Atherosclerosis of the proximal branches of the aortic arch has compelling clinical implications that warrant the application of direct noninvasive detection of the disease. The prevalence of aortic arch vessel disease in an aging and at-risk community and clinical population has been underreported and undertreated despite an associated increase of all-cause and cardiovascular mortality. Intrathoracic duplex imaging has been validated as an accurate noninvasive tool to detect, characterize, and follow native aortic arch vessel disease and its sequelae and correction. Such duplex techniques are easily integrated into routine echocardiography with focused training and minimal time investment in the examination. A paucity of available resources exists across disciplines regarding ultrasonographic investigation of these supra-aortic trunk vessels, including textbooks, journal articles, seminars, and manuals. This review has been compiled to familiarize physicians and sonographers with the relevant anatomy, pathophysiology, treatment, and diagnostic duplex surveillance of aortic arch vessel disease. Illustrative cases along with clinical rationale are discussed with the intent to facilitate the integration of arch vessel duplex imaging into the scope and practice of echocardiography.
Attention ASE Members:
ASE has gone green! Visit www.aseuniversity.org to earn free CME through an online activity related to this article. Certificates are available for immediate access upon successful completion of the activity. Non-members will need to join ASE to access this great member benefit!
Arch Vessel Anatomy
Several normal anatomic variations and anomalous vessel branching patterns radiating from the outer curvature of the aortic arch have been described. The standard arch configuration shows a shared brachiocephalic trunk, called the innominate artery (IA), which bifurcates superiorly, giving rise to the right common carotid artery (RCCA) and right subclavian artery (RSA). The left common carotid artery (LCCA) and left subclavian artery (LSA), in downstream sequence, have separate origins ( Figure 1 A). The diameter of these supra-aortic trunk vessels ranges from 5 to 9 mm, averaging 7.2 ± 0.6 mm, determined angiographically. The so-called bovine arch variation is apparent when the LCCA merges proximally off of a single trunk in common with the IA. In this instance, occurring overall in 13% of the general population (25% of African Americans, 8% of whites), there are a total of two brachiocephalic origins ( Figure 1 C). The bovine namesake is actually a misnomer, as the true bovine pattern found in cattle manifests a single brachiocephalic trunk from the arch. Yet a third anatomic variation occurs when the LCCA has a more distal, distinct origin from the IA trunk and is seen in about 9% of the population (10% of African American, 5% of whites) ( Figure 1 B). Additionally, two rare anatomic variants occur, one with a common left brachiocephalic trunk (LCCA and LSA) and another as a separate vertebral artery originating from the arch, seen in 1% and 0.5%, respectively ( Figure 1 D). There are multiple rare arch vessel branching anomalies that can be classified according to the position and caliber of the aorta and number of abnormalities. The most common aberration is the left aortic arch that gives rise to an anomalous RSA (0.4%–2%). A bicarotid trunk arising from the aorta is also found in association with an anomalous origin of the RSA as it branches from a common subclavian trunk at a more distal arch position. The right aortic arch with an aberrant LSA and double aortic arch are both associated with vascular rings that can encircle the trachea and esophagus, causing symptomatic impingement of those structures. The right aortic arch with mirror image branching, and left-sided or right-sided cervical arches associated with vessel branching variations, are seen among other rare congenital malformations. Patients with clinically significant arch anomalies are imaged best noninvasively using magnetic resonance angiography and CTA. Familiarity with these aortic arch and vessel attributes is fundamental to the acquisition and interpretation of duplex images demonstrating arch vessel disease.
Arch Vessel Anatomy
Several normal anatomic variations and anomalous vessel branching patterns radiating from the outer curvature of the aortic arch have been described. The standard arch configuration shows a shared brachiocephalic trunk, called the innominate artery (IA), which bifurcates superiorly, giving rise to the right common carotid artery (RCCA) and right subclavian artery (RSA). The left common carotid artery (LCCA) and left subclavian artery (LSA), in downstream sequence, have separate origins ( Figure 1 A). The diameter of these supra-aortic trunk vessels ranges from 5 to 9 mm, averaging 7.2 ± 0.6 mm, determined angiographically. The so-called bovine arch variation is apparent when the LCCA merges proximally off of a single trunk in common with the IA. In this instance, occurring overall in 13% of the general population (25% of African Americans, 8% of whites), there are a total of two brachiocephalic origins ( Figure 1 C). The bovine namesake is actually a misnomer, as the true bovine pattern found in cattle manifests a single brachiocephalic trunk from the arch. Yet a third anatomic variation occurs when the LCCA has a more distal, distinct origin from the IA trunk and is seen in about 9% of the population (10% of African American, 5% of whites) ( Figure 1 B). Additionally, two rare anatomic variants occur, one with a common left brachiocephalic trunk (LCCA and LSA) and another as a separate vertebral artery originating from the arch, seen in 1% and 0.5%, respectively ( Figure 1 D). There are multiple rare arch vessel branching anomalies that can be classified according to the position and caliber of the aorta and number of abnormalities. The most common aberration is the left aortic arch that gives rise to an anomalous RSA (0.4%–2%). A bicarotid trunk arising from the aorta is also found in association with an anomalous origin of the RSA as it branches from a common subclavian trunk at a more distal arch position. The right aortic arch with an aberrant LSA and double aortic arch are both associated with vascular rings that can encircle the trachea and esophagus, causing symptomatic impingement of those structures. The right aortic arch with mirror image branching, and left-sided or right-sided cervical arches associated with vessel branching variations, are seen among other rare congenital malformations. Patients with clinically significant arch anomalies are imaged best noninvasively using magnetic resonance angiography and CTA. Familiarity with these aortic arch and vessel attributes is fundamental to the acquisition and interpretation of duplex images demonstrating arch vessel disease.
Examination Technique
Duplex surveillance of the intrathoracic supra-aortic trunk vessels may be accomplished as an adjunct to the standard echocardiographic examination of the aortic arch. The supine patient is instructed to slightly hyperextend the neck and, if necessary, turn the head to the right, facilitating placement of the ultrasound probe in the suprasternal notch. The long axis of the aorta is displayed using a 2-MHz to 5-MHz multifrequency phased-array transducer with system settings to maximize resolution. High-resolution harmonic imaging is beneficial, as is sacrificing frame rate in favor of improved image detail, because the temporal resolution normally required for dynamic cardiac motion is not an issue with these structures. Usually, the arch vessels are readily apparent on grayscale imaging and can be visualized in their longitudinal orientation either simultaneously with the arch or with minimal manipulation ( Figure 2 A). Qualitative color flow Doppler imaging is routinely used to differentiate and locate the arch vessels and discern laminar versus disturbed flow patterns ( Figure 2 B). The color Doppler scale should be set to 40 to 60 cm/sec and optimized by adjusting the color box size, image depth, sector size, and gain. More extensive quantitative Doppler sampling is reserved for absent or disturbed flow signals.
Careful inspection of the arch vessels typically begins with the LSA and continues in a retrograde and medially directed fashion to include the LCCA, followed by the IA and its branches. Color Doppler optimization will help identify the LSA takeoff signal, whose flow stream tends to be somewhat perpendicular to the incident beam. The LSA is also deeper and prone to reverberation image artifact, whereby one or two weaker “ghost” color flow Doppler signals may appear posteriorly. When image quality is marginal, this artifact presents an obstacle to confirming a variant separate origin of the left vertebral artery directly from the arch. Highly suboptimal arch images, although not uncommon, do not preclude inspection of the arch vessels. In such cases, a strategy of finding the more distal vessels and following them back to their arch origins, using complementary color flow and spectral Doppler, is frequently successful. For example, by sliding the probe over the left sternocleidomastoid muscle or into the left supraclavicular fossa, the distal portion of the LSA may be identified and traced back to the arch, allowing inspection of its proximal takeoff. The LCCA has a distinct curvilinear course and is typically imaged along its proximal trunk without difficulty. Its ease of imaging and distinguishing features can be used as a helpful landmark to locate the other arch vessels in technically challenging examinations. Slight counterclockwise probe rotation while migrating superiorly will permit visualization of the IA, frequently laying out its RCCA and RSA bifurcation, whereby the proximal and quite superficial portions of these trunks can be evaluated ( Figure 3 ). When unable to demonstrate the IA bifurcation, the RSA and RCCA should be located and examined individually. Following the natural cephalad course of the IA from the usual echocardiographic arch image will allow swift screening and visualization of its branching with minimal probe manipulation. Although the display of RCCA and RSA branching is anatomically reversed, retaining standard arch orientation and continuity is essential particularly when technically difficult examinations require reliance on color flow mapping to identify relative arch vessel positions. Electronic left-to-right image inversion of the bifurcation of the IA trunk can be used, if desired, to reorient the vessels to anatomic and angiographic views, especially when demonstrating lesions. Electronically inverted images should be clearly annotated as such on the image to avoid confusion. The shallow branches of the IA and more superficial courses of the other arch vessels, including the vertebral artery origins are best imaged with high-frequency linear-array transducers. This is normally integrated within the extracranial carotid survey, which may also include provocative arm exercise or reactive hyperemia to assess vascular steal.
Careful attention to these arch vessel imaging techniques permits efficient and accurate qualitative confirmation of normal anatomy and laminar color Doppler flow signals. A study published in 1984 by Ackerstaff et al. identified 112 of 118 (95%) of angiographically normal subclavian arteries. Another report on 20 patients with Takayasu’s arteritis found that duplex imaging, validated by angiography, correctly distinguished normal vessels in 12 of 15 IAs (80%), 12 of 13 LCCAs (92%), and all subclavian arteries. In our experience, normal arch vessel anatomy and flow are confirmed by adding 30 to 60 sec to the echocardiographic aortic arch examination.
When color flow Doppler turbulence is apparent, best adjustment of the real-time image and quantitative Doppler interrogation is performed. Split-screen comparative display techniques with and without color flow Doppler are often helpful by providing anatomic context with the arch and proximal vessel trunks. After image depth and quality are fine tuned, digital clips are acquired, identifying the vessel and demonstrating lesion characteristics, such as mural thickening and plaque calcification and irregularity. Acoustic shadowing due to calcified vessels and lesions may interfere with color flow and spectral Doppler signal quality. Color flow Doppler is used to guide sample volume placement and determine flow angle, which is enhanced by probe angulations to achieve a Doppler incident angle of ≤60°. This will optimize quantitative Doppler spectral signals before angle correction. Although angle correction is strongly discouraged in cardiac Doppler applications, the technique is widely applied in noninvasive vascular sonography and is essential to estimate peak systolic velocity in the arch vessels. By convention, antegrade flow should be displayed above the baseline as a positive velocity. On occasion, the RSA, and rarely other tortuous arch vessel trunks, requires signal inversion to accurately display flow direction. This further underscores the need to use techniques from both cardiac and noninvasive vascular approaches in evaluating AAVD. Angle corrections >60° are susceptible to large velocity errors and should be avoided. Poor Doppler angles are common in the subclavian arteries, in which case interrogation from the supraclavicular fossa should prove helpful. Unless the angle to the flow stream can be confidently determined, the sonographer should use imaging strategies to measure maximal, uncorrected flow velocities from the most parallel orientation. The IA flow direction is typically in line with the ultrasound beam, eliminating the need for angle compensation. The LCCA Doppler velocity invariably requires angle correction, although favorable incident angles are quite easily achieved. Pulsed-wave Doppler is useful for identifying lesion site and peak flow velocity quantification while demonstrating spectral broadening. Image-guided continuous-wave Doppler is frequently beneficial, as the primary diagnostic parameter determining lesion hemodynamic significance is peak systolic velocity.
Although flow criteria have not been directly applied to arch vessel Doppler interrogations, carotid, renal, and peripheral arterial guidelines should prove both instructive and applicable. An authoritative noninvasive vascular text cites a normal range for subclavian artery velocity of 70 to 120 cm/sec. A threshold of >175 cm/sec to track the progression of hemodynamic significance of asymptomatic carotid artery stenosis risk for progression beyond 60% narrowing has been described. Ratios using peak systolic and end-diastolic velocities commonly determined in carotid evaluations do not find relevance in the high-resistance brachiocephalic arterial flow profiles. Renal artery velocities > 180 to 200 cm/sec are said to correlate with stenoses ≥ 60%. These flow ranges and their corresponding derivative peak systolic pressures have been supported by hemodynamically significant invasive gradients of ≥20 mm Hg determined in a systematic approach to evaluate aortoiliac occlusive disease. Angle-corrected pulsed-wave and continuous-wave Doppler quantitations, as applicable, are an especially useful adjunct in vessels with poor image delineation but evidence of flow disturbance by color mapping. Abnormal velocities are typically >180 to 200 cm/sec on the basis of the previously mentioned large vessel cerebrovascular and peripheral arterial criteria. In our laboratory, we apply a threshold of 225 cm/sec to identify hemodynamically significant lesions with critical stenoses reaching velocities in the 275 to 500 cm/sec range. Unfortunately, specific guidelines for defining hemodynamically significant AAVD Doppler findings and Doppler-derived peak and mean pressure gradients have not as yet been validated. Monophasic, low-velocity profiles are suggestive of either trickle or collateral flow from complete or subtotal narrowing. When an arch vessel cannot be identified, every effort should be made to aggressively seek a flow signal, including, if available, power Doppler. The sonographer should be wary of potential arch vessel branching variations, such as the so-called bovine arch, before a diagnosis of vessel occlusion is made. Sequential and, preferably, automated bilateral arm blood pressure determinations are obligatory after abnormal arch vessel examinations and should be correlated with Doppler findings on the report. When clinically indicated, patients with positive results are referred for confirmatory angiography.
Arch Vessel Disease
Atherosclerosis is far and away the most prevalent cause of obstructive lesions in the supra-aortic trunks. This degenerative process is abetted by the usual risk factors. Nonmodifiable risk factors of advancing age, male gender, and family history are coupled to the familiar set of modifiable ones: smoking, diabetes mellitus, hypertension, and elevated lipid levels. Increased high-sensitivity C-reactive protein concentration, hyperfibrinogenemia, hyperhomocysteinemia, and chronic kidney disease are markers having particular associations with peripheral arterial disease (PAD). Arterial branching and bifurcation points, such as the supra-aortic trunks, are more vulnerable to atheromatous progression due to hemodynamic effects and stress. As atherosclerotic plaques engorge and mature, they become susceptible to erosion and rupture, intraplaque hemorrhage and ulceration, embolic generation, calcification, and eventual narrowing or obliteration with concomitant hemodynamic blood flow alterations. A postmortem analysis found that 22% of IA atherosclerotic lesions were ulcerative. A study of 113 patients undergoing endovascular interventions for subclavian artery stenosis (SAS) discovered lesion calcification in 55%, eccentricity in 52%, and ulcerative plaque in 38%. The angiographically proved prevalence of significant subclavian artery disease is on the order of 0.5% to 2.0% of the general population. Some authors have cited the lack of large community-based studies and standardized methods to systematically screen and diagnose arch vessel disease as factors contributing to the underestimation of its prevalence and impact. When systolic interarm blood pressure difference (IAPD) is used to estimate subclavian artery vascular disease, the estimates are much higher. An exhaustive meta-analysis by Clark et al. recently concluded that systolic blood pressure measurement exceeding 10 to 15 mm Hg between arms occurs in 10% of patients with diabetes and up to 20% of those with hypertension. These findings are consistent with those of lower extremity PAD, which is also commonly underdiagnosed and undertreated.
The most definitive studies examine arch vessel disease in certain at-risk populations, such as those with extracranial or coronary atherosclerosis. The Joint Study of Extracranial Arterial Occlusion VII found that 17% of 6,534 subjects with symptoms of cerebral ischemia, randomized between surgical and nonsurgical treatment of extracranial disease, had ≥30% arteriographically determined stenosis of a subclavian artery or the IA. English et al. reported that 3.5% of patients undergoing cardiac catheterization had angiographic confirmation of SAS, rising to 5.3% in those with surgical coronary disease. Lobato et al. examined 86 consecutive patients with prior documented internal mammary grafts referred for noncardiac surgery. In six subjects with IAPD ≥ 20 mm Hg, four (5%) had angiographically proved ipsilateral SAS with myocardial ischemia due to coronary-subclavian steal.
The distribution of supra-aortic trunk lesions heavily favors the proximal LSA. Henry et al. , using a standard of ≥70% angiographic narrowing for the subclavian trunks in 113 patients, detected 3% of the IA, 13% of the RSA, 84% of the LSA, and a combined 17% with total occlusions. Further detailed classification of the subclavian stenoses revealed that 80% were proximal to the vertebral artery origins, 10% distal to the vertebral artery origins, and 11% both pre and post. Sullivan et al. reported on a series of 83 patients who had primary stenting of the proximal arch vessels. They retrospectively reviewed interventions on the following distribution of lesions: 66 patients (75.9%) of the subclavian arteries, 14 (16.1%) of the common carotid origins, and seven (8%) in the IA. In general, subclavian lesions occur, by a factor of 3, greater on the left side.
The defining clinical and hemodynamic manifestations of AAVD are associated with vascular steal phenomena. The subclavian arteries supply the vertebrobasilar and upper extremity vascular territories. From the hemodynamic perspective, an occluded or severely stenosed proximal subclavian or innominate lesion can instigate blood flow reversal or “steal” from the ipsilateral vertebral artery and its corresponding cerebrovascular bed. The term subclavian steal syndrome is applied when documented retrograde vertebral artery flow causes symptoms of cerebral ischemia primarily related to vertebrobasilar insufficiency or ipsilateral upper extremity rest pain or claudication. Transient vertebrobasilar complaints are seen in the majority of symptomatic patients and are usually expressed as syncope, vertigo, or dizziness ( Figure 4 ). Hemispheric symptoms of anterior cerebrovascular ischemia associated with arch vessel disease are due primarily to concomitant extracranial carotid disease. Upper extremity and cerebral ischemic symptoms may be exacerbated with exercise or reactive hyperemia, which amplifies the steal by increasing extremity vascular bed demand. A rich supply of intracranial pathways and thyrocervical trunk collaterals in the shoulder are protective against permanent neurologic deficit. Subclavian-carotid siphoning is possible because of critical stenosis or occlusion of a brachiocephalic trunk such as the IA, resulting in a more pronounced hemodynamic defect and disabling cerebrovascular insufficiency.
In patients with ipsilateral in-situ internal mammary artery (IMA) grafts the subclavian artery becomes an integral part of the corresponding distal coronary circulation. Symptomatic or objective evidence of myocardial ischemia from reversal of IMA graft flow in the presence of significant subclavian arterial stenosis defines the coronary-subclavian steal syndrome (CSSS). Most patients with CSSS experience angina as the presenting symptom, although asymptomatic or silent ischemia is also seen. Congestive heart failure, ischemic cardiomyopathy, and acute myocardial infarction have been observed as well ( Figure 4 ). Symptoms of upper extremity and cerebral insufficiency due to the associated subclavian-vertebral steal may accompany cardiac symptoms. In a subset of 27 patients with CSSS, Sullivan et al. found that 16 (59.3%) had stable angina, and 11 (40%) presented with acute coronary syndromes or myocardial infarctions. Adverse effects of CSSS are potentially disastrous, making its detection and treatment imperative.
Large-vessel vasculitis is an extremely rare cause of AAVD. Takayasu’s arteritis is a panarteritis with a 10-fold female preponderance. Those afflicted tend to be young and of Asian descent. Takayasu’s arteritis is characterized by thickening of the vessel intima-media complex causing luminal impingement. The clinical presentation is often asymptomatic and indolent but may manifest as upper extremity claudication, bruits, diminished or absent pulses, and renovascular hypertension. Giant cell arteritis, likewise, involves granulomatous infiltrative damage but is generally seen in patients aged > 50 years. Clinical manifestations include temporal arteritis (70%) and polymyalgia rheumatica (30%–50%). Aortitis and potential arch vessel involvement occur in about 15% of patients affected by giant cell arteritis. Radiation arteritis as sequelae from brachytherapy for Hodgkin lymphoma may also give rise to arch vessel occlusive disease.
Diagnosis and Treatment
Flush arch aortography with four-vessel cerebral arteriography remains the diagnostic standard for the identification and quantification of AAVD and decision making for surgical and endoluminal reconstruction. This is achieved by visualization in the 30° to 60° left anterior oblique position delivering a radiographic contrast bolus through a pigtail catheter positioned in the ascending aorta, yielding a longitudinal arch image similar that of the echocardiographic suprasternal aortic arch long-axis view. Selective cannulation and multiplanar opacification of involved aortic trunk vessels using digital subtraction techniques better delineate target lesions. Stenoses are assessed according to site, lesion characteristics (eccentricity, ulceration, thrombus burden, and calcification), and length and extent of luminal narrowing compared with nearby normal segments. CTA and magnetic resonance angiography are other less invasive angiographic techniques, but they have a tendency to overestimate vascular lesion severity. Definitive studies are needed to establish valid alternatives to invasive digital subtraction angiography (DSA) in detecting proximal disease of the arch vessel takeoffs before surgical and endovascular treatment of extracranial vascular disease.
Noninvasive techniques have a vital role in the detection and monitoring of AAVD and its treatment. The most basic noninvasive screening test for AAVD is the measurement of systolic IAPD, which is equated to subclavian artery occlusive disease in much the same way as ankle-brachial index is to lower extremity atherosclerosis. A peak systolic difference of 10 to 20 mm Hg or more identifies patients at elevated risk for AAVD who could benefit from further assessment. Established guidelines for clinicians stipulate repeat sequential arm pressure determinations in patients with hypertension, beginning with their initial evaluation. The Joint Study Group on Subclavian Steal found that all but nine of 168 patients (5.4%) with documented subclavian steal syndrome had ≥20 mm Hg of interarm blood pressure gradient. English et al. recommended a threshold of only 10 mm Hg in arm pressure asymmetry for those patients with surgical coronary artery disease (CAD) needing subclavian arteriographic screening before coronary artery bypass grafting (CABG). Lacey and Grosveld et al. concluded that an IAPD ≥ 20 mm Hg was suggestive of subclavian steal. However, robust collateralization to the upper extremity vascular bed as well as the possibility of bilateral or distal subclavian occlusive disease may moderate or cause discordant differential measurements between arms and alter the assumptive linear relationship between degree of stenosis and the IAPD.
Duplex scanning affords direct intrathoracic noninvasive assessment with simultaneous grayscale imaging and color flow Doppler mapping. In the noninvasive vascular laboratory, ultrasonic duplex evaluation of the extracranial vessels includes imaging and Doppler assessment of the carotid, subclavian, and vertebral arteries, though the deep proximal trunks are not adequately visualized with high-frequency linear probes. Nevertheless, the accuracy of this technique in detecting subclavian steal, frequently using reactive hyperemia, is impressive and widely used. Grosveld et al. elaborated a protocol for deep thoracic arch vessel duplex analysis with provocative ergonometric arm exercise testing to detect cerebrovascular ischemia in IA lesions ultimately confirmed angiographically. Takach et al. adapted this approach and described a technique to evaluate patients after operative and endovascular reconstruction in their excellent review of CSSS. Others rely on duplex ultrasound to monitor subclavian artery stent patency. Transesophageal echocardiography has been proved effective in establishing the relationship between cryptogenic stroke and extensive plaque in the aortic arch but has not been found to be reliable for investigating the arch vessel trunks for atherosclerotic lesions. Direct duplex imaging of the arch and its proximal branches can be assessed intraoperatively using epiaortic imaging. Transthoracic echocardiographic aortic arch imaging provides the ability to directly visualize the arch vessel takeoffs. In most cases, real-time echocardiographic duplex images supply useful information regarding branching patterns and complexity, plaque and mural characterization, location and length of stenosis, and Doppler hemodynamics.
Color flow Doppler imaging is an excellent complement to localize stenosis-induced flow disturbances and guide quantitative Doppler flow analysis. A comparison of color duplex arch vessel imaging in a series of patients with Takayasu’s arteritis was undertaken by Handique et al. Angiographic stenosis correlation to duplex determination was carried out for the entire array of arch vessels, with encouraging findings. The IA had the lowest correlation for stenosis grading (0.53), but sensitivity (86%–100%), specificity (92%–100%), and correlations (0.91–1.00) were high for the other arch vessels. Long-segment, circumferential intima-media thickening characteristic of Takayasu’s arteritis, described as the “macaroni sign,” was another helpful finding.
Many patients with arch vessel disease are asymptomatic and may not benefit from aggressive surgical or endovascular treatment options. Vertebrobasilar symptoms are nearly universally transient and self-limiting, rarely causing permanent neurologic insult. Peterson et al. did not treat asymptomatic arch vessel disease unless lesions were proved to be high grade (>90%). Others reaffirm that symptoms of cerebral ischemia in SAS and subclavian steal can be attributed to coexistent carotid artery disease. Their treatment paradigms assert that as these extracranial lesions are repaired first, good long-term outcomes and symptom relief will ensue. However, recent investigations have independently correlated an IAPD of 10 to 15 mm Hg or more in at-risk but asymptomatic community cohorts with significant increases in both all-cause and cardiovascular mortality. A 10-year study on patients without history of cardiovascular disease in the primary care setting found that an IAPD threshold ≥ 10 mm Hg or more was associated with a 2.6-fold increase (adjusted hazard ratio) in all-cause mortality and a 3.2-fold increase in combined cardiovascular events and death. These findings suggest that SAS and AAVD are not benign, and asymptomatic patients with mild or moderate disease would, at least, benefit from early detection with aggressive lifestyle modification and best medical therapy targeting reduction in cardiovascular risk.
Occlusive arch vessel disease is amenable to both surgical and endovascular techniques to restore flow and alleviate symptoms. Preventative or preservative reconstruction has been advocated for severely diseased common carotid and innominate vessels when cerebral circulation is in jeopardy, similar to the approach for extracranial arterial disease. Patients with debilitating hemispheric or vertebrobasilar symptoms have proven benefit from surgery or stenting. Subclavian interventions are also indicated for ipsilateral limb ischemic and embolic manifestations. Proponents of operative reconstruction in AAVD point to excellent long-term patency with acceptably low morbidity and mortality. Endoluminal adherents tout its less invasive nature, lower costs, fewer complications, better patient satisfaction, shorter hospital stay, elimination of general anesthesia, and good short-term and midterm results. Clearly, endoluminal treatment has been, of late, gaining momentum. Cervical, extrathoracic carotid subclavian bypass using Dacron (E. I. du Pont de Nemours & Company, Wilmington, DE), polytetrafluoroethylene or Gore-Tex conduits (W. L. Gore & Associates, Newark, DE), carotid-subclavian transposition, and other surgical approaches have set the standard by which all other treatment modalities are measured. Ten-year patency rates for these surgical procedures are 90%. Comparable with certain surgical approaches, endoluminal stenting has the theoretical advantage of preserving aortic inflow. Patient comfort and minimal access site complications have recently spurred interest in and application of the transradial approach for treating subclavian lesions. Lack of endoluminal procedural success in the proximal arch vessels is invariably due to impassable total occlusions leading to open revascularization in surgical candidates. Ultimately, the treatment for AAVD is largely dependent on the experience and expertise available in the institution.
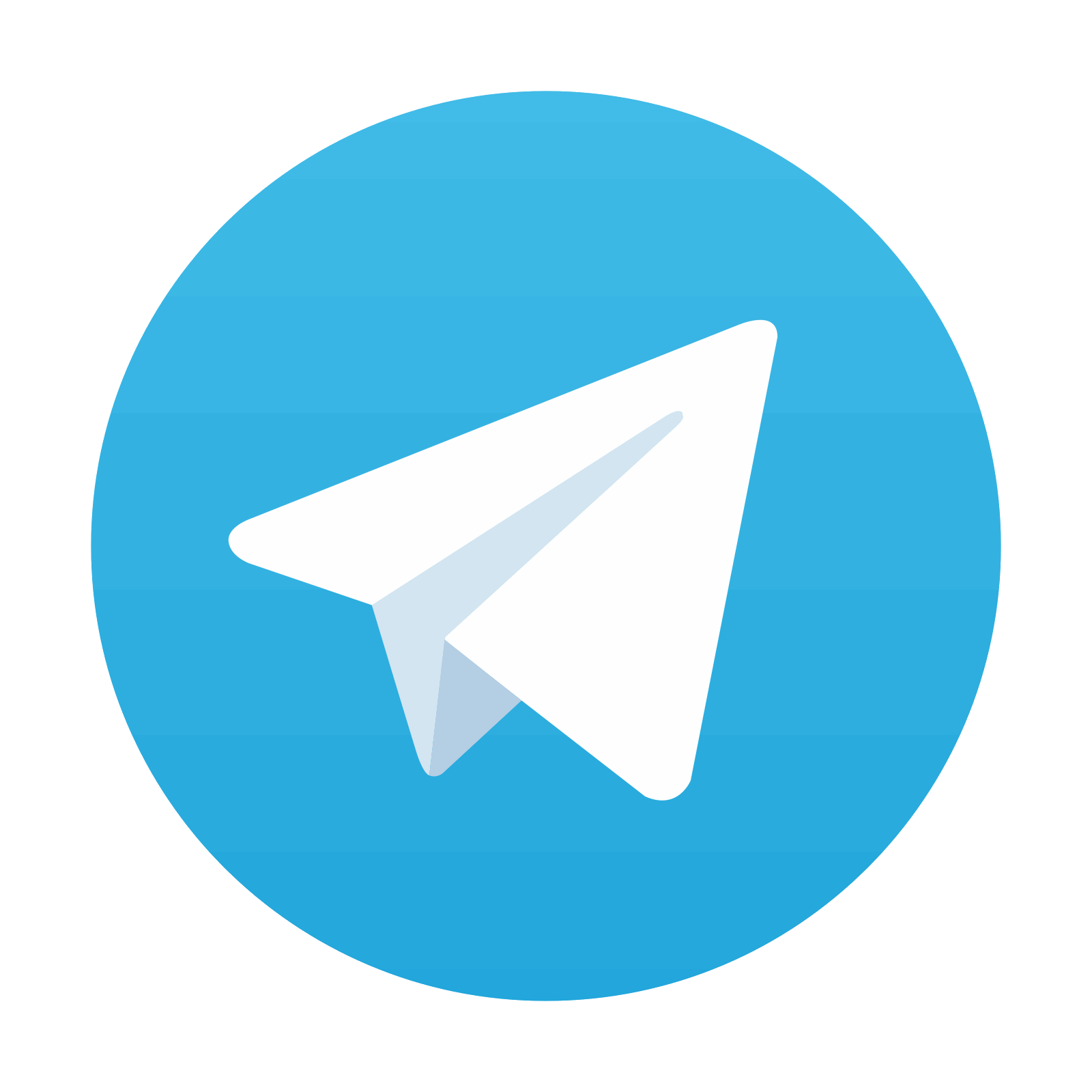
Stay updated, free articles. Join our Telegram channel

Full access? Get Clinical Tree
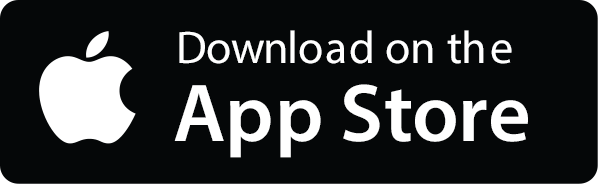
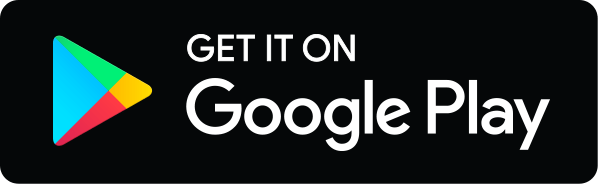