Fig. 22.1
Interplay of Donor and host immune responses in cardiac AMR
B cells are critical players in the pathogenesis of the antibody mediated immune response. B-cell immunoglobulin protein receptors are uniquely coded to a specific antigen. When the antigen is encountered in its native form (not in association with MHC antigens as required by T cells), the B cell can undergo activation to specific plasma cells and memory cells. T-cell dependent activation requires a co-stimulatory signal from a corresponding specific Th2 cell, a T helper cell, that secretes cytokines leading to clonal expansion and differentiation into plasma cells and memory cells. With T-cell independent antigens, the activation signals of antigen recognition and co-stimulation are both provided by the antigen. In some cases the co-stimulatory signal is supplied by TLR receptors on cells such as endothelial cells. T-cell independent antigen stimulation results in differentiation of B cells into Immunoglobulin M producing plasma cells, exclusively. Fully activated B lymphocytes of either type possess surface markers CD 19, part of the antigen receptor, and CD 20, a marker of complete activation, particularly important in T-cell independent antigen recognition. Activated B cells can produce small quantities of specific immunoglobulin and those responding to T-cell dependent antigens can undergo immunoglobulin class switching [52–54].
Plasma cells are end stage cells that produce large quantities of an antigen specific antibody of a defined immunoglobulin class. They are incapable of antigen recognition and do not possess the usual B-cell markers CD19 and CD20. They do possess other surface markers including CD27, CD 38 and CD 78 and IL-6 receptor. They lack the common leukocyte antigen, CD45 [55–57].
The other important effector cells of the antibody adaptive immune response are macrophages, neutrophils, platelets and NK Cells, which possess Fc receptors for immunoglobulin as well as TLR receptors and complement receptors. These effector cells, while not possessing specific antigen recognition properties, are stimulated through these cell surface receptors to produce specific proteins that act as effectors of the innate immune response, chiefly through the coagulation, kinin and complement cascades [41, 44, 47, 58, 59].
In AMR, the principal site of immune interaction is the vascular endothelium. Endothelial cells function to present antigen and act as co-stimulatory cells to T cells. They possess TLR receptors that allow them to respond to injury associated molecular profiles created during allograft injury or ischemia. This interaction leads to endothelial activation and cytokine release which triggers inflammation, dendritic cell activation, and also lymphocyte activation. Endothelial cells express CD40 and other molecules which can contribute to T-cell activation (CD58; CD134 ligand, ICOS ligand). CD 40 is critical in providing cognate T-cell help for B-cell Ig production and class switching. CD 40 and its ligand have been demonstrated in cardiac allografts undergoing rejection [59–61].
Antibodies produced against MHC and other antigens released during tissue injury bind to MHC antigens on the vascular endothelium. These antibodies may or may not fix complement which can accelerate the level of injury of the endothelium. Importantly, the antibodies also can bind immune cells with receptors for the Fc portion of the immunoglobulin molecule, including macrophages, NK cells, B lymphocytes, neutrophils, and platelets, which further accelerate allograft injury [62–65].
In summary, the reaction of the host to an allograft is a complex interplay of factors involving both the innate and adaptive immune responses. The centerpiece of these reactions is the endothelium of the microvasculature which acts both as an active participant and the target of the destructive elements of this response. If the vascular endothelium is repeatedly injured by these processes, the graft will experience ischemic damage that either eventuates in global myocardial damage and heart failure or allograft coronary artery disease and graft loss [66, 67].
Pathology of Cardiac AMR
In 2005, a consensus conference was held at the National Institutes of Health concerning AMR in solid organ allografts, which led to a concerted effort to standardize the definition of AMR in hearts and the approach to diagnosis [8, 29].The ISHLT 2005 guidelines recommended diagnosis of AMR based on interpretation of pathologic changes in conjunction with cardiac allograft dysfunction and/or hemodynamic compromise and the presence of DSA [29]. AMR has since been recognized to exist along a spectrum from an asymptomatic phase with occurrence of DSA in isolation to a symptomatic phase with allograft dysfunction and hemodynamic compromise. This is a clinically useful distinction as asymptomatic AMR has been shown to be associated with poorer outcomes [1, 3, 17, 18, 68, 69]. Furthermore, a universally accepted definition for allograft dysfunction does not exist. The ISHLT 2005 working formulation for cardiac AMR was further refined in 2013. For the remainder of this section, the pathologic features published in 2013 will be described and illustrated. Table 22.1 contrasts the recommendations across the three versions of the ISHLT guidelines. A key difference between the 2005 and 2013 ISHLT guidelines is the proposed shift from a clinical to pathologic diagnosis [15, 16].
1990 grading schema [70] | 2005 grading schema [29] | 2013 grading schema [16] | |
---|---|---|---|
Required samples | Four pieces of myocardium | Three pieces of myocardium | Three pieces of myocardium |
Sample type to be used for IHC | Frozen tissue should be saved for potential IF to detect ‘vascular’ rejection | Frozen tissue or tissue fixed for routine processing could be used for detection | Same as 2005 |
IHC surveillance | Optional | Only in biopsies suspected histologically to be positive | 2 biopsies in first month; then at 1, 3, 6 and 12 months |
Histopathology of AMR | Vasculitis, edema without cellular infiltrate | Edema, capillary swelling, intravascular macrophages or neutrophils, interstitial edema | Same as 2005 |
IHC, IF method | IF optional with IgG, IgM, C3 scoring as additional information | IgG, IgM, IgA, C3d and/or C4d or C1q, fibrin | C4d and/or C3d required; IgG, IgM, fibrin optional; HLA for capillary integrity assessment |
IHC, IP method | None | IP for CD68 or C4d and CD34 or CD31 to define capillaries | IP for CD68 and C4d; CD31, CD 34 optional to define capillaries; C3d optional |
AMR scoring | Humoral Rejection | AMR positive (AMR1) if positive histology, IHC, graft dysfunction, and DSA | pAMR 1–3 based only on histology and IHC |
DSA | None required | Required for AMR positive (AMR1) | Should be checked with biopsy, but not required for pAMR diagnosis |
Follow up of AMR | None required | None required | After 2 weeks until negative by IHC |
Specimen Handling Considerations
Specimen requirements for cardiac biopsy interpretation were standardized in the first ISHLT grading schema. At least four biopsy fragments of viable non-scarred myocardium are necessary to ensure an adequate sample for interpretation. Early studies of AMR in cardiac allografts used frozen tissue samples obtained at the time of routine endomyocardial biopsy. Such samples were considered highly desirable for this purpose in the original ISHLT grading schema because they obviate the artifacts associated with routine formalin fixed paraffin embedded (FFPE) tissue processing which includes tissue fixation, dehydration and lipid extraction associated with xylene infiltration prior to paraffinization. Newer experience suggests that these processing issues can be surmounted by using other fixatives and different immunohistochemical methods. The recent updating of the ISHLT schema for cardiac allografts allows for either frozen or FFPE samples to be used. Reagents and procedures vary depending on the sample type [70] (Table 22.2).
Histologic features | IF features | IP features | Comments | |
---|---|---|---|---|
pAMR 0 | No suspicious findings | No findings | No findings | No findings |
pAMR 1 (H+) | Capillary endothelial swelling or denudation; intravascular accumulation of macrophages or neutrophils; interstitial edema | Negative | Negative | May see weak IF or IP staining that does not qualify as positive |
pAMR 1 (I+) | None or focal features as above | Diffuse or multifocal intense staining with C4d or C3d in capillaries | Diffuse or multifocal intense staining of capillaries with C4d and CD 68 if used | Either IF or IP features qualify as positive; Intensity is similar to controls |
pAMR 2 | Capillary endothelial swelling or denudation; intravascular accumulation of macrophages or neutrophils; interstitial edema | Diffuse or multifocal intense staining with C4d or C3d in capillaries; strong HLA staining of capillaries | Diffuse or multifocal intense staining of capillaries with C4d and CD 68 if used | Either IF or IP features qualify as positive |
pAMR 3 | Capillary endothelial swelling or denudation; intravascular accumulation of macrophages or neutrophils; interstitial edema; intravascular thrombi; myocyte necrosis without cellular rejection; interstitial tissue debris | Diffuse or multifocal interrupted staining with C4d or C3d in capillaries; weak staining of HLA ; fibrin accumulation; C3d or C4d autostaining of necrotic myocytes | Diffuse or multifocal intense staining of capillaries with C4d and CD 68 if used; CD31 or CD34 staining of capillaries demonstrates damaged walls or capillary loss | Histologic features hard to distinguish from severe rejection (ISHLT 3R) |
Histopathologic Features of Cardiac AMR
In acute AMR, there are well defined histopathologic features predominantly involving the capillaries (Fig. 22.2a–c). The capillaries are swollen with enlarged endothelial cells possessing obvious features of activation by electron microscopy [32]. The endothelial cells can occlude the lumina. Often there are also adherent macrophages and/or neutrophils in the capillaries that cause the vessels to have a cellular, rope like character. In later biopsies of AMR when capillary damage has been prolonged, the capillaries may appear discontinuous or even absent, giving a faulty innocuous appearance. In such cases, there is often significant interstitial edema manifested as widening of interstitial spaces, often with a bluish color. As this process continues unabated, the capillaries disappear, the edema dissipates and the process becomes very difficult to distinguish from a negative biopsy without evidence of any acute rejection. Two groups have documented that the histopathologic features do not correlate well with the immunopathologic features [71, 72].


Fig. 22.2
Pathology of Cardiac AMR. (a) Endomyocardial biopsy showing obvious adherence of macrophages to swollen endothelial cells throughout the fragment (hematoxylin and eosin (H&E) stain, 10×). Immunofluorescent (IF) staining for C4d was negative. Diagnosis: pAMR 1 (H+). (b) Endomyocardial biopsy shows prominent intravascular macrophages, neutrophils and prominent endothelial cells (H&E stain, 20×). IF staining was strongly positive for C4d (panel e). Diagnosis: pAMR 2. (c) Endomyocardial biopsy from patient with persistent AMR over several weeks. Capillaries are swollen with adherent macrophages. Interstial regions are edematous and contain cellular debris as well as more macrophages (H&E stain, 20×). IF staining was positive for C4d and fibrin (panel f). Diagnosis: pAMR 3. (d) C4d on frozen section of endomyocardial biopsy from patient without significant histologic features of AMR on the current biopsy, but obvious AMR on previous biopsies. Strong linear stained capillaries are seen throughout the section (IF stain, 10×). Diagnosis: pAMR 1 (I+). (e) C4d on frozen section of endomyocardial biopsy from patient with obvious histologic evidence of AMR (panel b). Round capillary profiles show intense staining (IF stain, 10×). Diagnosis: pAMR 2. (f) Endomyocardial biopsy (same patient, panel C) showing fibrin intensely stains swollen capillary profiles and extends into the neighboring interstitium (IF stain, 20×). Diagnosis: pAMR 3. (g) Immunohistochemical (IHC) stain for C4d from patient suspected of having AMR on the basis of prior history of AMR. Tissue previously fixed in formalin was used and shows strong brown outlining of capillaries. Staining of similar intensity was found in the control biopsy (immunoperoxidase (IP) stain, 10×). Diagnosis: pAMR 1 (I+). (h) IHC stain for CD68 to highlight macrophages from the biopsy shown in panel G. Strongly stained brown macrophages are scattered throughout the capillaries (IP stain, 20×). Diagnosis: pAMR 1 (I+). (i) IHC stain for CD34, a marker of activated endothelial cells and platelets. Capillaries have ragged profiles which extend into the interstitial spaces suggesting neovascularization. Patient had three previous episodes of AMR during the first year post-transplant (IP stain, 10×). Diagnosis: pAMR 1 (I+)
Immunopathologic Features of Cardiac AMR
To diagnose AMR, biopsies must be subjected to immunohistochemical (IHC) or immunofluorescence (IF) staining to detect immune reactants of AMR, especially complement components. By immunohistochemistry on FFPE prepared samples, macrophages are detected by CD 68 staining, capillary damage is defined using CD 34 or CD 31, and C4d is detected in capillaries [73] (Fig. 22.2g–i).
There is less agreement about the significance of the distribution of the C4d staining (all capillaries or just focal areas) and the intensity of C4d staining provided on a semi-quantitative scale. More studies must be done to correlate various patterns of staining with patient outcomes in order to see what patterns are most significant. C3d can be sought by immunohistochemistry, but no large studies have been published on the value of this staining method.
By IF staining, C4d and/or C3d can be detected in capillaries and issues of distribution and intensity are significant (Fig. 22.2d, e). One group has published that both C3d and C4d are needed to accurately diagnose AMR by IF. This result is controversial. Other IF reactants which can expand the diagnostic accuracy include IgG or IgM and HLA-DR which is upregulated on capillaries in acute AMR and downregulated when AMR becomes severe and longstanding. Detection of HLA DR by IF is optional. Evaluation of IF staining for fibrin is also optional. Fibrin staining has been shown by at least two groups to correlate with severity of AMR and with poor outcome [75–78] (Fig. 22.2f).
Outstanding Pathology Controversies
The most vexing issue of cardiac transplant pathology interpretation is the interplay of factors which can affect the histologic and immunopathologic findings as previously described. Pathologic examination relies on the expression of proteins and cells in a tiny sample of the heart transplant tissue. Traditional pathologic monitoring relies on interval biopsies which are morbid and prone to artifacts of sampling, interpretation, sample handling, and test method selection.
Fortunately, the genes and proteins involved in these complex interactions have been defined in experimental systems and molecular and proteomic methods are emerging to allow monitoring in vivo. The interplay between serum and tissue sampling will also require definition [79–82].
Molecular profiling using non overlapping pathogenesis based transcripts (PBT) have been successfully used to complement pathologic assessment of renal transplant biopsies and certain transcripts have shown high correlation with histopathology, DSA activity and poor outcome in renal AMR patients [79]. The transcript sets each define relevant biologic events in transplantation such as parenchymal injury, endothelial activation, infiltration by T cells or macrophages, and gamma interferon induced inflammatory events. The transcript sets were independently developed in experimental systems and tested in human transplant renal biopsy tissue. When the same approach was applied to cardiac transplant biopsies, expression of transcript sets reflecting T-cell and macrophage infiltration, and y -interferon effects correlated strongly with each other and with transcripts indicating tissue/myocardium injury. This molecular phenotype correlated with Quilty (p < 0.005), capillaritis (p < 0.05) and decreased left ventricular ejection fraction (LVEF) (p < 0.007), but not with the histologic diagnosis of acute cellular rejection. The study was done prior to the ISHLT grading schema characterizing AMR but capillaritis is a reasonable correlate of AMR. Further work is needed to validate this interesting study [79, 82].
A recent pilot study using plasma proteomics has also been reported in renal transplant patients, providing evidence that protein concentrations in plasma may provide a relevant measure for the occurrence of biopsy-proven acute rejection and offers a potential tool for immunologic monitoring [83].
Until markers of these diverse processes are available, we will have to rely on careful clinical-pathologic correlation among providers. Hopefully, molecular profiling based on discrete transcript sets and proteomic monitoring will eventually solve this conundrum.
Clinical Features of Cardiac AMR
Incidence/Prevalence
The true incidence of cardiac AMR has not been well documented in the absence of routine screening in asymptomatic patients. It is therefore likely that it had been underreported prior to 2011. Much of the literature instead describes the prevalence of symptomatic AMR. The prevalence of lone symptomatic AMR ranges between 10 and 15 %, whereas the prevalence of AMR when routinely surveilled (symptomatic and asymptomatic) or when diagnosed concurrently with acute cellular rejection (mixed rejection) may surpass 40 % [10, 84–86]. This wide range is also the result of the lack of prior standardized diagnostic criteria as well as the diversity of the populations studied in their predisposition to AMR.
Time to Occurrence
Observational studies indicate that cardiac AMR tends to become manifest early after transplantation [2, 3, 77, 84, 87]. Additionally, the risk of recurrence is higher in heart transplant recipients who have three or more episodes of AMR in the first 3 months post-transplant, and repeat episodes of AMR are usually clustered soon after the first episode occurs. AMR can occur late after heart transplantation, though less commonly, and is usually associated with de novo DSA and poor outcomes [67, 88]. It is difficult sometimes to separate early from late AMR since most reports of late symptomatic AMR come from transplant programs that do not routinely screen asymptomatic patients earlier on.
Presentation
Most episodes of cardiac AMR are mild in severity and perhaps silent [87]. When clinically manifest, there are no signs or symptoms specific to AMR. Instead, patients typically present with congestive (shortness of breath, dyspnea on exertion, cough) or low-flow (fatigue, exercise intolerance) symptoms that are common to overt heart failure from any cause. Signs on physical exam will vary depending on the patient’s acuity and whether a single or both ventricles are involved. A lower than usual blood pressure, an elevated central venous pressure, or a new S3 gallop are all warning signs that mandate immediate attention and further diagnostic investigation, preferably in the hospital. Signs and symptoms can be subtle and mild in a stable out-patient or severe and catastrophic in another with cardiogenic shock. Unlike for acute cellular rejection, patients with AMR are more likely to present with allograft dysfunction, hemodynamic compromise, or heart failure [1, 2, 4, 6, 13, 14, 89, 90].
On echocardiogram, besides systolic dysfunction, AMR may be associated with abnormal diastolic function (Fig. 22.3), increased left ventricular mass [91]. Findings on 12-lead electrocardiogram are also non-specific but we have found diffuse low QRS voltage on presentation to be invariably associated with fatal acute AMR. Blood tests abnormalities are typically related to the patient’s other co-morbidities and circulatory stability in general. Non-invasive methods including biomarkers to detect rejection may be abnormal with acute AMR, but to date none can exclusively be used to reliably diagnose it or rule it out. As such, cardiac AMR remains a pathological diagnosis on endomyocardial biopsy.


Fig. 22.3
Diastolic abnormalities on echocardiogram. (a) Pulse wave Doppler of the mitral valve inflow in a patient with acute AMR. Increased E/A ratio. The Mitral Valve Deceleration Time measures 61 msec. (b) Tissue Doppler of the septal mitral valve annulus showing reduced e’ velocity and reversal of e’/a’ ratio
Outcomes
The chances of pathological resolution of asymptomatic cardiac AMR when left untreated are related to its severity and time of occurrence since transplant (Fig. 22.4). Milder AMR episodes and those taking place beyond 12 months post-transplant are more likely to resolve or improve on follow up biopsies [87]. Even then, asymptomatic cardiac AMR tends to recur and has been linked to a higher risk of CAV [18] and cardiovascular mortality [92] (Fig. 22.5).



Fig. 22.4
Outcome of the Initial Antibody-mediated Rejection (AMR) on Next Follow up. (F/U) Endomyocardial Biopsy (EMB) (Reprinted from the Kfoury et al. [87], with permission from Elsevier) *AMR status was not checked for or reported in a few follow-up EMBs hence the small differences in totals

Fig. 22.5
Kaplan-Meier survival curves by rejection pattern (Reprinted from the Kfoury et al. [92], with permission from Elsevier) CR cellular rejection, MR mixed rejection, AMR antibody-mediated rejection
Definitive therapeutic success with acute cardiac AMR is difficult to gauge given the limited experience and the lack of standardized approach. Better odds of recovery appear to be related to timely and aggressive intervention. The availability of newer agents specifically targeting various steps of the humoral immunologic response allows for a multi-target approach that may be both more effective and safer.
Besides the immediate clinical challenges that acute AMR poses, patients who develop a pattern of repeated AMR have a many fold higher risk of cardiac allograft vasculopathy, chronic cardiac dysfunction, and mortality [3, 7, 9, 17, 68, 89]. An accelerated form of diffuse coronary artery disease developing over weeks has been associated with AMR and notoriously portends very poor outcomes [93]. The nature of the mechanistic link between microscopic AMR and macroscopic CAV remains to be fully elucidated. It is also conceivable that AMR and CAV are part of one pathological-clinical continuum.
Treatment of Cardiac AMR
The diagnosis of AMR is made by pathological findings. The decision of whether to treat and with what requires careful consideration and will take into account the patient’s clinical presentation, biopsy grade, allograft function, and the presence of donor-specific antibodies (Fig. 22.6). General recommendations for the treatment of AMR were published by the ISHLT in 2010 [94]. As more is learned about the pathophysiology and consequences of AMR, additional therapeutic strategies are being tried. The alloantibody response is complex, however, and caution needs to be taken as these new treatments have potential toxicities and disruptive effects on the modulating action of the immune response [95]. Ultimately, there is great need for randomized controlled trials in the prevention and treatment of AMR.


Fig. 22.6
Determinants of the likelihood to treat cardiac AMR [87]. + likely, ++ more likely, +++ yes, ISHLT international society for heart and lung transplantation, H histopathology, I immunopathology, DSA donor-specific antibodies, N/A not applicable
ISHLT Guidelines1 [94]
Class IIa
- 1.
The following treatments can be used to disrupt the immune-mediated injury of the heart allograft in AMR: (1) high-dose intravenous (IV) corticosteroids; (2) cytolytic immunosuppressive therapy.
- 2.
The following treatments may be used to remove circulating anti-HLA antibodies or decrease their reactivity: (1) plasmapheresis; (2) immune apheresis (immunoadsorption); (3) IVIg.
- 3.
The following treatments are used to maintain adequate cardiac output and systemic blood pressure: (1) IV inotropes and vasopressors; (2) mechanical circulatory support.
- 4.
When AMR is suspected, EMB examination should be expanded to include immunohistochemistry stains for complement split products and possible antibody.
- 5.
Recipient serum should be screened for presence, quantity and specificity of anti-donor (HLA) antibodies.
- 6.
Follow-up EMB should be performed 1–4 weeks after initiation of therapy and include immunohistochemistry examination.
- 7.
Adjustment of maintenance immunosuppressive therapy may be considered. This can include increase in the dose of current immunosuppressive agent(s), addition of new agent(s) or conversion to different agent(s).
Class IIb
- 1.
Systemic anticoagulation may decrease intravascular thrombosis in the heart allograft.
- 2.
Emergent retransplantation may be considered if the above measures do not restore acceptable heart allograft function, but outcomes in this situation are unfavorable.
Therapeutic Targets: Current and Future
T-Cell Inhibition
B-cell activity, including activation, isotype switching, and antibody production, is tightly regulated by T cells, therefore therapy targeting T-cell activity is an important aspect of treatment for AMR. Antithymocyte globulin (ATG) is prepared by immunizing rabbits or horses to macerated human thymus which contains predominately T cells, but also B cells, plasma cells, and dendritic cells, resulting in multiple antilymphocyte immunoglobulins. Various preparations are available and include rabbit antithymocyte globulin (rAThG), rabbit anti-T cell globulin (rATcG), equine antithymocyte globulin (eAThG), and equine antilymphocyte globulin (eALG). rAThG is the most commonly used preparation in the United States. ATG is very effective at killing or modulating T cells and is used as an induction agent and for treatment of cellular rejection [94, 96–100]. In addition to T-cell targets (CD3, CD4, CD8, and T-cell receptors), ATG contains antibodies to B-cell and plasma cell antigens and antigens expressed on both T and B cells, including CD20, CD30, FcR, CD126, and CD138 [101–103]. ATG may therefore be effective in the treatment of AMR beyond its inhibition of T cells via B-cell apoptosis, blockade of cytokine receptors, and binding of inhibitory receptors. It is generally used in moderate to severe cases of AMR associated with graft dysfunction and/or hemodynamic compromise. A usual course of treatment is between 3 and 7 days, and a CD3-count guided strategy may result in lower adverse events [103, 104].
The primary effect of calcineurin inhibitors (tacrolimus, cyclosporine) is inhibition of T-cell activation; there is no conclusive evidence for activity on T-cell-independent B-cell antibody production. Based on data showing less rejection in patients treated with tacrolimus/mycophenolate mofetil (MMF) versus cyclosporine/MMF, some advocate changing from cyclosporine to tacrolimus in a patient with AMR [105]. Rapamycin is an mTOR inhibitor that inhibits T-cell activity by blocking transcription of IL-2. Unlike the CNIs, rapamycin may also inhibit B-cell antibody production and proliferation via T-cell independent mechanisms [106, 107]. In clinical studies, rapamycin has been associated with decreased incidence and slower progression of cardiac allograft vasculopathy [108–110], a process likely related to AMR-mediated mechanisms. Changing from MMF to rapamycin or the addition of rapamycin is a reasonable strategy in a patient with AMR.
B-Cell Depletion/Inhibition
The spleen is the largest lymphoid organ in the body and houses antibody-producing memory B cells and plasma cells [111]. Splenectomy has been successfully used as a rescue therapy in cases of severe AMR in kidney transplant recipients refractory to standard therapy [112–114], however data for its use in heart transplant patients is lacking. When used, it is often combined with other B-cell targeted therapies to achieve maximal response.
B-cell depletion is also achieved with the administration of antibodies which bind antigens expressed on the surface of B cells. Agents used include the anti-CD52 antibody alemtuzumab as well as antithymocyte globulin, both of which deplete T cells in addition to B cells [100, 115–118]. Recently, the anti-CD20 antibody rituximab has been increasingly used. CD20 is not present on pro-B cells or mature plasma cells, thus it effectively eliminates peripheral B cells, but does not prevent the regeneration of B cells from precursors and may not directly decrease immunoglobulin levels. Research and therapeutic interest in rituximab has been greatest in the kidney transplant field [119–121]. A few case reports and series describe the use of rituximab in the treatment of AMR in cardiac transplant recipients, always as part of a multi-therapy regimen; therefore it is difficult to determine the exact efficacy of rituximab [90, 122–125].
Additional B-cell depleting agents developed for the treatment of lymphoma and autoimmune diseases and which may be useful in transplantation include the anti-CD20 antibodies ofatumumab and ocrelizumab, which are potentially more potent, have a lower risk for immunogenicity, and have less complement activation [126–130] CD22 is an inhibitory receptor that remains present as B cells mature and after expression of CD20 is lost. Epratuzumab is an anti-CD22 antibody shown to reduce B-cell numbers as well as inhibit their activation and proliferation [131]. Anti-CD19 antibodies are being developed and have the added advantage of acting against B cells and plasma cells [132].
Agents that target co-stimulatory receptors on the surface of B cells may also have therapeutic benefit in AMR. BAFF is a costimulator critical for B-cell differentiation, survival and expansion; naive B cells in particular are sensitive to BAFF depletion. Belimumab and atacicept target BAFF and have shown potential in the treatment of systemic lupus erythmatosus [133, 134]. Co-stimulation ligand receptor pairs, such as CD40-CD154, present on T and B cells are essential for activation. Anti-CD154 antibodies were promising but failed in clinical trials due to thrombotic complication [135–137]. Unlike CD154, CD40 is not expressed on platelets and may not have the same thrombotic risk. Anti-CD40 antibodies are being tested in autoimmune diseases and transplantation [137].
B-cell depletion may have adverse effects [95]. Regulatory B cells inhibit effector T cells and facilitate the expansion of regulatory T cells, thereby potentially promoting tolerance. Therapies that target B cells may also deplete these important regulatory B cells. Further research is needed to identify the optimal agents and timing of administration that balance the effects of B cell depletion on rejection and graft survival.
Plasma Cell Depletion
Plasma cells produce large amounts of IgG, a proportion of which will be misfolded and require degradation by the proteasome. Bortezomib acts as a proteasome inhibitor and therefore results in accumulation of misfolded proteins and apoptosis of plasma cells. Developed for the treatment of multiple myeloma, bortezomib has been used in the treatment of AMR in transplant recipients, and may be particularly useful by preferentially targeting alloantibody-producing cells [138–142]. Toxicities with bortezomib have been reported, and randomized controlled trials are needed to formally evaluate this therapy prior to widespread adoption.
Antibody Removal
Immunoadsorption (IA), plasmapheresis (PP), and therapeutic plasma exchange (TPE) effectively and quickly remove circulating antibodies and have been used for desensitization and treatment of AMR [14, 56, 143–145]. A single exchange of one plasma volume with PP or TPE removes approximately two-thirds of all solutes in the plasma; therefore multiple sessions are required for adequate antibody removal. According to ISHLT guidelines, common protocols consist of 1–5 sessions of PP per week for 1–4 weeks [94]. The plasma volume is usually replaced with albumin or fresh frozen plasma. IA uses regenerable adsorbers that are able to process high volumes of plasma and remove antibody through affinity adsorption. It is more effective and specific at antibody removal, generally achieving >90 % antibody reduction with two sessions, but does not remove circulating cytokines. It is less likely to cause hemodynamic instability, however it is less widely available. Additional therapies targeting antibody activity or production should be combined with these methods of antibody removal to minimize the rapid rebound in antibody titers that occurs following treatment.
Antibody Inhibition
The activity of circulating antibodies can also be targeted, most commonly with intravenous immunoglobulin (IVIg). IVIg contains polyclonal IgG obtained from pooled plasma samples. There are multiple potential mechanisms by which IVIg may be effective in the treatment of AMR, including the expansion of regulatory T cells, up-regulation of the inhibitory FcyRIIB receptor on B cells, and the suppression of complement activation [146–148]. Although there is very little data from clinical trials examining the use of IVIg in transplant, it is commonly used for desensitization and for treatment of rejection, often in combination with PP and/or agents that target antibody production such as rituximab [149, 150]. Future use of IVIg may include IgG that has been engineered to have greater affinity for the MHC class I receptor FcRn, in which binding would compete with endogenous IgG and lead to a reduction in IgG titers [151].
Many of the changes seen in AMR are mediated through the complement cascade, and there is recent interest in use of agents that target this pathway. Small animal studies showed improved graft survival, decreased incidence of AMR, and reduced inflammatory infiltration following treatment with an anti-C5 monoclonal antibody or C5aR antagonist [152–154]. Eculizumab, an antibody directed against the complement component C5, has been successfully used in the treatment of AMR in renal transplant recipients [155–157]. Additional data is needed prior to routine use.
Other Measures
Glucocorticoids bind to glucocorticoid receptors on T cells, antigen presenting cells, and endothelial cells and modify gene regulation via the glucocorticoid response elements. Immunosuppressive effects include decreased production of multiple pro-inflammatory cytokines and interference with cytokine receptor signaling [159–161]. For AMR associated with hemodynamic abnormalities, high-dose corticosteroids (methylprednisolone 1000 mg IV daily for 3 consecutive days) should be used. In less severe cases, an oral bolus dose may be used. Table 22.3 summarizes the dose, frequency, duration, and most common side effects of these various drugs/interventions used for cardiac AMR.
Dose | Frequency | Duration | Side effects | |
---|---|---|---|---|
Plasmapheresis (PP) [94] | 1–2 plasma exchanges | Daily Every other day 3 times per week Once weekly | 3–5 days 1–2 weeks 1–4 weeks 2–4 weeks | Hypotension, bleeding, blood-borne infection |
IV immunoglobulin [94] | 100–1000 mg/kg | 1–3 times per week, often after PP | 1–4 weeks | Headache, fever, chills, thrombosis, volume overload, aseptic meningitis, acute renal failure |
Rituximab [94] | 375 mg/m2 | Once weekly | 1–4 weeks | Fever, hives, chills, leukopenia, infection, nausea |
1.3 mg/m2 | Every 3–5 days | 4 doses (2–4 weeks) | GI toxicity, thrombocytopenia, neutropenia, peripheral neuropathy | |
600–900 mg | Once weekly | 1–4 weeks | GI toxicity, nasopharyngitis, anemia, headache, leukopenia, | |
Corticosteroids Methylprednisolone Prednisone | 250–1000 mg 1–3 mg/kg | Daily Daily | 3 days 3–5 days | Fluid retention, hyperglycemia, osteoporosis, hypertension |
Antithymocyte Globulin (rabbit) | 0.75–1.5 mg/kg | Daily or every other day | 5–14 days | Fever, chills, hypotension, shortness of breath, thrombocytopenia |
In AMR, platelets and other components of the coagulation cascade are activated and microthrombi may develop in the small vessels of the allograft, potentially leading to ischemia and further graft dysfunction [32, 89]. When treating a patient for AMR, it is reasonable to administer systemic anticoagulation to decrease the risk of intravascular thrombosis [94].
Desensitization
Patients with pre-transplant sensitization have increased wait times to transplant, as well as increased rejection, higher rates of cardiac allograft vasculopathy, and decreased survival following transplant [3, 166–168]. Desensitization therapy may be performed in order to minimize these risks, and utilizes many of the same therapies used in the treatment of AMR [169]. Protocols vary by transplant center and may also be individualized for each patient. In 2009, the ISHLT published a report from a consensus conference on the management of sensitized patients which contains a description of desensitization protocols from six transplant centers [170]. Most programs treated patients with pre-transplant PRAs >50 % and used a combination of plasmapheresis, IVIg and rituximab. Bortezemib has also been successfully utilized, generally if initial treatments failed to adequately reduce PRA levels [171, 172]. Clinical trials are needed and are being organized around protocols for desensitization and prevention.
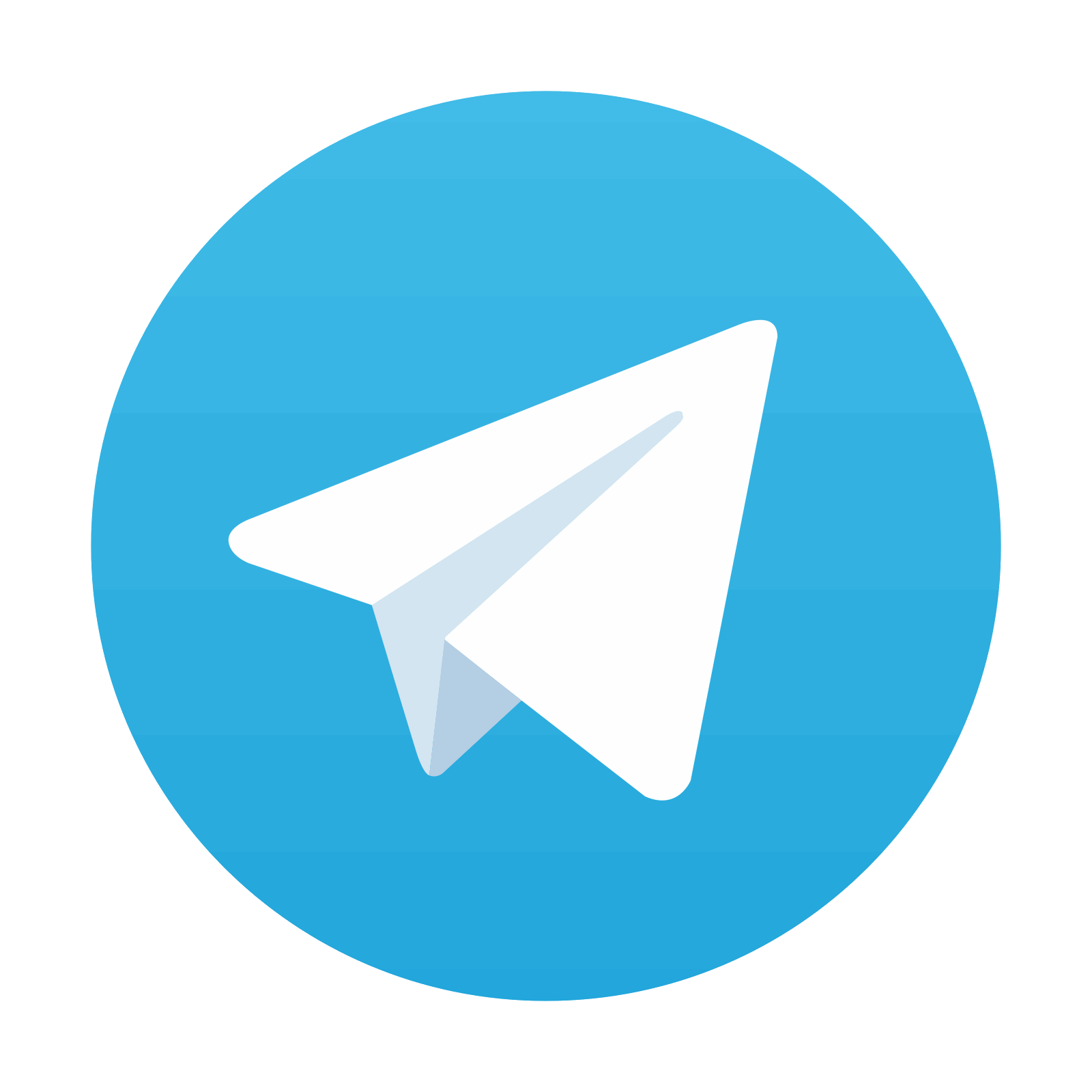
Stay updated, free articles. Join our Telegram channel

Full access? Get Clinical Tree
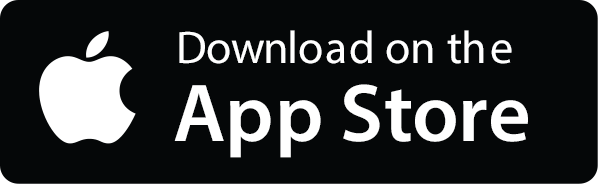
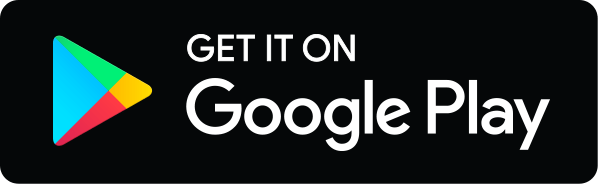