Clinical decisions in patients with aortic valve disease depend on an accurate estimation of the severity of the valve lesion and an understanding of the spectrum of hemodynamic derangements associated with the disorder. Echocardiography serves as the primary imaging modality for this purpose. In the current era, cardiac catheterization is generally employed as an adjunctive method to answer specific questions in patients with aortic regurgitation and aortic stenosis, such as disease severity if this is unclear on noninvasive testing or if there is discordance between symptoms and disease severity determined by echocardiography. In addition, with the advent of transcatheter aortic valve replacement (TAVR), invasive hemodynamic assessment of aortic valve disease has achieved greater importance in the preprocedural and postprocedural phases.
The cardiologist needs to understand the numerous potential sources of error when assessing the aortic valve. The adage “Bad data are worse than no data at all” is particularly relevant for aortic stenosis, in which the errors in estimating the stenosis severity may lead to an entirely wrong conclusion regarding the need for valve replacement. This chapter reviews the hemodynamic features of aortic regurgitation and aortic stenosis, potential errors in data collection and interpretation, and the hemodynamics observed with transcatheter valve interventions.
AORTIC VALVE REGURGITATION
Regurgitation of the aortic valve may be caused by a variety of conditions ( Box 6.1 ). Currently, in the United States, aortic regurgitation is most commonly due to aortoannular ectasia from hypertension or from a congenitally bicuspid aortic valve. Endocarditis is another frequently seen cause, and structural deterioration of an aged bioprosthetic aortic valve is also common. A mechanical aortic valve may result in regurgitation if thrombosis of the prosthesis occurs and results in a leaflet stuck in an open position.
- 1.
Dilatation of the ascending aorta (aortoannular ectasia)
- 2.
Prolapse or incomplete closure of a congenitally bicuspid valve
- 3.
Endocarditis
- 4.
Aortic prosthetic valve failure
- 5.
Rheumatic valvular disease
- 6.
Ankylosing spondylitis
- 7.
Rheumatoid arthritis
- 8.
Ehlers-Danlos syndrome
- 9.
Marfan disease
- 10.
Syphilis
- 11.
Aortic arch aneurysm
- 12.
Aortic dissection
- 13.
Ventricular septal defect (prolapsing cusp)
- 14.
Subaortic membrane
The pathophysiology and hemodynamic abnormalities observed in aortic regurgitation depend on several variables, including the severity of the regurgitation, whether regurgitation is acute or chronic, and the compensatory response to volume overload intrinsic to this lesion.
Chronic Versus Acute Aortic Regurgitation
Hemodynamic consequences of severe aortic insufficiency depend on whether regurgitation is acute or chronic. In chronic aortic regurgitation, the gradual onset of progressive valve regurgitation leads to compensatory changes, allowing a prolonged state of adaptation and a clinically asymptomatic state. Progressive left ventricular dilatation ensues with stroke volume increasing to maintain forward flow. Both end-diastolic and end-systolic volumes increase, maintaining ejection fraction. With enlargement of the left ventricular chamber, ventricular wall thickness must increase to maintain normal wall stress, as dictated by the law of Laplace, which states that the wall stress is proportional to the product of transmural pressure and radius divided by wall thickness. Traditionally, chronic aortic regurgitation has been considered an example of pure chronic volume overload. However, systolic pressure also rises in association with the augmented stroke volume; thus in chronic severe aortic regurgitation, the left ventricle is both volume and pressure overloaded with compensation consisting of both ventricular dilatation and hypertrophy. Chronic aortic regurgitation exceeds other pathological conditions that cause ventricular enlargement and results in the biggest hearts observed clinically (termed cor bovinum , Latin for cow heart). Eventually, however, this compensation is exhausted and contractility diminishes, ejection fraction falls, and decompensation occurs, leading to symptoms of heart failure.
The physiologic benefits of vasodilators in chronic severe aortic regurgitation relate primarily to improved left ventricular function and diminished afterload, especially when systolic hypertension is present. Vasodilator agents do not reduce the regurgitant volume, unless associated diastolic hypertension is present, because the amount of regurgitation is based on both the regurgitant orifice area (unaffected by vasodilators) and the mean gradient between the aorta and the left ventricle during diastole. Because aortic diastolic pressures are already low, vasodilators cannot diminish this gradient further without compromising coronary blood flow.
Many of the interesting physical examination findings of chronic severe aortic regurgitation parallel the hemodynamic findings and are consequences of the compensatory mechanisms that reflect primarily the increased left ventricular size and stroke volume. These include a wide arterial pulse pressure that may exceed 100 mm Hg, a carotid “shudder” from the increased stroke volume, the presence of a diffuse and hyperdynamic apical impulse with lateral displacement, and numerous named signs for various peripheral manifestations ( Table 6.1 ). Auscultation of the heart sounds reveals a soft or absent aortic component of the second heart sound and a characteristic decrescendo diastolic murmur. In chronic compensated aortic regurgitation, the severity of regurgitation correlates with the duration rather than the intensity of the murmur; the murmur is holodiastolic in severe aortic regurgitation and is heard only in early diastole with mild aortic regurgitation. A systolic ejection murmur reflects the increased stroke volume. In some cases of chronic severe aortic regurgitation, the regurgitant stream strikes the mitral valve, and the elevations of the left ventricular diastolic pressure may cause early and partial closure of the mitral valve, resulting in a functionally stenotic mitral valve with an associated diastolic rumble (the Austin Flint murmur).
FEATURE | FINDING |
---|---|
Hill sign | Systolic pressure in the popliteal artery exceeds the pressure in the brachial artery |
Corrigan pulse de Musset sign | “Water hammer” or collapsing pulse; visible arterial bounding pulse with quick upstroke in the carotids |
Quincke sign | Head bobbing |
Müller sign | Visible capillary pulsations of the base of the nail beds |
Traube sign | Pulsations of the uvula |
Duroziez sign | Loud systolic sounds (“pistol shot”) over the femoral arteries |
Systolic murmur heard over the femorals with proximal compression of the artery by a stethoscope; diastolic murmur when compressed distally |
Acute aortic regurgitation behaves entirely different. With the development of acute severe aortic regurgition, none of the compensatory mechanisms involved in the adaptation of chronic severe regurgitation, such as progressive left ventricular dilatation and hypertrophy, are possible. Instead, severe sudden onset of aortic regurgitation causes a dramatic rise in the left ventricular diastolic pressure. The inability to augment the stroke volume because of a normal ventricular cavity size causes a profound and life-threatening decrease in forward flow. The only possible compensatory mechanism in the setting of acute severe aortic regurgitation is tachycardia.
Patients with acute aortic regurgitation are critically ill with respiratory failure from pulmonary edema, hypotension, and shock from diminished cardiac output and a compensatory tachycardia. Interestingly, the diagnosis of acute aortic regurgitation may be difficult because the diastolic murmur is typically early and soft in acute aortic insufficiency and may be completely obscured by extensive pulmonary rales from the associated pulmonary edema. The absence of the compensatory mechanisms described previously prevents the development of the classic peripheral signs of chronic severe aortic regurgitation (i.e., wide pulse pressure, Duroziez sign). For these reasons, prompt diagnosis depends on heightened clinical suspicion for the condition; acute aortic regurgitation should always be considered by the clinician faced with an acutely ill patient with pulmonary edema and hypotension of unclear etiology.
Hemodynamic Findings
In chronic well-compensated aortic regurgitation, the hemodynamic findings typically reflect the associated marked increases in the left ventricular volume and stroke volume. The central aortic pressure waveform is characterized by high systolic pressure, low diastolic pressure, and, consequently, a wide pulse pressure ( Fig. 6.1 ). The marked increase in the stroke volume forms the basis of several hemodynamic findings. Increased systolic flow across an abnormal valve leads to turbulence and a prominent anacrotic notch during systole on the central aortic waveform ( Fig. 6.2 ). The normal physiologic phenomenon of peripheral amplification is even further exaggerated, yielding systolic pressures in the femoral artery greatly exceeding the central aortic pressures ( Fig. 6.3 ). This phenomenon accounts for many of the peripheral signs of aortic regurgitation described earlier.



Additional hemodynamic findings reflect the left ventricular function and the state of compensation. Asymptomatic patients with chronic severe aortic regurgitation whose ventricular function remains normal and who have achieved excellent compensation often exhibit normal or mildly abnormal, resting hemodynamics, except for a wide aortic pulse pressure and marked peripheral amplification. Left ventricular end-diastolic pressure (LVEDP) remains low with right-sided pressures unaffected. With the development of symptoms of left ventricular dysfunction, the left ventricular diastolic pressure rises. Typically, early diastolic pressure is normal and then rapidly rises by end diastole. The aortic and ventricular diastolic pressures may become equal in late diastole, a phenomenon known as diastasis ( Fig. 6.4 ). The point at which diastasis occurs defines the end of the diastolic murmur; at this phase of the cardiac cycle, flow no longer occurs between the aorta and the left ventricle. This fact accounts for the shortening of the murmur with decompensation and the associated rise in the left ventricular diastolic pressure. The degree of elevation of the LVEDP with decompensation varies widely; values more than 50 mm Hg are not unheard of ( Fig. 6.5 ). With the development of decompensation, chronic aortic regurgitation may lead to elevations in right-sided pressures typically associated with heart failure.


Chronic severe aortic regurgitation may elevate the right ventricular end-diastolic pressure in the absence of elevation of the pulmonary capillary wedge pressure or the pulmonary artery systolic pressure. This has been explained on the basis of the Bernheim effect, in which the increased left ventricular volume and the elevations in left ventricular diastolic pressures are transmitted to the right ventricle, thus elevating the right ventricular pressures.
With chronic aortic regurgitation, the low aortic diastolic pressure may adversely affect coronary perfusion. The combination of decreased coronary perfusion coupled with increased demand from greater myocardial mass may lead to ischemia from supply-versus-demand mismatch. Importantly, other causes of a wide pulse pressure exist besides chronic severe aortic regurgitation ( Fig. 6.6 ). They include marked bradycardia, severe systolic hypertension, the presence of a rigid and inelastic aorta as often seen in the elderly or in patients with vascular disease, and the presence of a high-output state as observed in patients with severe anemia, hyperthyroidism, anxiety, significant arteriovenous fistulas, or a large patent ductus.

The hemodynamic findings of acute aortic regurgitation reflect the physiologic effects of acute volume overload and diminished forward flow. The left ventricular diastolic pressure increases with a rapidly rising slope, obscuring the a wave and culminating in marked elevation of the LVEDP. Diastasis is commonly seen. By mid or late diastole, the left ventricular diastolic pressure may exceed the left atrial pressure, resulting in early closure of the mitral valve ( Fig. 6.7 ). Pulmonary edema and subsequent elevations in right-sided pressures ensue with associated hypotension and low cardiac output from diminished forward flow, despite a compensatory tachycardia.

An unusual but interesting finding may be present on the aortic pressure trace in acute aortic regurgitation. In the setting of acute severe aortic regurgitation, premature diastolic opening of the aortic valve may occur from marked elevations in the left ventricular diastolic pressure. With a prematurely opened aortic valve, the additional increase in the left ventricular pressure from atrial systole may transmit to the aortic pressure wave, inscribing an a wave on the aortic waveform ( Fig. 6.8 ). This rare finding is highly sensitive for acute aortic regurgitation.

Angiography in Aortic Regurgitation
Grading the severity of aortic regurgitation in the cardiac catheterization laboratory is based on angiography and not hemodynamics. The hemodynamic abnormalities described provide information regarding the physiologic consequences of aortic regurgitation and the extent of compensation. Nevertheless, when performing cardiac catheterization on patients with aortic regurgitation, both angiography and hemodynamics are important and provide valuable complementary information to the clinician regarding this valvular lesion. Echocardiographic methods of grading aortic regurgitation have been extensively discussed elsewhere.
Grading the severity of aortic regurgitation is based on contrast aortography of the ascending aorta, using a pigtail catheter positioned carefully above the aortic valve. Optimal opacification usually requires about 60 mL of iodinated contrast delivered rapidly (flow rate of 30 mL/s). A commonly used semiquantitative scale for grading aortic regurgitation is shown in Box 6.2 . The degree of regurgitation can also be quantitated by determining the regurgitant volume, which is simply a comparison of the angiographically determined stroke volume with the forward stroke volume determined by the Fick or thermodilution method, as shown by the formula:
- 1.
1+ Small amount of contrast in the left ventricle during diastole that clears with each beat and never completely fills the left ventricular chamber
- 2.
2+ Faint opacification of the entire left ventricle
- 3.
3+ Dense opacification of the left ventricle (as dense as the aorta)
- 4.
4+ Complete and dense opacification of the left ventricle during the first cardiac cycle, and the left ventricle is more densely opacified than the aorta
The angiographic stroke volume is estimated from the ventricular volumes obtained by angiography and is equal to the difference between end-diastolic volume and end-systolic volume. The forward stroke volume is calculated by dividing the cardiac output (determined by either the Fick or thermodilution method) by the heart rate. A regurgitant fraction of 0% to 20% represents mild aortic regurgitation, 20% to 40% represents moderate aortic regurgitation, and greater than 40% indicates severe aortic regurgitation. This method is rarely used clinically in the current era.
AORTIC STENOSIS
Aortic valve stenosis is one of the most common valvular lesions in clinical practice. Adult patients who present with aortic stenosis at relatively younger ages (i.e., younger than 65 years) often have a congenitally bicuspid valve, whereas patients who present older than this age have calcific, tricuspid aortic valves (termed calcific aortic stenosis or senile aortic stenosis ). Other disease processes rarely cause aortic valvular stenosis and include rheumatic heart disease, radiation-induced valvulitis, Paget disease of bone, and end-stage renal failure. It is important to distinguish aortic valvular stenosis from related conditions causing obstruction to left ventricular outflow, such as hypertrophic obstructive cardiomyopathy, subvalvular membranes, supravalvular aortic stenosis, or coarctation of the aorta.
Physiology of Aortic Valve Stenosis
The presence of a pressure gradient across the aortic valve defines aortic valvular stenosis. Normally, a small pressure gradient is present very early in systole when simultaneous left ventricular and aortic pressures are measured with sensitive, high-fidelity catheter-tipped micromanometers that represent an impulse gradient during the rapid phase of ventricular ejection. The pathological gradient of aortic stenosis persists through systole. Progressive obstruction leads to pressure overload of the left ventricle and compensatory left ventricular hypertrophy with associated diastolic abnormalities from increased myocardial stiffness and reduced left ventricular compliance.
Aortic stenosis is progressive. The rate of progression is variable for any individual, but the annual increase in pressure gradient averages 7 mm Hg, and the annual decrease in aortic valve area (AVA) averages 0.1 cm 2 . Patients with aortic stenosis remain asymptomatic for prolonged periods until valve stenosis is severe. Symptoms include chest pain, syncope, and dyspnea. Interestingly, the severity of stenosis alone does not necessarily correlate with symptom status or the nature of symptoms. A study of patients with severe aortic stenosis and preserved ejection fraction found that the extent of obstruction (based on the mean pressure gradient and AVA) was the same regardless of the nature of symptoms (e.g., asymptomatic, syncope, chest pain, or dyspnea). However, patients with syncope had smaller left ventricular cavities and reduced cardiac outputs, and patients with dyspnea had more profound diastolic abnormalities. The cause of angina chest pain is primarily from increased wall stress and subendocardial ischemia from coronary blood flow supply-demand mismatch, although coronary artery disease is commonly present in this population as well. Sudden cardiac death occurs primarily in symptomatic patients with severe aortic stenosis; it is rarely observed in adult patients without symptoms.
Several complex factors impact the pressure gradient that exists between the left ventricle and the ascending aorta in patients with valvular aortic stenosis. The first set of factors relates to the complex nature of fluid mechanics and flow through a stenotic orifice ( Fig. 6.9 ). Theoretical considerations of flow through a stenotic orifice predict the presence of intraventricular pressure gradients because of a drop in pressure from the body of the left ventricle to the outflow tract from a tapering of the flow field, with subsequent acceleration of blood flow as it approaches the stenotic orifice. This observation has been confirmed in patients with valvular aortic stenosis. Elegant investigations using micromanometer catheters to precisely measure the chamber pressure in patients with severe valvular aortic stenosis reveal that subvalvular gradients comprise nearly half of the total pressure gradient. This observation is critically important to clinicians using cardiac catheterization to estimate the severity of aortic stenosis because failure to properly position the catheter deep within the left ventricle may incompletely estimate the true transvalvular gradient. Fluid dynamic theory also predicts the phenomenon of pressure recovery . The maximum pressure drop and the zone of minimal pressure and maximum velocity exist at the site of obstruction (vena contracta) with the development of turbulent flow and loss of energy. Distal to the obstruction, some of this energy is recovered with the reestablishment of laminar flow, and, consequently, an increase in pressure occurs from recovery of some of the pressure dropped across the stenotic valve. This phenomenon has been observed in patients with significant aortic stenosis using micromanometer catheters to carefully measure the pressure from the left ventricle to the ascending aorta. The zone of minimal pressure occurred just above the aortic valve, and the zone of pressure recovery occurred higher in the ascending aorta. The magnitude of pressure recovery averaged 10 mm Hg. Although the fluid-filled catheters with multiple side holes used for clinical estimation of valve area may not detect pressure recovery, clinicians should be aware of this phenomenon and position the aortic catheter in the most proximal location. Neglecting the concept of pressure recovery and improper positioning of the aortic catheter when measuring a transvalvular gradient will lead to underestimation of the gradient and overestimation of the AVA with greater disparity observed with more severely narrowed aortic valves.

The second set of factors that influences the pressure gradient relates to the fact that the pressure gradient is proportional not only to orifice area but also to flow across the valve. Flow will vary with the heart rate, contractile state of the heart, and the degree of preload and afterload. Thus flow may vary from beat to beat and with the respiratory cycle. Common arrhythmias, including marked sinus arrhythmia, atrial fibrillation with variations in the RR interval, and frequent premature atrial or ventricular beats or intermittent pacing, will affect the transvalvular pressure gradient ( Fig. 6.10 ). For this reason the use of invasive techniques to estimate the severity of aortic valve stenosis mandates simultaneous left ventricular and aortic pressure measurements while maintaining the relative stability of these factors during data collection.

Determination of the Severity of Aortic Valve Stenosis
Estimating the severity of aortic stenosis often begins with noninvasive methods using echocardiographic techniques that utilize the Doppler methodology. These well-established methods provide accurate estimations of the extent of valve narrowing by estimating the transvalvular gradients and aortic valve orifice area. Severe aortic stenosis is defined by a Doppler maximum aortic velocity of greater than or equal to 4.0 m/s, a mean transvalvular gradient greater than or equal to 40 mm Hg, and an estimated valve area less than or equal to 1.0 cm 2 or an AVA indexed to body surface less than or equal to 0.6 cm 2 /m 2 . Understand that the definition of “severe” stenosis is somewhat arbitrary, and decisions regarding timing of valve replacement in a particular patient should not be constrained by fixation on a number.
The use of echocardiography to assess the severity of aortic stenosis has been well described elsewhere. The peak, or maximum, instantaneous velocity across the aortic valve is measured by continuous wave Doppler ( Fig. 6.11 ) and is an important direct measurement of the severity of aortic stenosis; a velocity of >4.0 m/s is consistent with severe aortic stenosis. The morphology of the continuous wave Doppler velocity envelope can provide helpful information regarding the severity of aortic stenosis. Mild and moderate degrees of aortic stenosis will have a rapid upstroke in the velocity envelope early in systole (see Fig. 6.11A ), while patients with severe aortic stenosis will demonstrate a delay (see Fig. 6.11B ). The peak velocity can be used to calculate a peak pressure gradient by using the simplified Bernouli equation as follows:


Note that the peak pressure gradient describes the highest gradient that exists during the cardiac cycle. The mean gradient is calculated as the average of the instantaneous gradients occurring during systole; this value is generated by the echocardiographic imaging program and is lower than the peak pressure gradient; a mean gradient greater than or equal to 40 mm Hg is consistent with severe stenosis. Finally, the valve area is calculated using the continuity equation, which states that the volume of blood passing through the left ventricular outflow tract (LVOT stroke volume) is the same as the volume of blood passing through the aortic valve orifice (AV stroke volume). In turn, the stroke volume in a tube can be determined by the formula:
Assuming a round orifice, the cross-sectional area of the LVOT can be calculated by:
Because the continuity equation states that the AV stroke volume equals the LVOT stroke volume, the AVA can be calculated by the equation:
These echocardiographic measurements form the basis for our clinical decisions; in fact, invasive methods to determine the severity of stenosis are discouraged if noninvasive methods clearly show severe stenosis. In some cases, however, noninvasive techniques may be inconclusive or of inadequate quality or may provide data that are discordant with the clinical examination or a patient’s symptoms. Pitfalls in the echocardiographic assessment of aortic stenosis severity include the inability to obtain an adequate continuous wave Doppler signal and improper measurement of the LVOT diameter. If the Doppler is not aligned with the ejected jet of blood, the peak velocity and therefore the aortic stenosis severity will be underestimated. Inaccurate measurement of the LVOT diameter is another common source of errors in valve area calculation. The stenosis severity will be overestimated if the LVOT diameter is erroneously measured too small, and the stenosis severity will be underestimated if the LVOT diameter is erroneously measured too large.
When the degree of stenosis is unclear from echocardiography, clinicians turn to an invasive assessment. There is evidence that in the current era the correlation between invasive and noninvasive determinations of stenosis severity is lower than previously thought because of variability in echo measurements, thus supplementary invasive data are often helpful in borderline cases.
Invasive methodology applies an adaptation of the Gorlin formula to calculate the area of the stenotic aortic valve. This technique has been in clinical use for many years, is fairly simple to calculate from variables obtained during catheterization, and provides clinicians and patients an easy-to-visualize number (i.e., AVA in centimeters squared).
The Gorlin Formula for the Estimation of AVA
The mathematical derivation of the Gorlin formula to estimate the orifice area is based on the idealized physics of hydraulic systems and has been explained during the discussion of mitral valve stenosis (see Chapter 5 ). Gorlin solved the equation using the valve area of a stenotic mitral valve measured at autopsy from a single patient and determined the value of the coefficient C to be 0.7. He then compared the calculated valve area with the area measured at either autopsy (six patients) or operation (five patients) for validation. Gorlin noted that the formula could be adapted to the aortic valve by using the systolic ejection period (SEP) to account for the time that flow occurs across the aortic valve, but he did not know the value of the coefficient. This has subsequently never been determined. Nevertheless, the Gorlin formula applied to the aortic valve is generally used as follows:
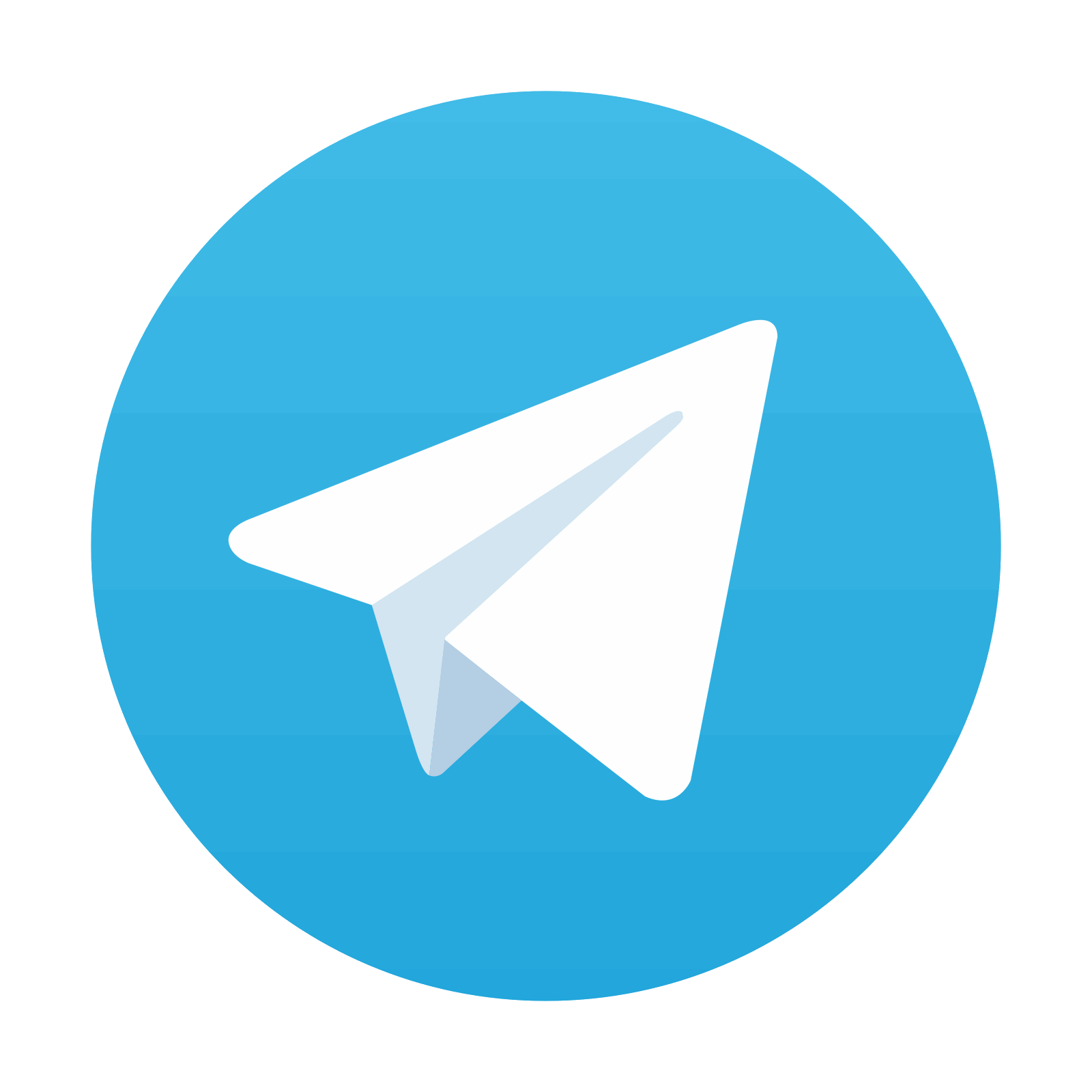
Stay updated, free articles. Join our Telegram channel

Full access? Get Clinical Tree
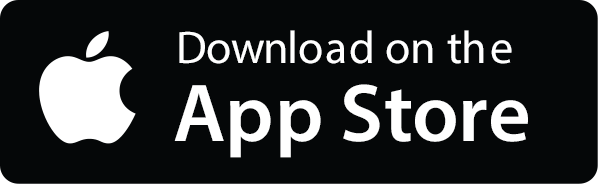
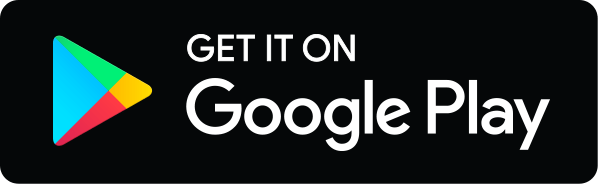
