Respiratory distress in the setting of normal peripheral perfusion and without overt cyanosis is the least common manifestation of symptomatic cardiovascular disease in the newborn. Particularly in the absence of a murmur, the diagnosis of heart disease is often delayed or missed entirely because respiratory distress alone in an acyanotic infant with normal perfusion is most often caused by lung disease rather than intrinsic cardiac disease. Furthermore, symptoms usually develop gradually over the first few days or weeks of life, and the respiratory symptoms, such as tachypnea with feeding, are often subtle. It may take several weeks or more to recognize that the infant is growing poorly and that heart disease may be the cause. This chapter reviews structural cardiovascular defects that can cause respiratory distress with normal systemic perfusion; obstructive structural heart disease is discussed in Chapter 8; cardiomyopathies and arrhythmias are discussed in Chapters 9 and 10, respectively; and heart failure is discussed in Chapter 11.
A diverse group of congenital structural cardiovascular defects share the common feature of increased pulmonary blood flow as the main pathophysiologic process. It is this common characteristic that is the basis for the majority of signs and symptoms caused by this group of defects. The arterial oxygen saturation, although sometimes mildly decreased, is not so low that either cyanosis is present or systemic oxygen delivery is compromised.
The primary symptom in these infants is tachypnea, often accompanied by mildly increased work of breathing. In addition to tachypnea, many of these infants exhibit other signs and symptoms of the heart failure syndrome (Chapter 11). These infants have heart failure with high cardiac output (“high-output failure”), which is very different than the low-output failure that occurs in adults with acquired heart disease and in neonates with decreased systemic perfusion (Chapters 8 and 11). In addition to increased pulmonary blood flow, systemic blood flow is often increased in response to the increased metabolic demands resulting from the greater respiratory effort. The increased cardiac output leads to greater circulating blood volume to maintain normal filling pressures. The heart is hypercontractile, and systemic and pulmonary venous filling pressures are usually normal. Peripheral edema does not occur because venous pressures are not increased. However, hepatomegaly is a fairly constant finding because the liver and hepatic veins are very compliant and enlarge to accommodate the increased circulating blood volume.
Oxygen consumption, or metabolic demand, is increased for a variety of reasons. The major contributor is the increased work of breathing. In a normal infant, breathing is a large component of basal oxygen consumption (20%), which is similar to the metabolic requirements for growth. As the work of breathing increases, it may comprise 30% to 40% of oxygen consumption. An increase in adrenergic drive is necessary to maintain the increased combined ventricular output, and this too increases oxygen consumption, particularly by stimulation of brown fat metabolism. This increased adrenergic drive is mediated by both neural and hormonal mechanisms and causes two other common signs of high-output heart failure: tachycardia and diaphoresis. In infants, diaphoresis is observed on the forehead and scalp, especially during feeding.
Finally, failure to thrive is an important and frequent component of the clinical presentation. It is caused by the increased caloric requirements associated with increased oxygen consumption and by decreased caloric intake. These infants feed poorly because of their increased respiratory effort; they vomit frequently because of gastroesophageal reflux in the presence of increased intra-abdominal pressure, which in turn is caused by increased respiratory effort. The caloric intake required for growth in a normal infant is about 100 to 120 kcal/kg/d. Failure to thrive occurs because the infant in high-output failure may take in only a fraction of this amount. These infants may require 160 to 180 kcal/kg/d to maintain a normal growth rate because of increased metabolic demands.
The mechanism for tachypnea in infants with increased pulmonary blood flow is not known. Left atrial pressures are generally normal. Ventilation and oxygenation also are not impaired, which indicates that significant alveolar edema is not present. The increased amount of fluid in the thorax caused by increased pulmonary blood flow, particularly distributed in the pulmonary veins, may make it more difficult for the infant to breathe by increasing the weight of the lungs, but this has never been shown to be an independent factor in respiratory work. More likely, the increased production of interstitial fluid with the attendant increase in lymphatic flow is the primary cause of tachypnea in these infants.
As blood flows normally through the lungs, interstitial fluid is produced. This fluid is drained from the interstitial spaces by the lymphatic vessels. As blood flow increases, lymphatic flow increases similarly. Defects with high pulmonary arterial pressures increase precapillary pressures, which further increase lymphatic flow. As interstitial fluid production increases, the ability of the lymphatics to drain the fluid may be exceeded, causing accumulation of interstitial fluid. The fluid is predominantly peribronchial, which may impair bronchial function. Airway size may decrease, and airway resistance may increase, further increasing the work of breathing. In addition, the infant may be more likely to develop wheezing, as only a small further decrease in airway lumen would impair air exchange. This scenario explains not only the tachypnea universally seen in infants with a symptomatic increase in pulmonary blood flow but also the radiographic findings of increased vascular markings, the presence of peribronchial edema, and other manifestations of interstitial but not of alveolar fluid. It also explains why some of them (especially those without murmurs) initially are diagnosed as having bronchiolitis.
Infants with normal lung size and function and no abnormalities other than cardiovascular disease do not develop tachypnea and failure to thrive until pulmonary blood flow is very high, usually exceeding two and a half times the normal flow. The onset of symptoms tends to track with the time course over which pulmonary blood flow increases after birth. In the normal infant, systemic blood flow peaks at around 6 to 10 weeks of age, at the time of the nadir of hemoglobin concentration. Pulmonary vascular resistance also decreases during this time period. Thus, pulmonary blood flow increases greatly during this time in the infant with a cardiovascular defect associated with increased pulmonary blood flow—there is an increase in systemic blood flow associated with physiologic anemia concomitant with an increase in the relative proportion of blood flow to the lungs (an increased pulmonary-to-systemic blood flow ratio) because of the decreased pulmonary vascular resistance.
In contrast, infants with intrinsic pulmonary disease who also have excessive pulmonary blood flow may develop tachypnea and heart failure at a level of pulmonary blood flow that is only modestly higher than normal. A common situation is an infant born prematurely who develops bronchopulmonary dysplasia. The presence of even a minor cardiac structural defect (such as an atrial septal defect) that would not cause symptoms in a normal-term infant may result in significant symptoms in this setting. The onset of symptoms and the requirement for medical and/or surgical intervention are often accelerated in this subset of infants.
Many congenital cardiovascular defects cause an increase in pulmonary blood flow as the dominant pathophysiological process, yet some are categorized according to other manifestations that are of less importance with regard to the clinical presentation. Truncus arteriosus and total anomalous pulmonary venous connection without obstruction are good examples. Because some degree of systemic arterial desaturation occurs in both of these conditions, they are often classified as “cyanotic heart disease.” However, in contrast to classical cyanotic defects (such as d-transposition of the great arteries and tetralogy of Fallot), truncus arteriosus and total anomalous pulmonary venous connection without obstruction are associated with only modest systemic arterial desaturation that may not be recognized visually. Furthermore, the predominant symptoms are tachypnea and failure to thrive, which are secondary to excessive pulmonary blood flow, not cyanosis. These defects should therefore be categorized among those that cause excessive pulmonary blood flow rather than cyanosis.
Defects that cause excessive pulmonary blood flow can be divided into two distinct subgroups. In both subgroups, excessive pulmonary blood flow occurs because a large volume of saturated pulmonary venous blood is diverted back into the pulmonary arteries via an abnormal communication (ie, a large left-to-right shunt is present). In the first subgroup, only a left-to-right shunt is present. No desaturated systemic venous blood is diverted into the systemic arterial bed. Thus, systemic arterial saturation is normal and equals that in the pulmonary veins (Figure 7-1). The second subgroup of defects also has a large left-to-right shunt but is distinguished by the additional presence of a right-to-left shunt; that is, systemic venous blood is diverted back into the systemic arterial circulation before passing through the pulmonary arterial system (Figure 7-2). Thus, some degree of systemic arterial desaturation is present in these infants. However, the dominant (or net) shunt is still left to right. Pulmonary blood flow is markedly increased, and systemic blood flow is normal or mildly increased. Consequently, the extent of systemic arterial desaturation is only modest, and the clinical effects of that desaturation are negligible.
FIGURE 7-1.
Exclusive left-to-right shunt. In this diagram of a ventricular septal defect, much of the fully saturated pulmonary venous blood (PV) passes through the ventricular septal defect to join the poorly saturated systemic venous blood (SV). This left-to-right shunt increases the saturation of pulmonary arterial blood (PA) above systemic venous saturation. However, none of the systemic venous blood crosses the ventricular septal defect to enter the aorta. Thus, the oxygen saturation of systemic arterial blood (SA) is normal and equal to that in the pulmonary veins.

FIGURE 7-2.
Predominant left-to-right shunt with an associated right-to-left shunt. In this idealized diagram of total anomalous pulmonary venous connection, the large volume of fully saturated pulmonary venous blood (PV) fully mixes in the right atrium with the smaller volume of systemic venous blood (SV). The majority of this fully mixed blood passes into the right ventricle to enter the pulmonary artery, and a lesser amount crosses the foramen ovale to the left side of the heart and into the aorta. Thus, pulmonary arterial (PA) and systemic arterial blood (SA) have the same oxygen saturation, which is determined by the relative volumes of pulmonary venous and systemic venous blood flows.

These principles are illustrated by considering an infant with truncus arteriosus with the following scenario: (1) all systemic and pulmonary venous blood mixes completely in the combined outflow tract so that aortic and pulmonary arterial blood oxygen saturations are the same, (2) pulmonary blood flow has increased to four times the systemic normal blood flow after 1 week of life, (3) pulmonary venous blood is nearly fully saturated at 98%, and (4) systemic venous blood is less saturated than normal, at 50%, because of the increased respiratory work and decreased systemic arterial saturation. Therefore, four volumes of pulmonary venous blood mix with one volume of systemic venous blood in the ventricular outflow, and that blood is ejected out the aorta and pulmonary arteries. When these five volumes of blood mix, the oxygen saturation of the fully mixed blood is
Thus, this infant has a systemic arterial saturation of about 88%. Moreover, the systemic arterial saturation in such an infant is even higher because of preferential flow of left ventricular blood anteriorly and rightward, toward the ascending aorta (see discussion of truncus arteriosus below). The infant may not appear cyanotic because the threshold for observing cyanosis is about 85% with a normal hemoglobin concentration and is even lower at a few weeks of age because of the normal physiologic anemia. In addition, oxygen delivery to the tissues is not impaired because normally only about 25% of the oxygen delivered is consumed. Tachypnea is a prominent manifestation because of high pulmonary blood flow, and failure to thrive would be present for the reasons described earlier. Thus, categorizing such an infant as having “cyanotic heart disease” is not consistent with either the physical examination or the pathophysiology of the defect. Instead, categorizing truncus arteriosus as a defect with “excessive pulmonary blood flow” is more appropriate on both accounts.
Infants with excessive pulmonary blood flow can therefore be categorized as having an exclusive left-to-right shunt or as having bidirectional shunting but with a dominant left-to-right shunt. Within each category, the defects can be considered according to the anatomic level, beginning arbitrarily with left-to-right shunts that empty into the right atrium and progressing through the right ventricle, pulmonary arteries, and the systemic veins (Tables 7-1 and 7-2).
Anatomic level | Structural defect |
---|---|
Atrial septum | Secundum atrial septal defect Primum atrial septal defect Sinus venosus atrial septal defect |
Atrioventricular septum | Complete atrioventricular septal defect Partial atrioventricular septal defect |
Ventricular septum | Inlet ventricular septal defect Perimembranous ventricular septal defect Muscular ventricular septal defect (may cause symptoms if a large mid-muscular defect is present) Outlet ventricular septal defect |
Truncal/aortopulmonary septum | Aortopulmonary window Anomalous origin of the right pulmonary artery from the ascending aorta |
Arterial communication | Patent ductus arteriosus Arteriovenous malformation |
Venous communication | Partial anomalous pulmonary venous connection |
Anatomic level | Structural defect |
---|---|
Atrial septum | Common atrium (usually left atrial isomerism) |
Atrioventricular septum | Complete atrioventricular septal defect with common ventricle (usually left atrial isomerism) |
Ventricular septum | Single ventricle physiology:
|
Truncal/aortopulmonary septum | Truncus arteriosus |
Venous communication | Total anomalous pulmonary venous connection without obstruction |
This section will consider only congenital cardiovascular defects with exclusive left-to-right shunts that cause symptoms in the otherwise normal infant. Several defects associated with left-to-right shunting will not be considered because they do not cause symptoms. For example, left-to-right shunting across an atrial septal defect occurs when the stiffness of the left ventricle is greater than that of the right ventricle. Newborn infants have a right ventricle that is of equal or greater thickness and stiffness as the left ventricle so that the left-to-right shunt at birth is small or nonexistent (Chapter 3). Although pulmonary vascular resistance begins to fall immediately at birth, it takes months for the right ventricle to remodel and accept a significantly greater amount of blood than the left ventricle. In addition, pulmonary arterial pressures are normal so that less interstitial fluid is produced for the same level of pulmonary blood flow. Thus, unless the infant has lung disease, even a large atrial septal defect does not cause symptoms in newborns and young infants.
At the atrioventricular and ventricular levels (eg, a ventricular septal defect), the magnitude of the left-to-right shunt increases much more rapidly after birth. These shunts depend on the relative resistances of the pulmonary and systemic vascular beds. The shunt occurs almost exclusively in systole when the distal vascular bed (the resistance vasculature) is filled with blood from the previous contraction, which limits the extent of the shunt. Although pulmonary vascular resistance falls precipitously immediately after birth, it does not fall so low that a systolic shunt will be large enough to cause symptoms. The further steady decline in pulmonary vascular resistance over the next several weeks of life occurs simultaneously with the normal decline in hemoglobin and hematocrit (physiologic “anemia” of infancy). Together, these processes promote a progressive increase in left-to-right shunting and pulmonary blood flow to levels high enough to cause symptoms. Typically, symptoms are noted between 4 and 8 weeks of age. An exception is a small number of infants with an atrioventricular septal defect who have a direct communication between the left ventricle and the right atrium or severe mitral insufficiency. Both of these conditions lead to a large atrial left-to-right shunt; the shunt is obligatory in that atrial pressure is always much lower than left ventricular pressure during ventricular systole. In these neonates, a very large left-to-right shunt may develop within the first days after birth, causing early symptoms of heart failure.
At the arterial level (eg, a patent ductus arteriosus), the shunt occurs during both systole and diastole. Diastolic shunts are always much larger than the corresponding systolic shunt because blood continues to flow from the resistance vessels of the lungs during diastole, affording much more vascular space to be filled. Thus, shunts at the arterial level are likely to cause high-output heart failure at an earlier age than shunts that occur at the ventricular level. These infants are frequently tachypneic when they leave the hospital after birth, but it may not be appreciated until they return for postnatal follow-up and are found to be failing to thrive.
If there is a large connection at the ventricular or arterial level, pulmonary arterial systolic pressure is equal to that in the aorta; these defects are considered “nonrestrictive.” If the amount of shunting is limited by the size of the connection, pulmonary arterial systolic pressures are less than systemic, and the defect is considered “pressure restrictive.” It is important to be aware that a pressure-restrictive connection can have a large amount of flow across it.
The presence of elevated pressures in the pulmonary vascular bed alters the normal regression of pulmonary arterial musculature. In the fetus and newborn, the small pulmonary arteries have a thicker medial smooth muscle layer in relation to diameter than similar arteries in adults. Birth is normally associated with a marked relaxation of these vessels, leading to a large decrease in pulmonary vascular resistance and increase in pulmonary blood flow (Chapter 3). The high pulmonary arterial pressures resulting from a nonrestrictive defect prevent regression of this muscle. In addition, increased blood flow in the presence of elevated pressures creates shear forces on the distal pulmonary arteries and arterioles, which, if uncorrected, can lead to irreversible damage to these vessels (pulmonary vaso-occlusive disease). This occurs over the first few years of life, more rapidly in the unrestrictive arterial level shunt (eg, ductus arteriosus) because pressure is increased not only in systole, as occurs in a ventricular level communication, but also during diastole.
Shunts that occur at an arteriovenous level (eg, a congenital arteriovenous malformation) are also obligatory shunts because, even in the fetus, arteriolar pressure far exceeds venous pressure. Thus, the magnitude of the shunt is directly related to the size of the malformation, and the transition from fetus to newborn or newborn to older infant does not alter the magnitude of the shunt. The difference in compliance of the two vascular beds is so great that any increase in ventricular output merely passes into the systemic veins.
Finally, exclusive left-to-right shunts at a pulmonary venous level (eg, partial anomalous pulmonary venous connection) drain into the right atrium or systemic veins. Partial anomalous pulmonary venous return presents similarly to an atrial septal defect, and it may take several months for a clinically significant shunt to develop, if ever.
Atrioventricular septal defects are often termed “endocardial cushion defects” or “atrioventricular canal defects,” but these terms assume an understanding of the embryologic origin, which has yet to be completely defined. The spectrum of defects that make up atrioventricular septal defects, from the primum atrial septal defect and the cleft mitral valve to the complete atrioventricular septal defect, have one common finding: absence of the atrioventricular septum (Figure 7-3). Thus, the term “atrioventricular septal defect” seems most appropriate. The septal leaflet of the tricuspid valve inserts inferiorly to the septal attachments of the mitral valve, and it is the atrioventricular septum that separates the right atrium from the left ventricle. This portion of the septum is very small and is recognized on echocardiography by the more inferior hinge point of the septal leaflet of the tricuspid valve compared to the anterior leaflet of the mitral valve. In all forms of atrioventricular septal defect, this discrepancy is absent, and the two valves lie in the same plane. Because of the absence of the atrioventricular septum, it is possible for blood to shunt directly from the left ventricle to the right atrium. As stated in the preceding discussion, this type of shunt is obligatory and can be quite large. However, the atrioventricular valve tissue usually prevents such a direct connection, and in most cases of atrioventricular septal defects, a left ventricle-to-right atrium shunt is not present.
FIGURE 7-3.
A. Apical four-chamber echocardiogram in a normal newborn infant. The atrioventricular septum (avs) lies between the medial hinge point of the mitral valve (mv) and the more inferiorly placed septal hinge point of the tricuspid valve (tv). B. Apical four-chamber echocardiogram in a newborn infant with a complete atrioventricular canal defect. Note that the right-sided component of the atrioventricular valve (ravv) hinges at the same level as the left-sided component (lavv) so that there is no atrioventricular septum. The absence of an atrioventricular septum occurs even in the presence of a partial atrioventricular canal defect (eg, a primum atrial septal defect) in which there is no ventricular septal defect. Abbreviations: LA, left atrium; LV, left ventricle; RA, right atrium; RV, right ventricle.

A complete atrioventricular septal defect does not usually cause symptoms in the fetal or early neonatal periods. Newborn infants may not have a cardiac murmur because pulmonary vascular resistance is still relatively high. This is an especially important consideration in a newborn with trisomy 21, in whom the likelihood of an atrioventricular septal defect is high. The absence of a murmur is common in these infants because they frequently have lung hypoplasia, which contributes to the maintenance of high pulmonary vascular resistance. Thus, every infant with trisomy 21, even in the absence of a murmur or other signs and symptoms, should have an echocardiogram reviewed by a pediatric cardiologist. Most infants with a complete atrioventricular septal defect present within the first few weeks of life with tachypnea, diaphoresis, poor feeding, and failure to thrive.
On physical examination, the weight percentile is usually at least one or two standard deviations below the length percentile. If the infant has trisomy 21, it is important to use growth charts specific to this condition, which show quite a different normal growth pattern than that of the normal population. There is often moderate resting tachycardia, in the range of 130 to 150 beats per minute. Tachypnea with intercostal and substernal retractions is common. The extremities are warm and well perfused, and the peripheral pulses are strong, indicating that systemic blood flow is well maintained by peripheral vasodilation. Systemic oxygen saturation measured by pulse oximetry may be normal at rest but may be in the low 90s, especially during crying, because of right-to-left shunting at the atrial level. The liver is moderately enlarged with a sharp margin, and splenomegaly can often be appreciated. The precordium is diffusely hyperactive to palpation. The first heart sound is often normal but occasionally may be louder than normal. If the atrial or ventricular-atrial shunt is large and there is a right bundle branch block, the second heart sound may be widely split with little variation. More often, however, the rapid heart rate and high pulmonary arterial diastolic pressures make it difficult to appreciate splitting of the second heart sound. However, the second heart sound is almost always louder than normal because of high diastolic pulmonary arterial pressure. Extra heart sounds are not common, but occasionally an S3 can be heard at the apex. There is often a harsh mid-frequency systolic murmur along the mid-sternal border that may be holosystolic and that radiates throughout the chest. A separate systolic murmur of higher frequency may be appreciated at the apex if there is significant left-sided atrioventricular (mitral) valve insufficiency. This situation should be suspected if the pulses are decreased and the peripheral extremities are cool. A diastolic inflow rumble is often present at the apex or the lower left sternal border, though rapid heart rates may make it difficult to appreciate. More often, the absence of silence in diastole suggests that a diastolic murmur is present. The diastolic murmur is caused by excessive blood flow across the mitral valve and usually indicates that pulmonary blood flow is at least twice the normal.
The chest radiograph shows generalized cardiomegaly with a globular heart, often including a prominent right contour indicative of right atrial enlargement and increased pulmonary vascularity.
The electrocardiogram shows left axis deviation (QRS axis between 0 and –120 degrees) with a counterclockwise loop. Right ventricular hypertrophy is demonstrated by persistence of upright T waves with prominent R waves in the right precordial leads (Figure 7-4). If a large ventricular shunt is present, the electrocardiogram shows biventricular hypertrophy, indicated by the presence of normal left-sided forces despite right ventricular hypertrophy. These left-sided forces are demonstrated by prominent S waves in the right precordial leads as well as left-sided forces (R waves) in V6 and in the inferior limb leads.
The echocardiogram clearly shows the common atrioventricular valve with atrial and ventricular septal defects. The attachments of the valve, the relative size of the two ventricles, and the degree of atrioventricular valve insufficiency are critical elements of the echocardiographic examination. Associated defects commonly occur, particularly secundum atrial septal defects and patent ductus arteriosus. The most important commonly associated defects are subaortic stenosis and coarctation of the aorta because of their deleterious effects on systemic perfusion.
Treatment of heart failure and failure to thrive are important elements of the medical management of infants with atrioventricular septal defects. However, because of the large left-to-right shunt and propensity for the early development of pulmonary vascular disease, it is important to plan for early surgical repair. Infants with complete atrioventricular septal defects generally undergo surgical repair in the second or third month of life, even in the absence of severe heart failure and failure to thrive. Repair in the first few months of life is associated with a more benign postoperative course and fewer problems related to residual pulmonary hypertension. Although several surgical techniques are utilized, the general approach involves closure of the atrial and ventricular septal defects and repair of the atrioventricular valve. Postoperatively, these infants may have residual atrioventricular valve insufficiency (or occasionally atrioventricular valve stenosis). However, the prognosis is favorable, and most infants and children are symptom free after surgical repair.
The most common congenital cardiac structural malformation is a ventricular septal defect. Ventricular septal defects are categorized according to their position within the septum (Figure 7-5). The most common ventricular septal defects occur in the membranous septum, the very small component of the ventricular septum where the aortic, mitral, and tricuspid valves attach and where there is no muscle in the septal wall. These defects extend from the membranous region in any direction and are thus commonly termed “perimembranous ventricular septal defects.” Most commonly, they extend toward the outlet of the ventricles. In this situation, the outlet septum may be normally aligned with the inlet septum, allowing for unobstructed flow of blood from each ventricle to its respective great vessel. Less commonly, the outlet septum may be malaligned with respect to the inlet septum. If there is anterior deviation of the outlet septum, the defect is then subaortic, and it is associated with subpulmonic and pulmonic valve stenosis (tetralogy of Fallot; see Chapter 6). If there is posterior deviation of the outlet septum, the defect is subpulmonic, and there is often associated subaortic stenosis and coarctation of the aorta (Figure 7-6).
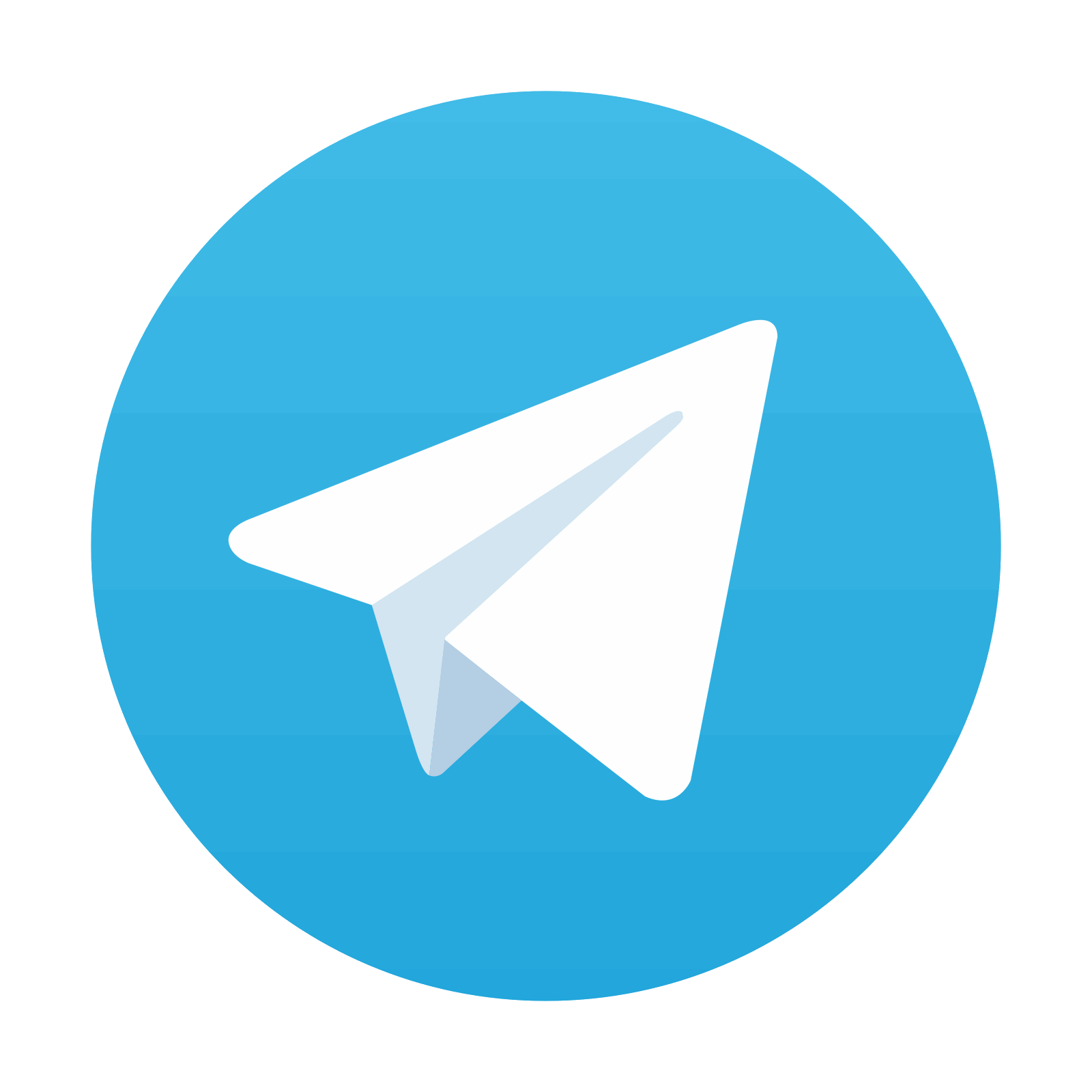
Stay updated, free articles. Join our Telegram channel

Full access? Get Clinical Tree
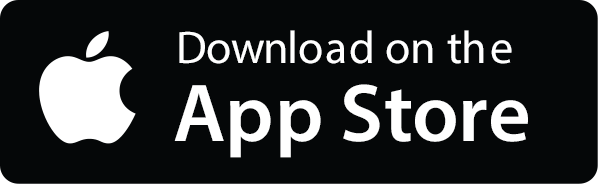
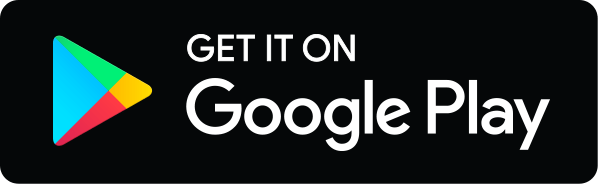