) ventricular tachycardia.
Apart from their electrophysiological effects, most available antiarrhythmic drugs also exhibit negative inotropic effects, thereby limiting their applicability in patients with diminished LV function (i.e., most patients with structural heart disease) and/or heart failure. Disopyramide and procainamide are perhaps the most negatively inotropic agents. On the other hand, quinidine, dofetilide, bethanidine (not used), and amiodarone are perhaps the most innocent agents in this regard.
Due to their negative inotropic effects, Class 1C agents and dronedarone are generally not used in LV dysfunction/heart failure patients. On the other hand, quinidine (not often used these days), dofetilide, sotalol, and amiodarone are generally acceptable in heart failure, but patient response must be carefully monitored. Other factors limiting AAD use in individual patients include the mechanism of clearance of the agent and how that might be affected by systemic disease (◘ Table 19.6). By way of example, elimination of both sotalol and dofetilide is highly dependent on renal function status, and their dosages must be adjusted accordingly. Similarly, AAD plasma concentrations may be altered by the presence of other AADs. Perhaps the quinidine-digoxin interaction was the earliest important AAD-AAD interaction to be identified, and it may have contributed to many cases of the so-called quinidine syncope (see later).
Table 19.6
Antiarrhythmic drug dosing and side effects
Antiarrhythmic drug | Metabolism/dose | Major non-cardiovascular toxicity | Major cardiovascular toxicity |
---|---|---|---|
Quinidine | • Hepatic CYP3A4 (70 %), renal (30 %) | Thrombocytopenia, cinchonism, pruritus, rash | QRS prolongation with toxic doses, torsades de pointes (not dose related) |
• Sulfate, 600 three times a day | |||
• Gluconate, 324–648 every 8 h | |||
Propafenone | • Hepatic | Metallic taste, dizziness | Atrial flutter with 1:1 conduction; ventricular tachycardia; may unmask Brugada-type ST elevation; contraindicated with coronary disease |
• 150–300 every 8 h | |||
• Sustained release 225–425 twice a day | |||
Flecainide | • Renal/hepatic CYP2D6 | Dizziness, headache, visual blurring | Atrial flutter with 1:1 conduction; ventricular tachycardia; may unmask Brugada-type ST elevation; contraindicated with coronary disease |
• 50–100 mg twice a day | |||
Sotalol | • Renal: 80–120 mg twice a day | Bronchospasm | Bradycardia, torsades de pointes |
• Maximum dose 240 mg twice a day | |||
Dofetilide | • Renal/hepatic CYP3A4 | None | Torsades de pointes |
• 500 μg twice a day | |||
• Renally dose adjusted | |||
Amiodarone | • Hepatic; half-life 50 days | Pulmonary (acute hypersensitivity pneumonitis, chronic interstitial infiltrates); hepatitis; thyroid (hypothyroidism or hyperthyroidism); photosensitivity; blue-gray skin discoloration with chronic high dose; nausea; ataxia; tremor; alopecia | Sinus bradycardia |
• Oral load 10 g over 7–10 days, then 400 mg for 3 weeks, then 200 mg/day | |||
• Intravenous: 150–300 mg bolus, then 1 mg/min infusion for 6 h followed by 0.5 mg/min thereafter | |||
Ibutilide (intravenous) | • Hepatic CYP3A4 | Nausea | Torsades de pointes |
• 1 mg intravenous over 10 min; repeat after 10 min if necessary | |||
Dronedarone | • Renal, hepatic, gastrointestinal | Anorexia; nausea; hepatotoxicity | Bradycardia |
• 400 mg twice a day | |||
Mexiletine | • Hepatic | Dizziness, heartburn, nausea, nervousness, trembling, unsteadiness | Bradycardia |
• 200 mg three time a day | |||
Disopyramide | • Hepatic | Dry mouth, constipation, Urinary retention, blurred vision | Negative inotrope |
• 125 mg four times a day | Hypotension |
Role of Specific Antiarrhythmic Drugs
Treatment of most paroxysmal supraventricular tachycardias (SVTs) is now focused predominantly on mapping and ablation, since ablation offers a cure, whereas AADs offer only palliation. Currently, AADs are primarily used for AF and to some extent for other primary atrial tachycardias (AT).
Quinidine, procainamide, and disopyramide are no longer widely used for the treatment or prevention of AF or ATs in developed countries. Although these drugs can be useful, their adverse effects and the presence of better tolerated alternatives have undermined their use; consequently, they are not discussed further in this section. Amiodarone , despite its multiple numerous side effects and lack of supportive US Food and Drug Administration labeling, has become ubiquitous in the AF population, but careful follow-up is mandatory to assess for development of adverse effects (◘ Table 19.6). Amiodarone is discussed in more detail below.
Flecainide
Flecainide , in addition to its prominent conduction slowing effect due to sodium channel-blocking activity (which may widen the QRS complex), also has mild IKr (rapid component of the delayed rectifier potassium channel) blocking effects, but is not generally associated with significant QT prolongation.
Flecainide is contraindicated in individuals with prior myocardial infarction and reduced LV function because of increased ventricular proarrhythmia risk [15]. It is also potentially hazardous in patients with conduction system disease, as it may predispose to aggravating the severity of atrioventricular (AV) block or sinus node dysfunction. Consequently, flecainide is rarely used for VT, since VT patients often have underlying LV dysfunction and/or conduction system disease. However, flecainide may be useful for prevention of AF in patients without severe structural heart disease. In this circumstance, flecainide may reduce recurrences and/or slow atrial rate in ongoing AF or AT.
On a cautionary note, it is important to recognize that flecainide-induced conduction slowing in AF patients may have unexpected adverse consequences. Flecainide (as well as other AADs, such as propafenone) can slow and regularize AF resulting in new-onset atrial flutter (so-called Type 1C flutter) [76]. The occurrence of “1C flutter,” which typically exhibits a slower atrial rate than does conventional atrial flutter, can result in a paradoxical increase of ventricular rate due to lesser block at the AV node level. The outcome may be substantial clinical distress. Thus, flecainide must be used in conjunction with a beta-blocker or calcium channel blocker to slow AV nodal conduction in the event 1C flutter develops.
In AF, flecainide may be used as first-line therapy in patients without structural heart disease. Oral flecainide (200–300 mg) has been used as a “pill-in-the-pocket” approach in patients who have infrequent AF and are capable of recognizing onset of an episode so they know to take the medication (preferably within an average of 30 min of arrhythmia onset).
Class IC antiarrhythmic agents such as flecainide are no longer recommended as therapy for VT in patients with ischemic heart disease or LV dysfunction from any cause. This limitation arose as a result of the CAST trial findings which showed that both all-cause mortality and arrhythmic death were increased with both encainide and flecainide. This exclusion of flecainide has been extended to nonischemic cardiomyopathy patients as well, despite the fact that they were not evaluated in CAST.
As a rule, flecainide tends to be well tolerated. However, common non-cardiovascular side effects include dizziness and visual disturbance in 5–10 % of patients [77].
Propafenone
Propafenone has beta-adrenergic blocking properties in addition to its 1C sodium channel-blocking activity. It also has mild negative inotropic and chronotropic effects.
In AF and AT, propafenone may be used as first-line therapy in patients without structural heart disease (typically 150 mg BID). High-dose oral propafenone (450–600 mg) has also been used as a “pill-in-the-pocket” approach in paroxysmal AF patients. As with flecainide, propafenone should be partnered with an AV nodal-blocking drug. Propafenone is not recommended for most VT patients with LV dysfunction.
The major non-cardiovascular adverse effects of propafenone include a metallic taste as well as dizziness and visual disturbances. High-dose oral propafenone (450–600 mg) has been used as a “pill-in-the-pocket” approach in patients who have infrequent AF and are capable of recognizing onset of an episode, preferably within an average of 30 min of arrhythmia onset.
Sotalol
Sotalol is a potassium channel (IKr) blocker and beta-blocker with minimal non-cardiovascular side effects and a high rate of utilization. Sotalol is cleared by the kidneys and is prescribed twice daily unless the creatinine clearance is low (between 30 and 60 mL/min) when single daily dosage is used. It is often started as an inpatient at a dose of 80 mg twice daily and up-titrated with attention to QT prolongation. The potassium channel-blocking effect increases with increasing dosage, and, as a result, the risk of torsade-de-pointes ventricular proarrhythmia (TdP) increases at a higher dosage.
Initially, sotalol was approved for treatment of AF with a recommendation for inpatient initiation. However, most recent guidelines allow for it to be started as an outpatient [15]. The Sotalol Amiodarone Atrial Fibrillation Efficacy Trial (SAFE-T) initiated either sotalol or amiodarone in the outpatient setting during AF without adverse effect and with an equivalent rate of restoring sinus rhythm [76]. In the OPTIC trial examining the potential to reduce shock frequency in secondary prevention ICD recipients, amiodarone plus beta-blockers was shown to be superior to monotherapy with sotalol or beta-blockers [78].
Sotalol is considered reasonable as first-line therapy for patients with coronary artery disease and relatively preserved left ventricular function. Sotalol may also be considered as an option for first-line therapy in VT, particularly in patients in whom beta-blockade is tolerated. The principal sotalol side effects parallel those of most beta-blockers and include fatigue, bronchospasm, and dyspnea. Sotalol can also exacerbate sinus node dysfunction (◘ Table 19.6).
Dofetilide
Dofetilide is also primarily an IKr blocker, without other clinically significant electrophysiological effects. It is cleared by the kidneys and must be dosed according to creatinine clearance (◘ Tables 19.1 and 19.2). It was approved for use in the USA in 2000 with a 3-day mandatory in-hospital loading period. ◘ Figure 19.4 shows an onset of TdP during inpatient dofetilide loading. Dofetilide is more effective for the maintenance of sinus rhythm in AF patients than it is for restoring sinus rhythm [79].


Fig. 19.4
50-year-old male with a history of ischemic cardiomyopathy with EF 20–25 % with a single-chamber ICD and difficult-to-control AF who presented as an elective admission for attempted chemical cardioversion with dofetilide. Two hours after his second dose of dofetilide, the patient developed torsades de pointes and received a shock from his ICD. Note the long-short sequence prior to initiation of tachycardia
Dofetilide is a reasonable first-line therapy choice in AF/AT patients in whom coronary artery disease is present and especially if associated with LV dysfunction. It has been demonstrated to be relatively safe in the settings of heart failure and post-myocardial infarction populations [80, 81]. Dofetilide is not recommended for use in VT; however, it has been used off-label when other more conventional options are contraindicated.
The principal risk factors for dofetilide adverse effects (particularly TdeP) include hypokalemia, hypomagnesemia, female gender, baseline prolonged QT interval, or congenital long-QT syndrome and concomitant use of other QT-prolonging therapies. It is one of the few antiarrhythmics that have little if no effect on sinus node function.
Amiodarone
Amiodarone is the most commonly prescribed antiarrhythmic drug for AF/AT, despite not having been approved by the US FDA for that indication. Amiodarone is a complex iodinated compound that acts on multiple channels including antagonism of α- and β-adrenergic receptors (◘ Fig. 19.2). It is the most effective antiarrhythmic drug currently available, but its ease of use is limited by a myriad of non-cardiovascular side effects [82]. The major cardiovascular side effect of amiodarone is sinus bradycardia, with a higher risk of pacemaker requirement in women [83].
QT prolongation is common with amiodarone but fortunately is very rarely associated with TdP (<0.5 %) [84]. The combination of amiodarone with the CYP3A4 substrate simvastatin has been associated with an increased risk of myositis [85]. Conversely, this risk seems to be smaller when amiodarone is combined with pravastatin, which does not use the cytochrome P450 system for metabolism. The most important drug-drug interaction with amiodarone occurs with potentiation of the anticoagulant effect of warfarin through inhibition of CYP2C9. In addition, amiodarone can reduce digoxin clearance.
Amiodarone is orally well absorbed with high bioavailability, but it may also be administered as an intravenous agent; the parenteral form has been used for terminating AF, but in reality it is only weakly effective for this purpose [86]. On the other hand parenteral amiodarone is a very effective agent for slowing ventricular response in AF.
A major limitation of amiodarone is its exceedingly long half-life, 58 days (range 15–142 days). As a result, it takes a long time to “load” amiodarone and also a long time to eliminate it if side effects become an issue. As rule, amiodarone can be loaded orally over the course of 3–4 weeks. A day or two of parenteral loading (0.5–1 mg/min IV) may help accelerate loading and is generally well tolerated.
EMIAT and CAMIAT evaluated amiodarone use in patients recovering from myocardial infarction (MI) [87, 88]. EMIAT was a European randomized double-blind placebo-controlled trial to assess whether amiodarone reduced all-cause mortality (primary endpoint) and cardiac mortality and arrhythmic death (secondary endpoints) in survivors of myocardial infarction with a LV ejection fraction (LVEF) of 40 % or less. CAMIAT was a Canadian randomized double-blind placebo-controlled trial designed to assess the effect of amiodarone on the risk of resuscitated ventricular fibrillation or arrhythmic death among survivors of myocardial infarction with frequent or repetitive PVCs. Both reports found that incidences of cardiovascular death and arrhythmic death or resuscitated cardiac arrest were significantly lower in patients receiving both beta-blockers and amiodarone than in those not receiving beta-blockers, with or without amiodarone. TdP occurred in less than 1 % of patients in the EMIAT and CAMIAT trials.
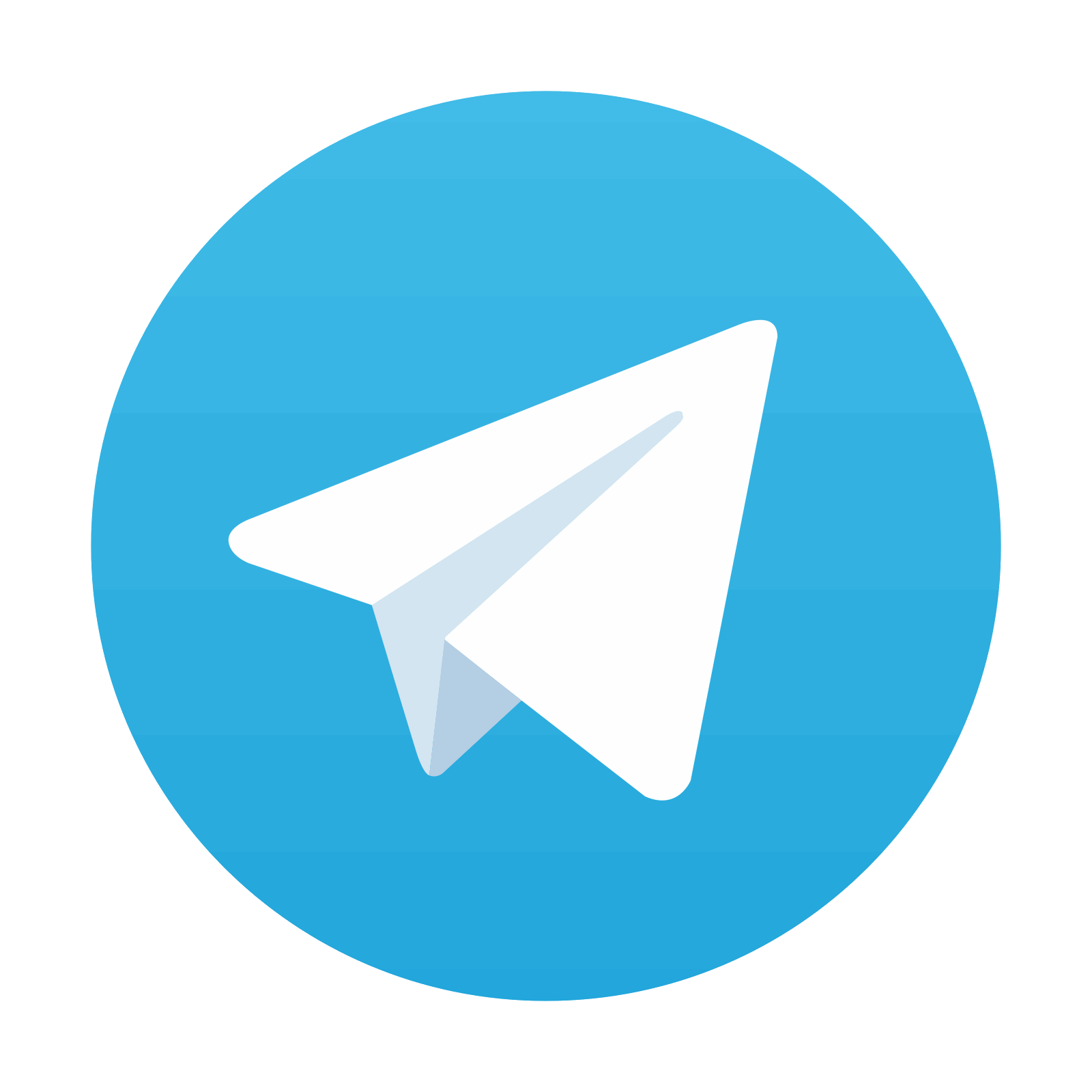
Stay updated, free articles. Join our Telegram channel

Full access? Get Clinical Tree
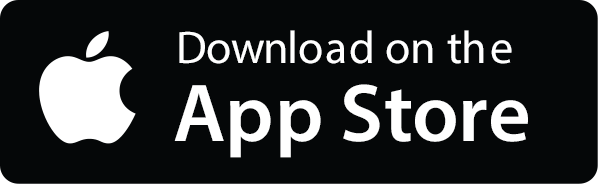
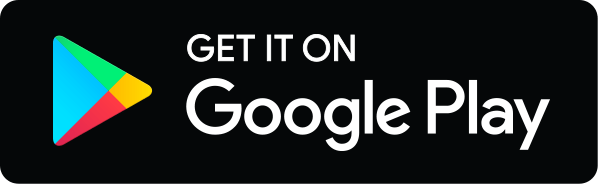