Abstract
Abnormalities of cardiac rhythm are important causes of cardiovascular disability, stroke, and sudden cardiac death. Two general approaches have been used to identify genes playing a role in arrhythmia susceptibility. First, studies in rare familial arrhythmia syndromes such as the long-QT syndrome have provided new insights into mechanisms of normal cardiac electrical behavior, arrhythmogenesis, and variable responses to antiarrhythmic drug therapy. The other major approach has used genome-wide approaches in large populations of normal subjects or of patients with common arrhythmias, notably atrial fibrillation, to identify new pathways in electrical signaling. This review describes the way in which these approaches are refining our view of the arrhythmia prone heart, and how understanding mechanisms identified in these studies can point to new approaches to arrhythmia treatment and prevention.
Keywords
Long-QT syndrome, atrial fibrillation, sudden cardiac death, ventricular fibrillation, torsades de pointes, ion channels, genome-wide association, proarrhythmia
Chapter Outline
Scope of the Problem 179
Overview of Cardiac Electrical Activity 180
From Rare Disease Genetics to Common Arrhythmias: The Long-QT Syndrome Example 180
Long-QT Syndrome Disease Genes 181
A Brief Primer in Normal and Abnormal Cardiac Repolarization 182
Defective Channel Function in cLQTS 182
Clinical Correlates of LQTS Mutations 183
Other LQTS Disease Genes and Variants 183
Genome-Wide Approaches to Studying the QT Interval 186
Acquired Long-QT Syndrome 186
Generalizing to Other Familial Arrhythmia Syndromes 190
From a Common Disease to Genetics: The AF Example 191
AF Mechanisms: Overview 191
Common and Rare Genetic Variants Associated With AF Risk 191
Antiarrhythmic Drug Pharmacogenetics 192
Application of Genomics to Arrhythmia Practice: Current Status and Future Prospects 193
References
Scope of the Problem
Abnormalities of cardiac rhythm can range from single abnormal ectopic beats to abnormally slow or rapid heart rates and are important causes of morbidity and mortality. Atrial fibrillation (AF) is the commonest arrhythmia requiring therapy and is a major cause of stroke. The incidence rises with age, reaching >10% in those above 80-year old; interestingly, despite its being a disease of the elderly, available evidence outlined below shows a prominent genomic component to risk. The other major arrhythmia considered here is sudden cardiac death (SCD), which kills over 250,000 Americans annually. The commonest cause is ventricular fibrillation (VF), usually within minutes of occlusion of a coronary artery. Sadly, in about half of victims, SCD is actually the initial manifestation of coronary artery disease, and so identifying patients at risk beforehand represents a major challenge to contemporary cardiac electrophysiology to which genomic markers may contribute. Other causes of SCD include other types of underlying heart diseases (including cardiomyopathies, a condition with a prominent genomic component) as well as primary familial arrhythmia syndromes, such as the long-QT syndrome discussed below. Defining disease genes and mechanisms in these instances is not only informing etiology and treatment in affected patients but is elucidating new pathways that control normal cardiac rhythm and susceptibility to common arrhythmias like AF and SCD in the general population.
Overview of Cardiac Electrical Activity
The normal heart beat is generated by the orderly propagation of a wave of excitation generated by action potentials, electrical signals 200–400-ms long in humans, reflecting depolarization and subsequent repolarization in individual heart cells. Abnormalities of cardiac rhythm arise when activity in individual cells is abnormal (e.g., action potentials are generated too rapidly) or abnormal conduction pathways are present (e.g., due to disease-related scarring). Orderly depolarization and repolarization in cardiac cells is driven by the activity of ion channels, multimeric protein complexes consisting of pore-forming structures (“alpha-subunits”), and ancillary function-modifying subunits. Ion channels open and close in response to changes in their environment (e.g., the presence of a ligand or a change in voltage) and when open conduct specific ions along their electrochemical gradients. This chapter will emphasize how genomic tools have been critical in advancing much of our contemporary understanding of the molecular basis of the normal action potential and of normal propagation, and how these processes are perturbed by DNA variation or disease to cause arrhythmias. Drugs used to treat cardiac arrhythmias are effective in only some patients and carry a risk of serious adverse drug reactions (ADRs), most notably the potential to trigger serious arrhythmias—the phenomenon of “proarrhythmia.” The pharmacogenomics of antiarrhythmic therapy, variability in response, and susceptibility to proarrhythmia, will also be discussed.
From Rare Disease Genetics to Common Arrhythmias: The Long-QT Syndrome Example
Studies of the congenital long-QT syndrome (cLQTS) are outlined here as a model for how understanding mechanisms in a rare genetic disease is informing practice. The lessons learned apply to other congenital arrhythmia syndromes, discussed briefly later, and in the tables. The QT interval is signature on the surface electrocardiogram (ECG) of repolarization in the ventricle; a long-QT interval, normalized or corrected for rate, indicates prolongation of cardiac action potentials in at least some cells in the ventricle ( Fig. 11.1 ). The cLQTS was described in the 1950s as an autosomal recessive disease (the Jervell–Lange-Nielsen syndrome) characterized by extraordinary prolongation of the QT interval on the surface ECG, congenital deafness, and a risk of abrupt episodes of loss of consciousness (syncope) and SCD in affected children. The commoner autosomal dominant form (the Romano–Ward syndrome), characterized by less extreme QT prolongation, no deafness, but a risk of syncopal episodes and of SCD, was described in the 1960s. Initial reports noted that many but not all episodes of syncope and SCD occurred with emotional or physical exertion, and beta blockers appear effective in reducing these events. A morphologically distinctive polymorphic ventricular tachycardia termed torsades de pointes is the cause of syncope and SCD in cLQTS.

Long-QT Syndrome Disease Genes
In the mid-1990s, linkage in large kindreds followed by sequence analysis of candidate genes in linked regions identified the three major cLQTS disease genes, all encoding ion channel alpha-subunits, in the potassium channel genes KCNQ1 and KCNH2 and in the major cardiac sodium channel gene SCN5A . It is (conveniently enough) relatively simple to generate ion channel cDNAs, transfect these into cells that do not ordinarily demonstrate significant electrical activity, and 1–2 days later use voltage clamp approaches to study the individual ion currents. Using this heterologous expression approach, a mainstay of contemporary experimental electrophysiology, to compare mutant and wild-type ion channels has defined mechanisms controlling normal cardiac depolarization and has elucidated mechanisms in cLQTS.
A Brief Primer in Normal and Abnormal Cardiac Repolarization
The opening of cardiac sodium channels allows sodium entry into cells and generates the fast depolarization that initiates the action potential in atrial, ventricular, and conduction system cells. This initial depolarization then opens calcium channels; the resultant entry of calcium into cells not only maintains depolarization but also provides a trigger for calcium release from intracellular stores (the sarcoplasmic reticulum). This calcium-induced calcium release further amplifies the rise in intracellular calcium and is responsible for the contraction of individual heart cells. The initial depolarization also activates potassium channels which allow potassium egress from cells to repolarize the cell. A sodium–calcium exchanger and the sodium–potassium pump restore normal sodium and calcium concentrations with each action potential. This general description emphasizes the highly interactive nature of the various individual components of electrical signaling; as a consequence, understanding the functional consequences of dysfunction of one component (e.g., arising from a DNA variant) requires consideration of how a single lesion may perturb function of the entire system. For example, reduction of the amplitude of potassium currents will delay repolarization and prolong action potentials; as a result, calcium channels may stay open longer (or reopen after closing) generating abnormal upstrokes that can serve as a trigger abnormal rhythms. Computational modeling can be a useful tool to understand how abnormal function of one channel affects other channels and the action potentials they together generate .
Defective Channel Function in cLQTS
First electrophysiologic principles assert that long-QT intervals reflect increased action potential durations in the ventricle ( Fig. 11.1 ), and thus either decreased repolarizing (predominantly potassium) current or increased repolarizing (calcium or sodium) current. KCNQ1 and KCNH2 mutations causing cLQTS are loss-of-function variants: they cause prolonged action potential durations in the ventricle by decreasing major outward repolarizing potassium currents, termed I Ks ( KCNQ1 ) and I Kr ( KCNH2 ) . Heterologous expression experiments have demonstrated multiple subtypes of defects, including decreased cell surface expression (presumably reflecting decreased trafficking to the cell surface of proteins recognized as misfolded) and abnormal gating of channels that do reach the cell surface. These potassium channels are actually tetrameric, i.e., four separate proteins assemble to generate the alpha-subunit. As a result, in some instances, even in the autosomal dominant form of the disease, a single mutant channel may produce >50% reduction of channel function, a dominant negative effect. SCN5A mutations causing cLQTS do so by increasing a “persistent” inward sodium current which is ordinarily absent or quite small during the plateau phase of the action potential . This increased “late” current reflects destabilization of a process termed fast inactivation and is often (probably incorrectly) termed a “gain of function” mutation. The Jervell–Lange-Nielsen syndrome arises in individuals who are homozygotes (usually due to consanguinity) or compound heterozygotes for loss-of-function variants in either KCNQ1 or in KCNE1 , encoding a function-modifying I Ks subunit. This loss of I Ks function also perturbs early inner ear development to cause congenital deafness .
Clinical Correlates of LQTS Mutations
The identification of disease genes in cLQTS led to recognition of large kindreds in which some mutations carriers had normal QT intervals, the phenomenon of incomplete penetrance . Indeed, as with many other familial arrhythmia and other conditions, as recognition of the syndrome and availability of commercial genetic testing has become widespread, it is clear that many mutation carriers have normal QT intervals and are at very low risk for syncope or SCD. The identification of three major disease genes has now allowed the association of specific clinical features with the different genes and, in some cases suggests specific therapeutic approaches ( Table 11.1 ) . A common feature is that randomized clinical trials have generally not been feasible in cLQTS and other familial arrhythmia syndromes conditions because cases are rare.
Molecular Defect | Major Disease Gene (Subtype) | Protein Encoded | Frequency Among All cLQTS Patients | Other Disease Genes Producing a Similar Phenotype | Protein Encoded | In Vitro Functional Defects | Provokers of Syncope or SCD | Other Features |
---|---|---|---|---|---|---|---|---|
↓Slow component of delayed rectifier (I Ks ) | KCNQ1 (LQT1) | I Ks alpha subunit | 50% | KCNE1 | I Ks function-modifying subunit | Altered channel gating; ↓cell surface expression | ExertionEmotional stress Diving or swimming |
|
AKAP9 | I Ks function-modifying subunit | |||||||
↓Rapid component of delayed rectifier (I Kr ) | KCNH2 (LQT2) | I Kr alpha subunit | 35% | KCNE2 | I Kr function-modifying subunit | ↓Cell surface expression; altered channel gating | Auditory stimuli (fire alarm, telephone) |
|
↑Persistent (“late”) sodium current ( I Na ) | SCN5A (LQT3) | Cardiac sodium channel alpha subunit | 10% | CAV3 | Caveoli n -3 | Destabilized fast inactivation | Sleep/rest |
|
SCN4B | I Na function-modifying subunit | |||||||
SNTA1 | Syntrophin alpha 1 (membrane scaffold) | |||||||
Altered ankyrin B function | Rare | ANK2 (LQT4) | Ankyrins link membrane proteins to the cytoskeleton | Altered expression of pumps and exchangers |
| |||
↑L-type calcium current ( I Ca-L ) | Rare | CACNA1C | L-type calcium channel alpha subunit | Slowed inactivation |
| |||
Altered calmodulin function | Rare | CALM1CALM2 | Calmodulins | Slowed I Ca-L inactivation (?) |
| |||
↓Inward rectifier channels | Rare | KCNJ2 | Inward rectifier potassium channel subunit |
| ||||
↓I K-Ach | Rare | KCNJ5 | Acetylcholine-gated potassium channel subunit |
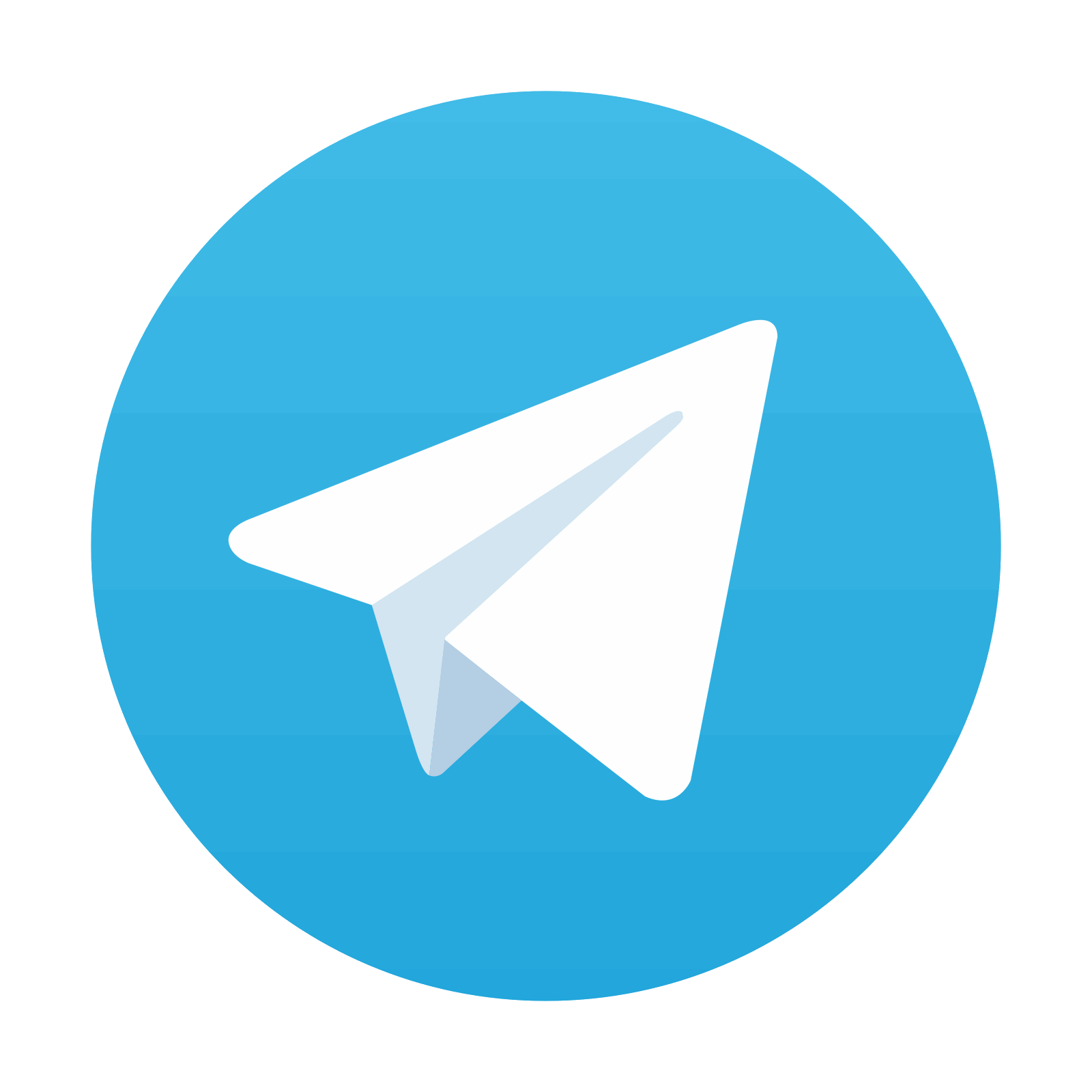
Stay updated, free articles. Join our Telegram channel

Full access? Get Clinical Tree
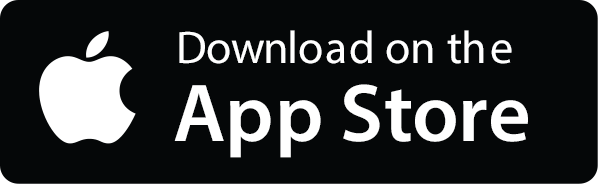
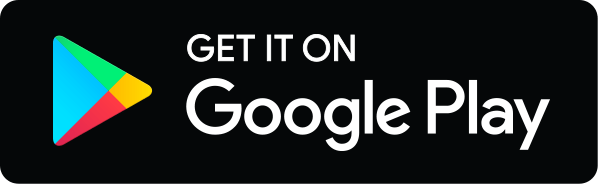
