Fig. 40.1
Mechanism of action for tranexamic acid (TXA), e-aminocaproic acid (EACA), and aprotinin during fibrinolysis (t-PA tissue-type-plasminogen activator, u-PA urokinase type-plasminogen activator, PAI plasminogen activator inhibitor, FDP fibrin degradation products)
Studies showed that major components of the coagulation system could be detected in the fetus approximately from 10 weeks of gestation. Immediately after birth, the coagulation system is developed with its all parts, but the blood concentrations are different. Reference values are given by Andrews and were documented in a more recent study by Monagle [4, 6]. One major difference is the concentration of plasminogen, which is reduced by more than half the amount compared to adults. After 6 months of age, the adult concentration is nearly equal, and it remains constant during childhood. t-PA in contrast is significantly increased immediately after birth. Very short after birth the plasma concentration of t-PA is decreasing progressively. There is a rapid increase in t-PA levels after the first period of life, and t-PA levels will reach about 50 % of the adult value during childhood compared to adulthood. Like t-PA, the plasminogen activator inhibitor levels are increased after birth compared to adult values. This may explain why, despite the remarkable decrease in plasminogen levels, the activity of the fibrinolytic system in infants and very young children is increased compared to adults. The most important differences in the plasma concentration of coagulation molecules compared to that of adults are presented in Table 40.1.
Parameter | Day 1 | 3 month of age | 6 month of age |
Antithrombin (AT) | ⇩ | ⇘ | – |
Protein C | ⇩ | ⇘ | – |
Protein S | ⇩ | ⇘ | – |
Plasminogen | ⇩ | ⇩ | ⇩ |
t-PA | ⇧ | – | – |
PAI-1 | ⇧ | – | – |
PAI-2 | ⇧ | – | – |
α2-AP | – | – | |
α2-M | – | – | – |
Antifibrinolytic Agents for Children
Aprotinin
Aprotinin is a so-called “serine protease” molecule inhibitor when administered in large doses. Serine molecules can be found in the coagulation pathway and are needed for initiating fibrinolysis [10]. Aprotinin augments and prolongs coagulation initiated by activation of both intrinsic and extrinsic pathways. The main influence on the fibrinolytic system is mediated by blocking kallikrein and the plasmin-activating molecules i-PA, u-PA, and t-PA. Additionally, aprotinin, like other antifibrinolytic drugs, will interfere with the lysine-binding site of plasminogen or plasmin. The effect of t-PA is enhanced more than 400 fold in the presence of fibrin. Several studies showed that aprotinin decreases thrombin formation during surgery with cardiopulmonary bypass. Therefore, it is recommended to start with the aprotinin therapy before fibrin formation has been initiated, which means before the skin incision in major surgery [11].
There are numerous studies on the aprotinin dosage regimes, especially for cardiac or pediatric cardiac surgery. In recent years, “high-dose” schemes were mostly recommended [12].
e-Aminocaproic Acid (EACA)
EACA is not available in all European countries. Comparable to tranexamic acid, EACA reversibly inhibits the transformation of plasminogen to the active protease plasmin. Saturation of the lysine-binding site of plasminogen with EACA displaces plasminogen from the surface of fibrin. Even if plasminogen is transformed into the active component plasmin, it cannot bind to fibrin, and its fibrinolytic action is inhibited [13]. Over 20 years ago, there were reports that high-dose EACA therapy will inhibit the platelet aggregation induced by collagen and ADP [14]. Shortly after the introduction of EACA, serious concerns arose about thromboembolic and renal complications. After a warning message published in the New England Journal of Medicine in 1969, the routine use of EACA dropped, especially for children [15, 16]. A recent large multicenter study including a total of 22,258 patients (25 centers) looking at adverse events associated with aprotinin, TXA, and EACA found no difference in renal failure requiring dialysis in any of the groups. Interestingly, the study favored the TXA group, and comparative analyses suggested similar efficacy of EACA and improved outcomes associated with TXA.
Tranexamic Acid (TXA)
Tranexamic acid has been available for a long time. In Europe, it was introduced for clinical use starting in 1970.
Tranexamic acid is a synthetic derivate of the amino acid lysine that exerts its antifibrinolytic effect through the reversible blockade of lysine-binding sites on plasminogen, thus preventing fibrin degradation. Its main action in the coagulation system is promoting clot stability. Tranexamic acid also preserves platelet function by reducing the effect of plasmin on glycoprotein 1b receptors.
The optimal dosage for TXA to prevent fibrinolysis has not yet been defined for pediatric patients. According to in vitro studies, a concentration of 10 μg/ml can decrease 80 % of t-PA activity, and a concentration of 16 μg/ml can inhibit platelet activation induced by plasmin [17–19]. The concentration of TXA necessary to prevent fibrinolysis in vivo is unknown. A recent study aiming to optimize the dosing schedule in adult patients in cardiac surgery had the objective of achieving a stable plasma concentration >20 μg/ml.
Indication for Antifibrinolytic Agents in Cardiac Surgery
There are many publications on adult cardiac surgery that support the use of antifibrinolytic substances for high-risk patients because of the reduction in the amount of blood products transfused. Less information is available for pediatric open-heart surgery. Moreover, estimation of the effectiveness of the prophylactic use of antifibrinolytics varies a great deal. For example, the study by Davies et al. [20] showed a reduction in neither blood loss nor in the blood products used under aprotinin therapy. Williams et al. [21] also found no effects of aprotinin on newborns with congenital heart defects. However, for Carrel et al. [22] and more recently Murugesan et al. [23], aprotinin had a blood-saving effect in surgery for transposition of the great arteries. Other authors demonstrated a blood-saving effect for all antifibrinolytic agents during repair of cyanotic defects [24]. In conclusion, complex neonatal cardiac surgery, cyanotic defects, and rethoracotomies constitute the three reported indications for using antifibrinolytic drugs for pediatric open-heart surgery. An up-to-date, large multicenter study including a total of 22,258 patients (25 centers) looking at blood saving aspects and adverse events associated with aprotinin, TXA, and EACA found no difference in the rates of renal failure requiring dialysis in any of the groups. The blood-saving aspects of all of the used substances were comparable. Interestingly, the study favored the TXA group, and comparative analyses suggested similar efficacy of EACA and improved outcomes associated with TXA [25].
Dosage and Infusion Schemes of Tranexamic Acid
Up to now, no consensus has been reached about the ideal dosage of TXA for pediatric patients undergoing congenital heart surgery with cardiopulmonary bypass (CPB). When analyzing the existing studies regarding the dosage and efficacy of TXA, it should be noticed that in most of the studies the age range was large, including neonates, infants, children, and adolescents, which makes the results analysis difficult, especially as the results are usually not stratified by age. Additionally, it is important whether the patients are suffering from a cyanotic or non-cyanotic disease because cyanotic heart defects are known to involve platelet dysfunction, and these patients tend to bleed more than non-cyanotic patients. Second, if analyzing efficacy studies, the main clinical endpoints could be blood loss volume, transfusions requirement, coagulation parameters, or a combination of these. Some other endpoints are also found in studies, such as time needed for chest closure, rethoracotomies, platelet activation analysis, and biochemical parameters. Of note, the endpoint blood product transfusion volume may be biased in low weight children since blood products may also be used for hemodynamic reasons and do not always consequently indicate perioperative blood loss. Additionally, in low body weight children, blood must be administered if platelets or fresh frozen plasma have to be given to prevent dilution and therefore low hematocrit values.
Chauhan and coworker could show the efficacy of TXA on blood loss and blood product requirements in three out of four groups with different dosage schemes except one single bolus application of 50 mg/kg [26]. In the four study groups, they have used: group 1, 50 mg/kg TXA at induction of anesthesia; group 2, 10 mg/kg at induction followed by an infusion of 1 mg/kg/h; group 3, 10 mg/kg at induction, 10 mg/kg on bypass, and 10 mg/kg after protamine (end of surgery); group 4, 20 mg/kg at induction and 20 mg/kg after protamine (end of surgery). These results could explain the absence of any significant reduction in the use of blood products in two Canadian studies of Levin and Zonis because they also used a single bolus injection of 50 mg/kg BW [16, 27]. Van der Staak and coworker used TXA continuously in major pediatric surgery. They found a significant reduction in postoperative blood loss and transfusion requirements with a dosage scheme of 4 mg/kg 10 min prior to skin incision followed by an infusion of 1 mg/kg/h for 24 h after surgery [28]. Bulutcu could show a significant decrease in blood loss and blood product requirements with TXA compared to placebo, especially in cyanotic patients [29]. The reported using a high dose scheme of one single bolus of 100 mg/kg followed by 100 mg/kg in the pump prime and 100 mg/kg after CPB. If comparing this high-dose scheme with that of Chauhan, no significant difference could be seen regarding the primary endpoints, allowing the conclusion that the efficacy does not increase dose dependently. Because even in the presence of heparin during cardiopulmonary bypass fibrin formation and therefore activation of coagulation could be assumed, a continuous dosage regimen seems favorable over a single-bolus injection. The most recent regimen used at our institution is based on our own experience [30] and was later modified after pharmacokinetic calculation according to the in-vivo studies of Andersson and Soslau [17, 19]. We now use a single bolus of 10 mg/kg BW followed by a continuous infusion of 3 mg/kg/h, adding 0.1 mg/kg TXA per ml of pump prime volume to the cardiopulmonary bypass circuit.
Timing of Antifibrinolytic Therapy in Pediatric Heart Surgery
Most of the study protocols using antifibrinolytic therapy are focused on different dosage schemes. Considering the mechanism of fibrinolysis and especially antifibrinolytic therapy, the drugs act by binding to the lysine-binding site of plasminogen by interfering with the tissue plasminogen activator. Remember that the potential of t-PA is enhanced more than 400 fold in the presence of fibrin. This means that the activation of the fibrinolytic system must be suppressed before a significant amount of fibrin or fibrin clots have been generated. In the setting of congenital heart surgery, it is therefore considered to be effective to start any antifibrinolytic therapy before skin incision. Data from the available literature do not support the mandatory initiation of antifibrinolytic therapy after skin incision or sternotomy, but strongly suggest starting before the beginning of CPB. Additionally, the initiation of a cardiopulmonary bypass as well as the reversal of heparin to start coagulation with administration of protamine is associated with a significant activation of the coagulation cascade. If not, a2-antiplasmin will immediately begin degrading plasmin, and a reduction of a2-antiplasmin is associated with the risk of excessive bleeding [31–34].
Risks and Side Effects
The aforementioned observational study by Mangano et al. published in 2006, observed an increase in the number of cases of renal failure, myocardial infarction, and stroke under aprotinin therapy [2]. In 2007, Mangano, using the same set of data, found an increase in the 5-year mortality rate, which was not the case in the tranexamic acid or in the ε-aminocaproic acid groups [35]. The criticism was that the aprotinin group was at a disadvantage in these observational studies because the high-risk constellation of the patients was countered in 2008 by the data of the prospective, randomized study by Fergusson et al. [3]. This study had to be prematurely abandoned as a distinct trend toward mortality was established. In his publication, Fergusson stated that for aprotinin, the “number needed to harm” is 50. However, in two other more recent studies with a large number of pediatric cardiac surgical patients, Szekely et al., with nearly 700 children, were not able to show that aprotinin was responsible for renal dysfunction [36], and Backer et al., with over 2,500 children, were not able to establish a connection between aprotinin and renal insufficiency, neurological complications, or an increase in mortality [37]. The question is to what extent the data compiled by Mangano and Fergusson in adult surgery can be transferred to the field of pediatric surgery. It is to be noted that in some recent studies, while aprotinin reduced the tendency to bleeding, it was not shown to influence the rate of mortality or to impair organ function. However, the meta-analysis of Brown et al. [38] states that the risks of using aprotinin outweigh the risks of using tranexamic acid, and the authors of a new issue of the Cochrane collaboration overview came to a similar conclusion.
< div class='tao-gold-member'>
Only gold members can continue reading. Log In or Register a > to continue
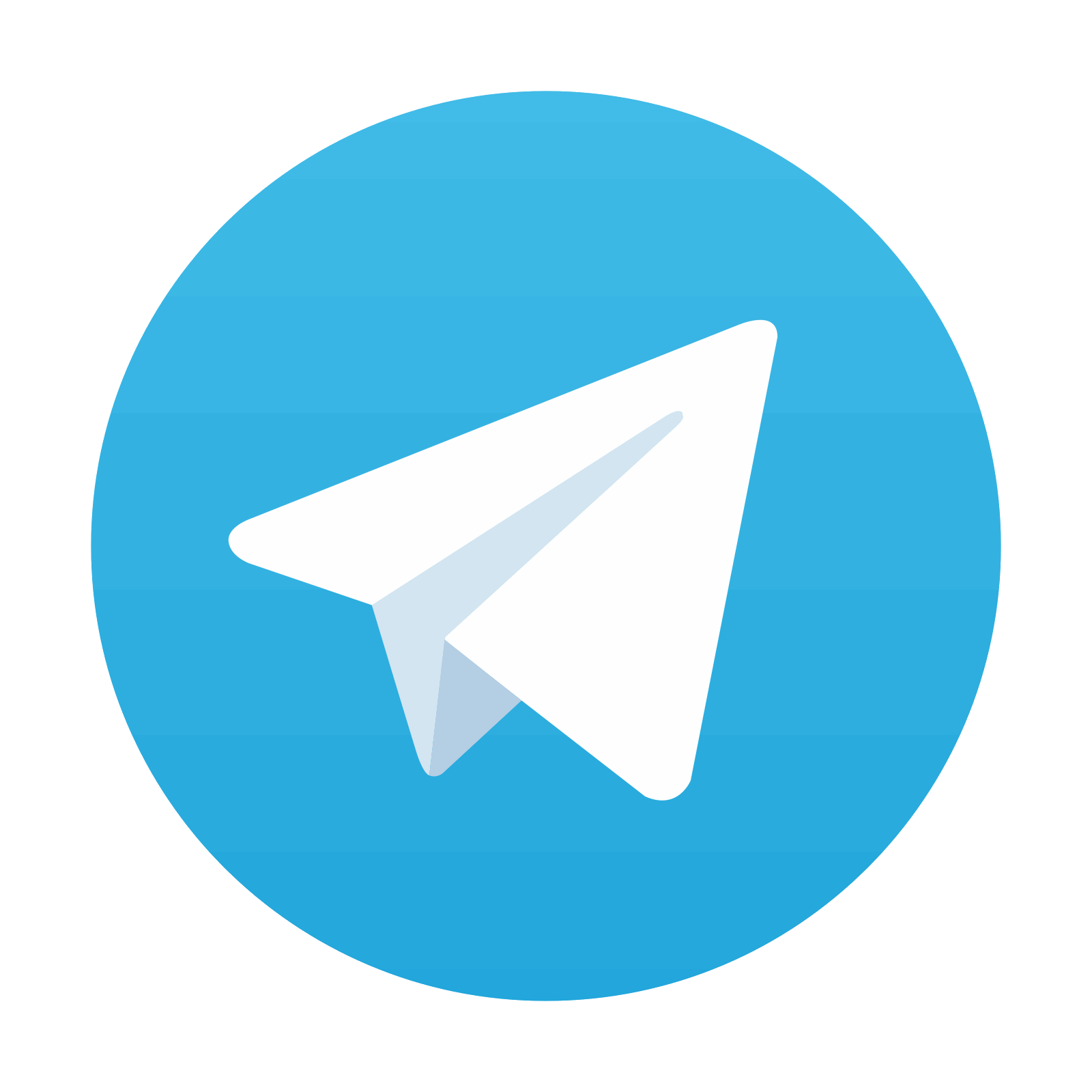
Stay updated, free articles. Join our Telegram channel

Full access? Get Clinical Tree
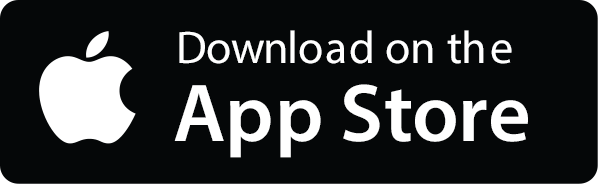
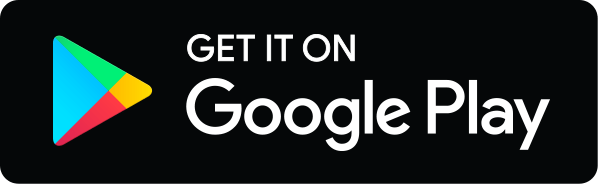