A cartoon of the histology of the initial plaque of aortic stenosis is demonstrated. There is a remarkable similarity to the atherosclerotic plaque of coronary artery disease with a lipid laden core infiltrated with inflammatory cells. Taken form Ref. [1]. From Otto CM, et al. [1]. Reprinted with permission from Wolters Kluwer Health, Inc.
About 1–2% of the US population is born with a bicuspid instead of a tricuspid aortic valve. Possibly because of less favorable hemodynamics causing increased shear stress, bicuspid valves become stenotic a decade or two earlier in life than do tricuspid aortic valves. Or it may be that the NOTCH 2 gene which plays a role in both cusp development and calcification is involved [9].
Rheumatic fever and subsequent rheumatic heart disease are a rare cause of AS in developed countries but are a much more common cause in the developing world. When rheumatic heart disease is the etiology, the mitral valve is almost always involved. These and other rarer causes of AS are listed below.
Causes of aortic stenosis
- 1.
Calcific atherosclerotic disease
- 2.
Rheumatic heart disease
- 3.
Post radiation
- 4.
Carcinoid syndrome
- 5.
Serotonergic drugs: ergotamine, pergolide, cabergoline, fenfluramine
- 6.
Ochronosis
- 7.
Paget’s disease
Bicuspid Aortic Valve and Aortic Root Dilatation
Bicuspid aortic valve may also be associated with aortopathy leading to aortic root or ascending aorta dilatation, more commonly with joined right and left cusps than with other patterns of bicuspidization [10, 11]. Root enlargement occurs in AS but is more prominent in aortic regurgitation. The cause of this dilatation is debated between two major theories [12–14]: (1) bicuspid valve is part of a syndrome associated with a genetic predisposition to aortic root dilatation distinct from other aortic syndromes (Marfan, Loeys Dietz, etc.); (2) abnormal flow exiting the misshapen aortic valve impinges on the aorta, increasing wall stress at the impingement points, causing aortic dilatation [12]. In fact, matrix metalloproteinases (MMPs) appear to be activated in areas of jet impingement supporting the flow hypothesis [12, 14]. However other areas of the aorta not impacted by flow impingement also show root dilatation. It may be that both mechanisms play a role in bicuspid valve-associated aortopathy. A broader discussion of this topic is found in Chap. 4.
Pathophysiology and Its Relationship to Symptoms

(a) The natural history of aortic stenosis from mid-twentieth century is demonstrated. Following a long latent period where survival is nearly normal, the onset of symptoms of angina, syncope and heart failure heralds a dramatic worsening in prognosis. Adopted form Ref. [15]. From Ross JR, Braunwald E [15]. Reprinted with permission from Wolters Kluwer Health, Inc. (b) The natural history of aortic stenosis in the twenty-first century as depicted by Bonow and confirmed by randomized trials finds the same impact of symptoms but with onset 10–20 years later in life owing to a change in etiology of the disease. From Carabello BA [29]. Reprinted with permission from Wolters Kluwer Health, Inc.
Aortic valve area and transvalvular gradient
AVA (cm2) | Mean gradient (mmHg) |
---|---|
1.5 | 11 |
1.2 | 17 |
1.0 | 25 |
0.7 | 51 |
0.5 | 100 |
0.4 | 156 |
The LV response to this pressure overload is the development of concentric hypertrophy (LVH) and/or concentric remodeling. Pressure overload in some way triggers the addition of sarcomeres in parallel so that each myocyte becomes thicker, in turn leading to increased wall thickness, and, if total mass increases, left ventricular hypertrophy (LVH) . In some cases, ventricular geometry changes in such a way that LV radius decreases as wall thickness increases so that there is not true hypertrophy but rather concentric remodeling. Left ventricular hypertrophy is usually viewed as a double-edged sword, with both beneficial and pathologic consequences. Afterload, the force that opposes contraction is often described as wall stress (σ), where σ = P × r/2h and P = systolic pressure, r = LV radius, and h = wall thickness. Increased thickness in the denominator (concentric hypertrophy) offsets increased pressure in the numerator, thus maintaining normal stress (afterload) helping to maintain normal ejection and thus is beneficial [18, 19]. Unfortunately pathologic LVH contributes to the causes of angina, heart failure and syncope. Left ventricular hypertrophy impairs coronary blood flow reserve that normally accompanies the increased oxygen demands of increased stroke work [20, 21]. Impaired reserve may stem from diminished capillary ingrowth insufficient to meet the increase in muscle mass [22]. Reserve is further decreased from increased LV filling pressure due to impaired diastolic relaxation. Increased LV filling pressure reduces the pressure gradient for coronary flow (aortic diastolic pressure minus LV diastolic pressure) especially to the subendocardium, further contributing to potential exercise induced ischemia [21]. Increased wall thickness and increased collagen deposition also impair diastolic filling leading to heart failure symptoms [23–25]. Eventually LVH also causes systolic dysfunction, the exact mechanisms of which are still debated but ischemia, impaired calcium handling, excess afterload, and apoptosis play a role [21, 26–28]. LVH by diminishing LV cavity volume reduces stroke volume and cardiac output contributing to hypotension and syncope during activity.
Evolution of the Natural History of Aortic Stenosis

The heart in aortic stenosis modeled as an electrical circuit where RV = right ventricle, R1 = the pulmonary vascular resistance, LV = left ventricle, R2 = the resistance offered by the stenotic aortic valve and R3 the total peripheral resistance. Earlier detection and treatment of aortic stenosis has caused R2 to lessen over the past 50 years while onset of aortic stenosis later in life occurs when more patients have hypertension increasing the importance of R3. From Carabello BA [31]. Reprinted with permission from Wolters Kluwer Health, Inc.
The Stages of AS and Grading of Severity
Stages of valvular heart disease
Stage | Definition | Description |
---|---|---|
A | At risk | Patients with risk factors for development of VHD |
B | Progressive | Patients with progressive VHD (mild-to-moderate severity and asymptomatic) |
C | Asymptomatic severe | Asymptomatic patients who have the criteria for severe VHD: C1: Asymptomatic patients with severe VHD in whom the left or and right ventricles remains compensated C2: Asymptomatic patients with severe VHD with decompensation of the left or right ventricle |
D | Symptomatic severe | Patients who have developed symptoms as a result of VHD |

Valve area and mean gradient from over 3400 patients with aortic stenosis are plotted against each other. The data fit (yellow line) closely to that predicted by the Gorlin formula (blue line). Importantly in 30% of patients (lower left quadrant) the data are discordant with valve area in the “severe” range while gradient only predicts moderate disease. From Minners J, et al. [33]. Reprinted with permission from Oxford University Press

Endocardial left ventricular shortening (left) and mid-wall shortening (right) are plotted against systolic stress (afterload) for patients with left ventricular hypertrophy . Dotted lines represent the boundaries for normal subjects. Mid-wall shortening that better reflects total myocardial function across the left ventricular wall is low normal or abnormal in aortic stenosis patients, performance not reflected when only examining endocardial events, i.e., ejection fraction. From Baicu CF, et al. [36]. Reprinted with permission from Wolters Kluwer Health, Inc.
Disease Progression
AS is caused by an active inflammatory process and as such is predictably a progressive disease, with the degree of stenosis worsening over time. While the disease is seldom static, the rate of progression is remarkably variable from patient to patient. Jet velocity on average increases 0.2–0.4 m/s/year, mean gradient 7–20 mmHg/year and AVA decreases 0.1–0.2 cm2/year [3, 5, 37–40]. These data are useful in determining the interval between patient follow-up visits. Every patient should be educated to look for the development of the classic symptoms of AS and advised to alert the provider if they occur. However knowledge that the disease is entering the severe phase serves to focus increased scrutiny onto the patient, questioning him/her closely about symptomatic status. To this end, periodic echocardiography helps define the progression of disease specific to the patient being followed. Considering the data noted above, it would be highly unlikely that a patient with mild disease (jet velocity <2.5 m/s) would progress to severe disease in 1–2 years so that echocardiography every 2–3 years should provide sufficient surveillance unless there is a change in symptom status. On the other hand, patients with more advanced disease require more frequent echocardiographic observation, every 1–2 years for jet velocity 2.5–3.0 m/s and yearly for jet velocity >3.0 m/s. The above suggestions presume normal LV stroke volume and must be modified if stroke volume is abnormally low.
Diagnosis
Physical Exam
The diagnosis of AS is often first suspected when a murmur is detected during routine physical examination. Typically the murmur of AS is a raspy systolic ejection murmur that radiates to the neck. In mild disease the murmur peaks in mid-systole creating a crescendo-decrescendo murmur. However as stenosis increases, peak intensity occurs progressively later in systole and peaks at end systole in severe disease. In some cases the murmur is heard loudest in the aortic area, diminishes over the sternum, and is heard loudly again at the LV apex, misleading the examiner to believe that two murmurs (aortic and mitral) are present (Gallavardin’s phenomenon ). The intensity of the murmur is often inversely related to disease severity because in advanced AS reduced stroke volume decreases the loudness of the murmur. Thus a soft late peaking systolic ejection murmur may indicate severe disease. If the patient experiences variation in R–R interval during the exam, either due to premature beats or atrial fibrillation, murmur intensity increases after longer R–R intervals because stroke volume increases. This finding helps distinguish the murmur from that of mitral regurgitation where murmur intensity does not change following longer pauses.

Carotid pulse tracings similar to the pulse felt during physical examination for a normal subject (left) and the delayed pulse of a patient with aortic stenosis (right) is shown. From Carabello BA [41]. Reprinted with permission from Lange Medical Books/McGraw Hill
Imaging
The EKG may exhibit criteria for the presence of LVH and the chest X-ray may demonstrate a boot-shaped heart consistent with concentric LVH but echocardiography forms the mainstay of diagnosis. The 2-D images assess LV function and the extent of LVH. In severe AS the aortic valve is heavily calcified and nearly immobile. If tricuspid valve regurgitation is present, right ventricular and peak pulmonary artery pressure can be estimated and may reflect LV filling pressure. Doppler interrogation of the valve demonstrates acceleration of blood as it passes through the stenotic valve because velocity must increase to maintain flow through a narrowed orifice [flow = area (A) × velocity (V)]. Using the modified Bernoulli equation, the pressure gradient (g) across the valve is calculated as g = 4V 2. Because flow must be equal on either side of the valve (continuity), (A 1 × V 1 = A 2 × V 2). In turn CSA1 × VTI1 = CSA2 × VTI2 so that CSA2 = CSA1 × VTI1/VTI2, where CSA1 = aortic outflow tract (LVOT) cross-sectional area, VTI1 = aortic outflow tract time-velocity integral, VTI2 = aortic valve time-velocity integral, and CSA2 = aortic valve area. Because peak transvalvular jet velocity is directly measured primary data, not relying on calculation form other data, it has become the foundation of AS severity assessment when LV function and stroke volume are normal. However, because stroke volume generates flow velocity, AS severity may be underestimated in low flow states. In these cases, valve area that assesses gradient and velocity in the context of flow assumes greater importance in severity assessment. Obtaining these data is subject to several pitfalls of which the clinician must be aware.
Challenges and Pitfalls
- 1.
The maximum aortic velocity and the mean gradient can be grossly underestimated if the continuous Doppler beam is not aligned parallel or near parallel to the stenotic jet. As such the insonification angle between the Doppler beam and the jet should be maintained at less than 30°. However the stenotic jet can be eccentric and the aorta can be unwound making this technically challenging (Fig. 5.7). In such cases, a transesophageal echocardiogram may help to obtain the maximum velocities by careful manipulation of the probe using multiple planes (Figs. 5.8, 5.9, and 5.10).

Continuous wave Doppler of the aortic stenosis jet in a patient with uncontrolled chronic systemic hypertension resulting in an unwound aorta. This anatomy constrains the ability to obtain the best Doppler angle of insonification resulting in underestimation of the maximum velocities. The mean gradient appears to be 28 mmHg and the maximum velocity is estimated to be 3.5 m/s

In this case the eccentric aortic stenosis jet causes the inability to align the Doppler beam to obtain the maximum aortic velocity as shown below

Note the faint spectral Doppler envelopes from the deep trans gastric TEE views. The Doppler envelope is poorly defined giving an unreliable estimate the mean gradient and the peak velocity is underestimated

In the same patient withdrawal of the probe to a mid-esophageal location and sampling the flow with continuous Doppler yields a higher aortic velocity with a dense spectral envelope. This case illustrates the importance of using multiple planes and views to obtain the highest velocities, avoiding underestimation of the severity of aortic stenosis. The true mean gradient was 45 mmHg and the maximum velocity was 4.5 m/s
Challenges When Using the Continuity Equation for Assessment of Aortic Stenosis
Based on the principle of conservation of mass, the aortic valve area is derived from the continuity equation noted above. Deriving the area of the LVOT is crucial to the accuracy of the calculation.

Thus, if the diameter of the LVOT is not measured diligently using multiple attempts and in “ZOOM” view, overestimation or underestimation of the severity of aortic stenosis may occur. This is by far the most common reason for underestimating aortic valve area.
Technique for Proper Measurement of LVOT Diameter
- 1.
Always use the “ZOOMED” view and acquire a cine loop in the left parasternal long axis view.
Measure at the “hinge points’—inner edge to inner edge in mid-systole as shown in Fig. 5.11.
In the left PLAX view, the lateral resolution is better and the risk of underestimating the LVOT diameter is reduced. Moreover the left PLAX view offers axial resolution which is always better than lateral resolution in echocardiography. The measurement should be made in mid-systole as there conformational change in the aortic root during the cardiac cycle and the annulus assumes a more circular shape during this phase.
- 2.
Always measure at the “hinge points” where the cuspal insertion is noted and, in a plane, parallel to the LVOT (Fig. 5.12). A parallel view gives a more accurate measurement than an oblique view. If the measurement is made from the apparent (virtual) annulus as shown in Fig. 5.13, it may overestimate the LVOT dimension.
- 3.
Once a parallel view is confirmed, rotate/angulate the transducer till the largest diameter of the LVOT is obtained. This can be noted by dissecting the plane through the right coronary cusp anteriorly and the interleaflet triangle between the left coronary and the noncoronary cusp posteriorly. If the t wo leaflets are visualized anteriorly and posteriorly, the largest diameter has not been obtained (Figs. 5.14 and 5.15).
- 4.
Morphological changes in the subaortic location that may hinder accurate measurement of the LVOT diameter such as the bulky calcium protrusion shown in Fig. 5.16 but can be excluded for greater accuracy as shown in Fig. 5.17.
- 5.
Septal hypertrophy . Septal hypertrophy is frequently seen in patients with aortic stenosis (Fig. 5.18). Care should be taken to identify the aortic annulus and measurements should be done at proper location so that the diameter is not underestimated. This can be corrected by using the zoomed view and careful alignment parallel to the LVOT (Fig. 5.19).
- 6.
Technical limitations
- (a)
Body habitus
Poor acoustic windows in a patient with COPD or morbid obesity, the aortic root may not be well visualized. Alternate methods of imaging such as TEE can be used for estimation of the LVOT diameter.
- (b)
Acoustic shadowing from calcified aortic valve and aortic root as shown in Fig. 5.20 may cause inaccurate measurement of the LVOT.
- (c)
Diligent attention must be paid to the gain and the use of focalization to improve image optimization.
- (a)

The “zoomed” view for proper measurement of the aortic annulus is shown

TEE study with measurement taken from the “hinge points” between the cusps at the “virtual annulus” estimates the diameter of the LVOT at 2.2 cm. The arrow points to the cuspal separation during systole

TEE study with measurement taken from the commissure between the left and noncoronary cusp to the right cusp at the “virtual annulus” overestimates the diameter of the LVOT at 2.4 cm

The right coronary cusp (RCC) and the posterior cusp are both well-defined and the LVOT dimension measures 2.2 cm

The RCC is well visualized but the posterior RCC is not well visualized and the LVOT dimension is overestimated at 2.4 cm

A bulky calcium deposit (arrow) blocks proper annulus measurement

Using biplane TEE , the mass seen in Fig. 5.16 was excluded, the “hinge points” were clearly defined and the annular size is more accurately estimated at 2.2 cm

The green line indicates septal wall thickness measured at 1.5 cm. The blue line indicates LVOT measurement which has been underestimated at 1.9 cm

Using zoomed views from the case noted in Fig. 5.18 and more careful alignment in parallel to the LVOT, the measurement of the AV annulus is now 2.2 cm

The arrow points to the acoustic shadowing from the subaortic calcification that results in poor definition of the aortic valve annulus
Other Imaging Modalities
Because calcification is a key bodily response to tissue damage, it is logical that the degree of calcification of the aortic valve would correlate with AS severity. Indeed valve calcification measured using multidetector CT scanning correlates with valve area [42–44]. Thus valve calcification may be a useful adjunct in deciding AS severity in borderline cases or when standard measures of assessment are discordant with one another.
Cardiac Catheterization
Prior to the advances made in echocardiography, invasive hemodynamic evaluation was the gold standard for AS severity assessment. Both noninvasive and invasive techniques use the same principle to define valve area (A) where A = F/V and F = flow and V = flow velocity. During echocardiography , velocity is measured directly. Invasively, velocity is calculated using Torricelli’s equation v = 2√gh, where g is the velocity due to gravity (converting force to mmHg) and h = the mean pressure gradient. Valve area is determined by the Gorlin formula [45], AVA = F/2√gh where flow is determined by thermodilution or Fick methods and the gradient obtained by directly measuring pressure proximal (left ventricle) and distal (aorta) to the aortic valve. Invasive technique is used when there are discrepancies between the clinical presentation and echocardiography or when body habitus precludes obtaining clear echocardiographic data. Thus when invasive AVA determination is made, it is assumed that the data must be of the highest quality in order to resolve the diagnostic dilemma at hand. As such there must be fastidious attention paid to pressure recording and cardiac output determination. Pulse delay makes recording from the femoral artery inaccurate and should not be used. Cardiac output today is often made using an assumed value for oxygen consumption in the Fick equation potentially leading to large errors in its determination [46] and as a consequence large errors in calculating valve area.
Medical Therapy
Aortic stenosis is a mechanical problem with only a mechanical solution, i.e., aortic valve replacement. No medical therapy has been demonstrated to alter the natural history of the disease. Because most patients today are elderly and because the incidence of hypertension increases with age, many AS patients also have systemic hypertension. There is a natural concern that antihypertensive agents that cause vasodilatation might lead to hypotension because fixed valve obstruction to flow could impair cardiac output. Thus any antihypertensive agent should be used on a “start low and go slow” basis. However there are several reports of the use of vasodilators to treat both hypertension and acute heart failure in AS [47–50], although no antihypertensive regimen has been shown superior in the treatment of AS patients with hypertension. If heart failure has intervened and AVR is contraindicated because of the presence of severe comorbidities, diuretics can be used to relieve symptoms. Because standard heart failure therapy has not been tested in AS in any large randomized trial, there is no evidence that such therapy would be beneficial nor is there any defined mechanism by which heart failure therapy might prolong life in AS.
Indications for Surgery
Symptomatic AS

Life expectancy (LE) for British Columbia (BC), for the United Kingdom (UK), and the United States (US) is plotted for normal subjects and those with bioprosthetic aortic valves (BP), and patients with mechanical prosthetic valves (MP) . On average, normal 50-year-old patients are expected to live another 28 years while patients with valve prostheses are projected to live only 18 years longer. The difference narrows with age, in part because death is inevitable and in part because the prosthesis is present for fewer years. From van Geldorp MWA, et al. [51]. Reprinted with permission from Elsevier
Asymptomatic Severe AS

Life expectancy is shown for a normal matched population and for asymptomatic patients with severe aortic stenosis censored at the onset of symptoms (a) or when valve replacement was performed (b). Asymptomatic patients have an excellent prognosis as indicted in Fig. 5.2a. However the worse prognosis when assessed at aortic valve replacement (b) suggests that there is delay from symptom onset to definitive therapy with patients dying in the interim. From Pellikka PA, et al. [52]. Reprinted with permission from Wolters Kluwer Health, Inc.

Prognosis in severe aortic stenosis is stratified according to results of exercise testing. The difference in event-free survival between patients with a negative versus positive exercise test is striking. From Amato MC, et al. [54]. Reprinted with permission from BMJ Publishing Group Ltd.

The impact of transaortic jet velocity on patients with aortic stenosis is demonstrated. The higher the jet velocity the more likely that an event (symptoms or death) will occur with jet velocity exceeding 5 m/s being particularly ominous. From Rosenhek R, et al. [55]. Reprinted with permission from Wolters Kluwer Health, Inc.

Prognosis in patients with severe aortic stenosis according to brain type natriuretic hormone is demonstrated. Patients with a BNP/normal ratio of >2 (b) is compared to that of patients with a ratio <2 (a) for patients treated surgically (black line) or “medically” (red line). Patients with high BNP fared worse with both types of therapies. From Clavel MA, et al. [61]. Reprinted with permission from Elsevier
An issue often arises concerning the aortic valve management of patients with only moderate AS (and asymptomatic from the AS itself) who are undergoing cardiac surgery addressing another cardiac issue, usually coronary revascularization. As noted above, AS is a progressive disease. Bypass surgery leaving moderate AS untreated could result in symptomatic AS developing in 2–3 years, necessitating AVR during a second operation. While TAVR might be reasonable in that situation, currently, most heart teams would elect to perform AVR at the time of bypass surgery in patients with moderate AS. While there are no randomized trials addressing this issue, data support better long-term outcomes when AVR accompanies such operations [62].
Of note a recent study of propensity matched pairs demonstrated benefit for patients undergoing AVR prior to symptom onset [63]. However almost two-thirds of these patients had one of the indications noted above for undergoing early AVR and over 30 patients who developed symptoms did not undergo AVR. The issue may be settled by the results of an ongoing randomized trial comparing TAVR to observation in asymptomatic patients with severe AS (Edwards Early TAVR Trial).
Mechanical Therapy: SAVR and TAVR
Valve replacement is the only definitive therapy for AS and can be accomplished either at cardiac surgery (SAVR ) or through transcatheter techniques (TAVR). The decision regarding which approach is best for a given patient is decided by the Heart Team composed of structural cardiologists, general cardiologists, imagers, heart surgeons, anesthesiologists and most importantly the patient (TAVR is covered extensively in Chap. 10). The decision will in part be based upon LV function, the need for other cardiac procedures, comorbidities, surgical history, age, patient preference, social considerations and the risk calculated from the STS database. As an example of the factors involved in decision-making, consider an 80-year-old woman who is healthy except for aortic stenosis but uses a walker for mobility due to severe knee and hip problems and has no family or other local support. Although her mortality is low for SAVR , a TAVR might be recommended because the patient would have trouble adhering to sternal precautions post-operatively and bed rest postoperatively might worsen her immobility. On the other hand, a patient with a high predicted mortality from AVR but who also has severe coronary disease not amenable to percutaneous intervention might be recommended for a surgical valve so that the associated coronary disease can be addressed at the time of surgery.
Surgical Therapy (SAVR)
Aortic Valve Anatomy for the Surgeon: Avoiding Injury to Vital Structures
The aortic valve is usually trileaflet and the leaflets are recognized by their relationship to the coronary arteries: left, right, and noncoronary. The noncoronary leaflet abuts the anterior leaflet of the mitral valve. The Bundle of His lies adjacent to the commissure between the right and the noncoronary cusp. An aortic valve annular stitch that inadvertently sews into mitral tissue can cause mitral dysfunction postoperatively. If calcium is debrided too deeply, heart block can result from direct injury to the His Bundle; if not enough calcium is debrided from the annulus, compression of the His bundle may also cause heart block.
Type of Valve Replacement: Mechanical vs. Bioprosthetic
Once a decision has been made that a patient should undergo surgical aortic valve replacement, the type of replacement valve must be addressed. For most patients the two options are either a bioprosthetic or mechanical valve. Bioprosthetic valves are made from precisely cut pieces of bovine or porcine pericardium although some are actual pig allograft aortic valves secured inside a reinforced fabric suture ring. Human homografts are still used in some centers but difficulty in obtaining them reduces their usefulness. While enhanced durability was expected from homografts, it is not clear that homografts are more durable than other tissue valves. Mechanical valves are made of pyrolytic carbon, a graphite material that has extremely smooth surfaces and are designed with “washing jets” that prevent clot formation on the hinges. The valves are so smooth that metal instruments touching the valve during the operation damage them; instead, plastic tools must be used to manipulate mechanical valves.
The strengths and weaknesses of each valve type are well known and well summarized [64]. Mechanical valves require vitamin K antagonist (VKA) anticoagulation to reduce the risk of thromboembolism while tissue valves carry the risk of structural deterioration requiring reoperation. Mechanical valves generally function well for the lifetime of the patient although they are not entirely free of structural failure because valve obstruction can occur from thrombus or pannus ingrowth of fibrous tissue. This risk is low, probably less than 1% per year [65]. Unfortunately the risk of major bleeding from anticoagulation is 1–2% per year. A patient receiving a mechanical valve in his/her 20s carries a 50–80% lifetime risk of bleeding or thromboembolic complications [64]. In some cases the clicking sound of mechanical valves is so bothersome to the patient that the valve may have to be removed. Tissue valves on the other hand require no anticoagulation. The risk of deterioration varies according to the age of the patient at implantation. In patients older than 70, 95% of tissue valves function well at 10 years and 90% function well at 15 years. In patients younger than 50, 50% of bioprostheses deteriorate in 5–10 years [64]. It is unclear why bioprosthetic valves deteriorate more rapidly in younger patients, but it is thought that it might be due to differences in calcium metabolism. Bioprosthetic deterioration is accelerated in patients on dialysis [66].
Older age, bleeding risk (history of bleeding, exposure to trauma in the workplace, frequent falls) and patient preference for avoiding anticoagulation favor bioprosthetic AVR and avoidance of anticoagulation. Young age and a second disease requiring anticoagulation such as atrial fibrillation favor AVR with a mechanical valve. While it had been hoped that newer non VKA anticoagulants might make anticoagulation easier and thus make mechanical valves more attractive, this hope has not yet been realized [67].
Aortic Valve Autografts: The Ross Procedure
In the Ross procedure the patient’s pulmonary valve is transplanted into the aortic position while a homograft replaces the pulmonic valve. The principle of the Ross procedure is that the native pulmonic valve functions durably in the aortic position while the homograft has enhanced durability (compared to placement in the aortic position) in the low-pressure pulmonary position. This procedure is advantageous in young patients who wish to avoid the complications of anticoagulation from mechanical valves or the almost certain need for reoperation for standard bioprosthetic valves . Freedom from reintervention for the Ross procedure in properly selected patients can be comparable long term to that for mechanical aortic valves. Reoperation rate on the autograft in adults is 1.0% per year and on the homograft 0.65% per year [68]. In a randomized trial, the Ross procedure was superior to homograft AVR [69]. The Ross procedure is a technically demanding operation that has generated controversy and a wide disparity in outcome. It trades disease of one valve for potential disease in two. Candidates for the Ross should be referred to surgeons who specifically specialize in the operation and who have outcome records that prove success.
Operative Planning
Predicting Valve Size
Preoperative planning is integral to the success of the procedure and many issues must be considered prior surgery. Data needed for preoperative planning is obtained largely through preoperative imaging at echocardiography and cardiac catheterization. Standard bioprostheses and mechanical valves are inherently stenotic; while they have a larger effective orifice area than the diseased valve being removed, their orifice area is still smaller than a normal native valve. Patient-prosthetic mismatch wherein the replaced valve is too small for the cardiac demands of the patient in which it is placed, worsens postoperative outcome [70]. Thus every effort is made to insert the largest valve possible. Several factors affect this practice. Valve size can be estimated preoperatively from echocardiographic or CT images of the aortic annulus and outflow tract. Sizing will be confirmed at surgery but it is helpful to predict sizing beforehand. General practice is to insert at least a 23 mm valve which will help avoid patient prosthetic mismatch and will facilitate valve-in-valve TVAR later if the SAVR valve fails (see Chap. 10). If the aortic annulus is small, root enlargement may be necessary which complicates the procedure and increases cross-clamp ischemic time. Generally 2.5 h of cross clamp time with the heart arrested and ischemic is well tolerated by patients with preserved cardiac function. In this context, root enlargement takes about 30 min, the aortic valve replacement itself takes an hour, each bypass graft takes an additional 15 min or longer if using arterial grafts or if the targets are difficult. A maze procedure takes 30 min. Patients with a low ejection fraction or pulmonary hypertension tolerate long clamp times poorly occasionally necessitating mechanical support postoperatively.
Planning for Myocardial Protection
Left ventricular hypertrophy . Patients with aortic stenosis have LVH that may require greater doses of cardioplegia to cool and arrest the heart and may require multiple attempts at defibrillation when the heart is reperfused after the aortic cross clamp is removed.
Right heart protection. Cardioplegia can be administered both antegrade into the coronary arteries and retrograde through the coronary sinus. Retrograde cardioplegia is very effective for the left ventricle but less so for the right. Protecting the right ventricle (RV) is further complicated because the right ventricle is more subject to warming due to room light, ambient air, and blood returning to the right atrium. Protecting the right ventricle is made more difficult when the right coronary is stenotic or occluded as demonstrated by the preoperative coronary arteriogram. In a patient with a chronically occluded right coronary, also undergoing coronary grafting, an effective strategy is to perform the right coronary distal anastomosis immediately and then administer cardioplegia into the vein graft. Right heart protection is even more of a concern if the patient suffers from preoperative RV dysfunction. Patients with RV dysfunction may require epinephrine and milrinone during separation from cardiopulmonary bypass and these drugs are often administered before weaning from cardiopulmonary bypass in such cases, an eventuality that can be anticipated preoperatively.
Aortic regurgitation. If greater than mild aortic regurgitation is present, the heart may not arrest effectively when antegrade cardioplegia is administered to the coronaries indirectly through the aortic root. In this case, a retrograde cardioplegia cannula is placed into the coronary sinus and cardioplegia is administered to arrest the heart immediately. Next, after aortotomy, cardioplegia is delivered directly into the coronary ostia. Thus the presence and severity of aortic regurgitation must be assessed preoperatively during echocardiography.
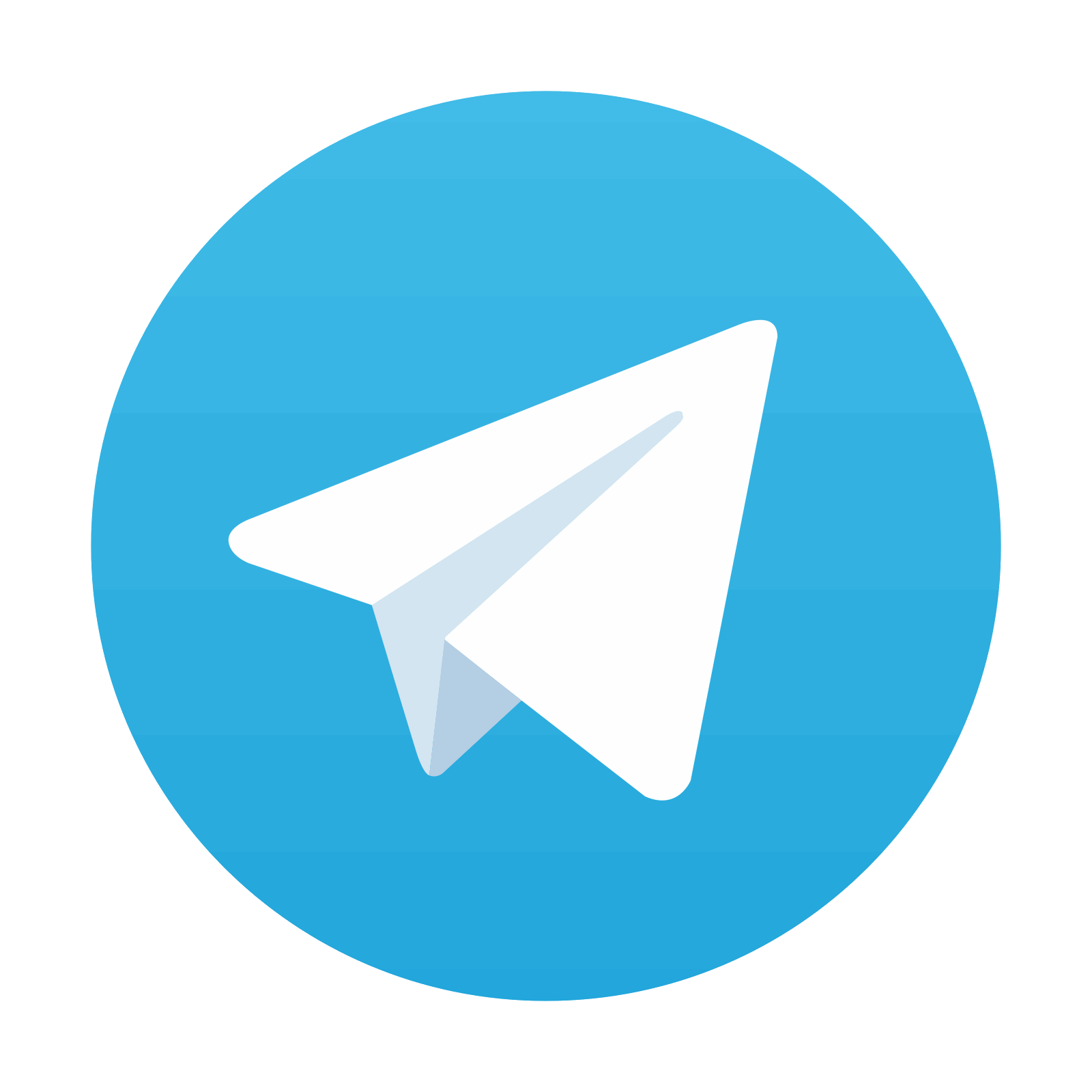
Stay updated, free articles. Join our Telegram channel

Full access? Get Clinical Tree
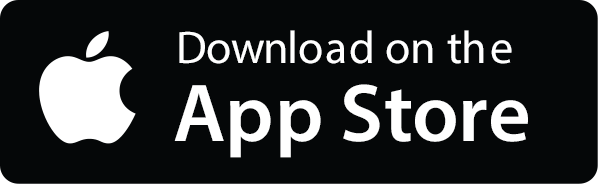
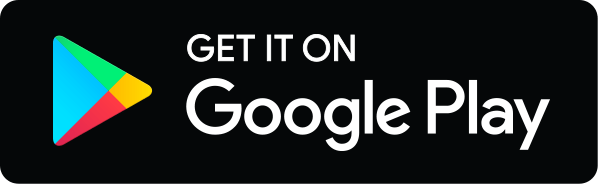