Although cardiac adaptation to different sports has been extensively described, the potential effect of top-level training on the aortic root dimension remains not investigated fully. To explore the full range of aortic root diameters in athletes, 615 elite athletes (370 endurance-trained athletes and 245 strength-trained athletes; 410 men; mean age 28.4 ± 10.2 years, range 18 to 40) underwent transthoracic echocardiography. The end-diastolic aortic diameters were measured at 4 locations: (1) the aortic annulus, (2) the sinuses of Valsalva, (3) the sinotubular junction, and (4) the maximum diameter of the proximal ascending aorta. Ascending aorta dilation at the sinuses of Valsalva was defined as a diameter greater than the upper limit of the 95% confidence interval of the overall distribution. The left ventricular (LV) mass index and ejection fraction did not significantly differ between the 2 groups. However, the strength-trained athletes had an increased body surface area, sum of wall thickness (septum plus LV posterior wall), LV circumferential end-systolic stress, and relative wall thickness. In contrast, the left atrial volume index, LV stroke volume, and LV end-diastolic diameter were greater in the endurance-trained athletes. The aortic root diameter at all levels was significantly greater in the strength-trained athletes (p <0.05 for all comparisons). However, ascending aorta dilation was observed in only 6 male power athletes (1%). Mild aortic regurgitation was observed in 21 athletes (3.4%). On multivariate analyses, in the overall population of athletes, the body surface area (p <0.0001), type (p <0.001) and duration (p <0.01) of training, and LV circumferential end-systolic stress (p <0.01) were the only independent predictors of the aortic root diameter at all levels. In conclusion, the aortic root diameter was significantly greater in elite strength-trained athletes than in age- and gender-matched endurance athletes. However, significant ascending aorta dilation and aortic regurgitation proved to be uncommon.
The athlete’s heart is a cardiac adaptation to long-term, intensive training, characterized mainly by an increase in cavity diameters, wall thickness, and left ventricular (LV) mass. Although divergent models of cardiac remodeling in different sports (eccentric vs concentric LV hypertrophy in endurance- vs strength-trained athletes, respectively) have been extensively described, the potential effect of top-level training on aortic root morphology remains not fully investigated. However, it has been hypothesized that the brief increases in cardiac output associated with great elevation of systemic arterial pressure, correlating with power training, could cause a chronically elevated aortic wall tension responsible for aortic dilation and regurgitation. The aim of the present study was to investigate the range of aortic root dimensions and the relative training effect in a large population of elite athletes.
Methods
From June 2008 to April 2009, 620 consecutive elite athletes were referred to the Sports Medicine Ambulatory service of Monaldi Hospital (Naples, Italy) for cardiovascular preparticipation screening and afterward to our echocardiographic laboratory for the purposes of the present study. All subjects underwent a detailed history, physical examination, electrocardiography, chest radiography, and comprehensive transthoracic echocardiography, including Doppler studies. The diagnosis of Marfan syndrome was made according to the clinical criteria defined by Pyeritz and McKusick. Any subject thought to have Marfan syndrome was referred for slit-lamp examination with dilated pupils by an ophthalmologist and a complete evaluation of the skeletal system by an orthopedist. Those with Marfan syndrome (n = 1), Ehlers-Danlos syndrome (n = 1), and bicuspid aortic valves (n = 3) were excluded from the present study. The final study population included 615 competitive athletes.
On the basis of their training protocol and the type of sport’s activity (mainly dynamic or static) and for the purposes of the present study, they were categorized into 2 groups: endurance-trained athletes (ETAs, n = 370) and strength-trained athletes (STAs, n = 245). The local ethics committee approved the study.
All the athletes had been trained intensively for 15 to 20 hours/week for >4 years. The ETA group (long- and middle-distance swimming or running, soccer, basketball) underwent intensive aerobic isotonic dynamic exercise at incremental workloads of 70% to 90% of their maximal heart rate. In particular, they performed 3 hours/day of incremental long-distance swimming (7,000 m/day divided into series of 400 to 800 m) or 3 hours/day of long-distance running, with only 2 hours/week of weight lifting at a low workload. In contrast, the STA group (body-building, weight-lifting, martial arts, windsurfing) underwent anaerobic isometric static exercise at incremental workloads at 40% to 60% of their maximal heart rate. Their training protocol included 3 hours/day of short-distance running and 3 hours/day of weight lifting at a high workload.
Standardized transthoracic echocardiographic and Doppler examinations were performed using commercially available equipment for all subjects (Vivid 7, GE Healthcare, Milwaukee, Wisconsin). All studies were reviewed and analyzed off-line by 2 independent observers who were unaware of the clinical characteristics of the study population. Specific measurements were made using the average of 3 to 5 cardiac cycles.
The M-mode measurements (LV diastolic and systolic diameters, interventricular septum, and posterior wall thickness) were performed in the parasternal long-axis view with the patient in the left lateral position. The LV mass was calculated using the Penn convention and indexed for height (Cornell adjustment). The relative diastolic wall thickness was determined as the ratio between the sum of septal and posterior wall thickness and the LV end-diastolic diameter. The circumferential end-systolic stress (ESSc) was calculated as a measurement of LV afterload using a cylindrical model according to the following formula: ESSc (g/cm 2 ) = SBP × ½Ds {1 + [(½DS + PS) /(½DS + ½PS) ]}/(½DS + PS) − ½DS, where SBP was the systolic blood pressure, DS was the end-systolic diameter, and PS was the posterior wall thickness in systole.
The LV ejection fraction was calculated using Simpson’s rule in the apical 4-chamber view. The left atrial maximal volume was measured at the point of mitral valve opening using the biplane area–length method and corrected for body surface area.
Valvular regurgitation was quantified from color Doppler imaging and categorized as absent, minimal (within normal limits), mild, moderate, or severe. In particular, the severity of aortic regurgitation was assessed by the width of the regurgitant jet on color Doppler imaging and the rate of decrease of regurgitant flow on the continuous-wave Doppler signal.
Doppler-derived LV diastolic inflow was recorded in the apical 4-chamber view by placing the sample volume at the tip level. The following LV diastolic measurements were measured: E and A peak velocities (m/s) and their ratio, E wave deceleration time (ms), isovolumic relaxation time (ms, as the interval occurring between the end of systolic output flow and the transmitral E wave onset, by placing the pulsed Doppler sample volume between the outflow tract and mitral valve).
The LV stroke volume was calculated as the LV outflow tract area × outflow tract time velocity integral. The cardiac index was calculated as the stroke volume × heart rate/body surface area.
Two-dimensional measurements of the aortic root were made at end-diastole in the parasternal long-axis views at 4 levels: (1) the annulus (defined echocardiographically as the hinge points of the aortic cusps); (2) the sinuses of Valsalva; (3) the supra-aortic ridge; and (4) the proximal ascending aorta ( Figure 1 ). The measurements were made perpendicular to the long axis of the aorta using the leading edge technique in the views showing the largest aortic diameters. The diagnosis of ascending aorta dilation (AAD) was determined solely from the morphologic analysis of the aorta, and dilation was suspected in the case of an aortic root dimension at the sinus of Valsalva greater than the upper limit of the 95% confidence interval of the overall distribution. In those cases, enhanced thoracic and abdominal computed tomography was recommended, in addition to a detailed assessment of the ascending aorta on a 2-dimensional echocardiogram. If localized dilation of the ascending aorta was confirmed, and coarctation of the aorta was ruled out by both radiologists and cardiologists, a clinical diagnosis of AAD was made.
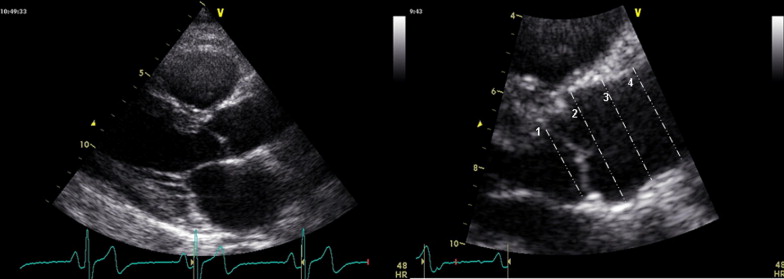
All the analyses were performed using the Statistical Package for Social Sciences for Windows, release 14.0 (SPSS Italy, Rome, Italy). The data are presented as the mean ± SD. The t test for unpaired data was used to the estimate the differences between the 2 groups. Stepwise forward, multiple linear regression analyses were performed to weigh the independent effects of the potential determinants not obviously related to each other as a dependent variable. Differences were significant at p <0.05.
Results
The clinical characteristics of the study population are listed in Table 1 . The mean age was comparable between the 2 groups. In accordance with the effects of the different training protocols, the STAs at rest had a greater heart rate, body surface area, and systolic blood pressure than did the ETAs.
Variable | Overall (n = 615) | Endurance (n = 370) | Strength (n = 245) | p Value |
---|---|---|---|---|
Age (years) | 28.4 ± 10.2 | 27.8 ± 10.1 | 29.2 ± 10.4 | NS |
Gender (n) | NS | |||
Men | 390 | 230 | 160 | |
Women | 225 | 140 | 85 | |
Body surface area (m 2 ) | 1.86 ± 0.6 | 1.84 ± 0.5 | 1.89. ± 0.6 | <0.01 |
Heart rate (beats/min) | 60.5 ± 9.6 | 52.1 ± 4.4 | 68.9 ± 9.8 | <0.001 |
Systolic blood pressure (mm Hg) | 124.8 ± 7.8 | 116.8 ± 8.1 | 132.9 ± 7.1 | <0.001 |
Diastolic blood pressure (mm Hg) | 75.6 ± 4.5 | 72.7 ± 4.9 | 78.9 ± 4.0 | NS |
The STAs had an increased sum of wall thickness (septum plus LV posterior wall), LV relative wall thickness, and ESSc. In contrast, the LV end-diastolic diameter was greater in the ETAs. The LV mass index and LV ejection fraction did not differ significantly between the 2 groups, but the left atrial volume index was increased in the ETAs. The prevalence of aortic regurgitation was comparable between the 2 groups. In contrast, all transmitral Doppler indexes were greater in the ETAs, with an increased E peak velocity, E/A ratio, and isovolumic relaxation time. The LV stroke volume and cardiac index were also greater in the ETAs ( Tables 2 and 3 ).
Variable | Overall (n = 615) | Endurance (n = 370) | Strength (n = 245) | p Value |
---|---|---|---|---|
Septal wall thickness (mm) | 10.5 ± 2.8 | 9.7 ± 3.1 | 11.3 ± 2.4 | <0.01 |
Posterior wall thickness (mm) | 10.4 ± 2 | 9.2 ± 2.1 | 11.6 ± 1.6 | <0.01 |
Sum of wall thickness (mm) | 20.5 ± 3.8 | 18.7 ± 4.4 | 22.3 ± 2.8 | <0.001 |
Left ventricular end-diastolic diameter (mm) | 52.8 ± 3.9 | 55.4 ± 4.7 | 48.2 ± 3.3 | <0.001 |
Left ventricular end-systolic diameter (mm) | 28.1 ± 3.4 | 28.3 ± 2.9 | 27.8 ± 4.1 | NS |
Relative diastolic wall thickness | 0.41 ± 0.05 | 0.37 ± 0.04 | 0.45 ± 0.06 | <0.001 |
Left ventricular mass index (g/m 2.7 ) | 63.6 ± 9.3 | 62.8 ± 9.9 | 64.5 ± 8.7 | NS |
Left ventricular circumferential end-systolic stress (g/cm 2 ) | 124.4 ± 18.4 | 90.3 ± 15.2 | 158.6 ± 19.5 | <0.001 |
Left ventricular ejection fraction (%) | 68.9 ± 4.3 | 69.7 ± 4.7 | 67.1 ± 3.8 | NS |
Left atrial volume index (mL/m 2 ) | 27.4 ± 8.8 | 29.1 ± 9.3 | 26.2 ± 8.4 | <0.01 |
Variable | Overall (n = 615) | Endurance (n = 370) | Strength (n = 245) | p Value |
---|---|---|---|---|
Aortic valve regurgitation (%) | 3.4% | 3.3% | 3.6% | NS |
Mitral valve regurgitation (%) | 10.3% | 10.9% | 9.8% | NS |
Tricuspid valve regurgitation (%) | 89.4% | 90.6% | 88.2% | NS |
Pulmonary valve regurgitation (%) | 95.3% | 96.4% | 94.3% | NS |
Peak E velocity (m/s) | 0.86 ± 0.15 | 0.94 ± 0.13 | 0.79 ± 0.16 | <0.01 |
Peak A velocity (m/s) | 0.45 ± 0.23 | 0.45 ± 0.12 | 0.46 ± 0.14 | NS |
Peak E/A ratio | 1.95 ± 0.7 | 2.2 ± 05 | 1.7 ± 0.8 | <0.001 |
E wave deceleration time (ms) | 162.5 ± 16.8 | 165.8 ± 16.3 | 159.5 ± 17.7 | NS |
Isovolumic relaxation time (ms) | 74.5 ± 18.3 | 79.8 ± 20.7 | 69.3 ± 15.6 | <0.05 |
Left ventricular stroke volume (ml) | 83.4 ± 5.9 | 98.4 ± 6.2 | 69.4 ± 3.3 | <0.01 |
Left ventricular cardiac index (mL/min/m 2 ) | 2.6 ± 0.6 | 2.8 ± 0.7 | 2.4 ± 0.3 | <0.01 |
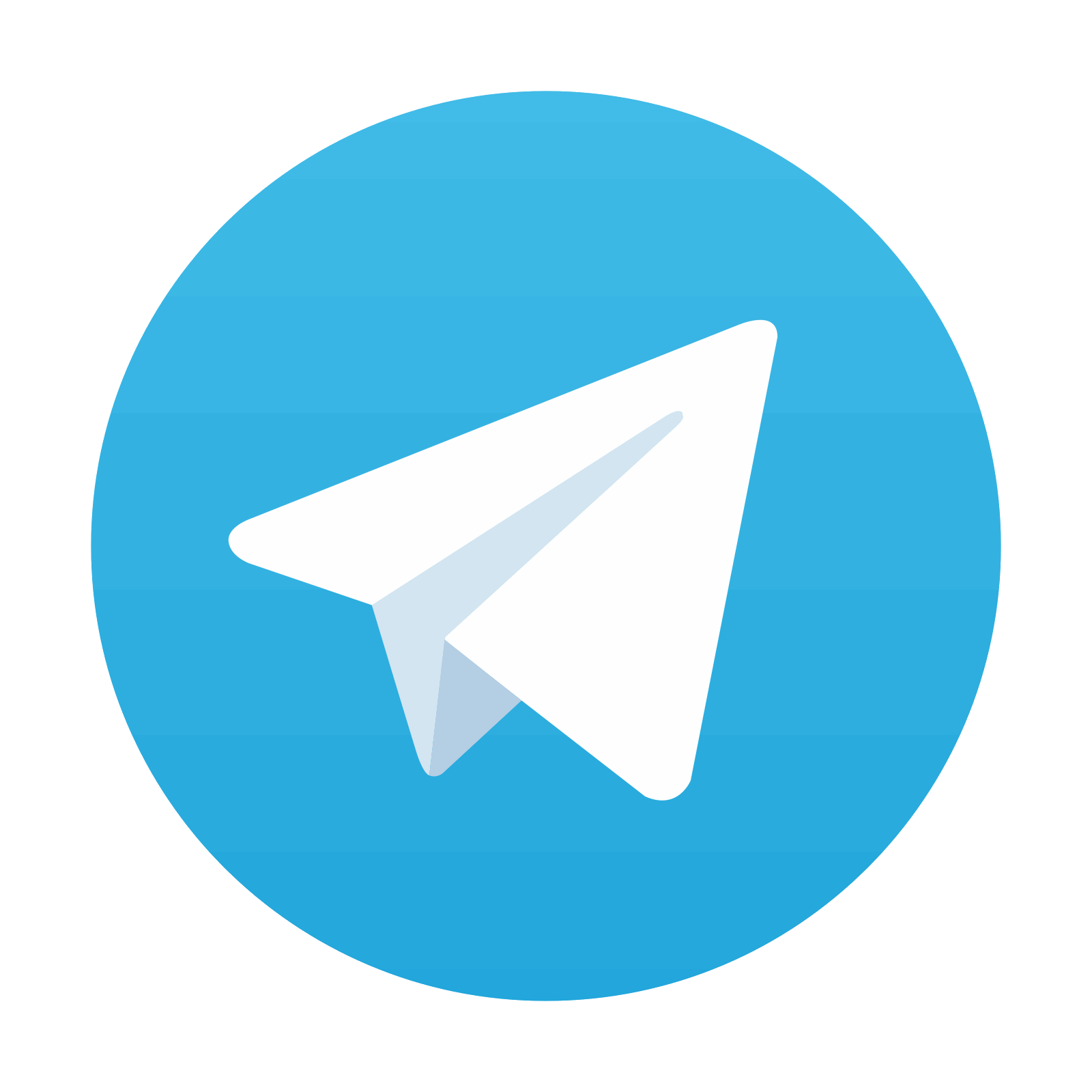
Stay updated, free articles. Join our Telegram channel

Full access? Get Clinical Tree
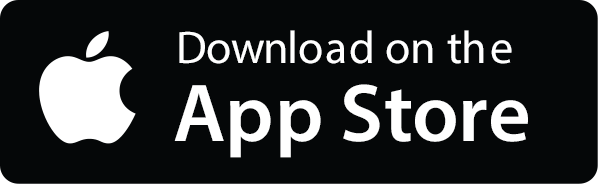
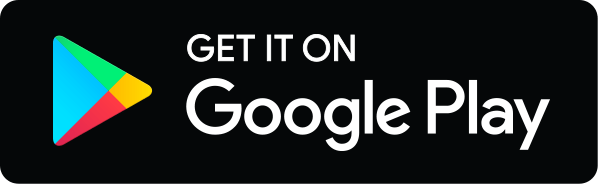
