Animal Models in Pleural Investigation
The pleural space is involved in many different disease processes. Animal models of pleural disease offer the opportunity to answer many questions regarding the pathophysiology and treatment of pleural diseases. There are several advantages to studying animal models rather than human disease. Animals are certainly easier to recruit. Variables can be more closely controlled in animal models than in humans. This reduces variance in the results and allows conclusions to be made with a smaller number of study participants. Investigational agents can be instilled in animals with much less bureaucracy than when they are instilled in humans.
When performing experiments in animals, it is important to select the appropriate species. Investigators should be aware of two important anatomical characteristics of the species they use. First, many animals, such as mice, have incomplete mediastinal separations with the left and right pleural spaces being in free communication (1). This eliminates the possibility of using the contralateral pleural space as a control. Second, some animals such as sheep have a thick visceral pleura resembling that of humans, whereas other animals such as dogs, rabbits, and mice have a thin visceral pleura. This difference in the thickness of the pleura has large influences on the formation and reabsorption of pleural fluid. Another important factor to consider is the availability of commercial enzyme-linked immunosorbent assays (ELISAs) for the proposed species. These kits are usually available only for humans and mice and one must determine if there is sufficient cross-reactivity with other species such as rabbits, guinea pigs, and sheep (1).
A large number of animal models have been developed to study diseases of the pleura. In this chapter, an attempt is made to outline the more common models that have been developed and to give examples of the type of information that has been obtained with their use.
MALIGNANCY
In general, there are three types of experimental models of malignant pleural effusion. In the first type, cells derived from spontaneously occurring tumors are injected into the species from which they are derived in an attempt to produce a malignant pleural effusion. The animals are immunocompetent. In the second type, tumor cells from different species are injected intravenously or intrapleurally. Most animals in which malignancies have been induced have some type of immunologic deficiency. In the third type, malignant pleural mesothelioma develops after animals are exposed to asbestos particles (intrapleurally, inhalationally intratracheally, or intraperitoneally) (2).
Adenocarcinoma
In Mice. The best model malignant pleural effusion in mice has been developed by Stathopoulos et al. (3). In this model, 1.5 × 105 Lewis lung cancer cells, derived from a spontaneously arising lung adenocarcinoma in C57B/6 mice, are injected directly into the pleural space of C57B/6 mice. This results in multiple discrete pleural tumors and a malignant pleural effusion with a mean volume of 667 &µL by day 14 and a 100% mortality by day 17 (3). The unique aspect of this model is that the animals are immunocompetent.
This model has been used to study several aspects of malignant pleural effusion including the following.
(a) Nuclear factor (NF)-κB is important for the progression of the tumor. If Lewis lung cancer cells expressing a dominant NF-κB inhibitor are injected, there is decreased tumor burden and decreased pleural effusion volume (3). (b) Administration of a vascular endothelial growth factor (VEGF) inhibitor decreases the bulk of the tumor and the amount of pleural fluid (4). (c) The administration of recombinant adenovirus-mediated delivery of human endostatin resulted in significant reduction in pleural effusion volume, the number of pleural tumor foci, microvessel density, and vascular permeability, while it significantly prolonged the survival time (5). (d) Host-derived IL-5 promotes experimental MPE and may be involved in the pathogenesis of human MPE (6). (e) A sundilac derivative with antiangiogenic capabilities halted experimental pleural effusion formation and intrapleural tumor dissemination, through down-regulation of pleural vascular permeability (7). (f) Angiopoietin/Tie2 axis blockade significantly reduced pleural fluid volume and pleural tumor foci (8). (g) Zoledronic acid, an aminobiphosphonate, treatment resulted in significant reductions in pleural fluid accumulation and tumor dissemination, while it significantly prolonged survival (9). (h) The knockout of osteopontin in the lung cancer cells significantly reduces the formation of MPE, but does not inhibit in vivo tumor growth of the cancer cells in mice (10). (i) Elevated expressions of aquaporin-1 and VEGF-protein are associated with increased volume of malignant effusion (11). Studies such as those outlined above can provide insights into possible treatment for malignant effusions in humans.
(a) Nuclear factor (NF)-κB is important for the progression of the tumor. If Lewis lung cancer cells expressing a dominant NF-κB inhibitor are injected, there is decreased tumor burden and decreased pleural effusion volume (3). (b) Administration of a vascular endothelial growth factor (VEGF) inhibitor decreases the bulk of the tumor and the amount of pleural fluid (4). (c) The administration of recombinant adenovirus-mediated delivery of human endostatin resulted in significant reduction in pleural effusion volume, the number of pleural tumor foci, microvessel density, and vascular permeability, while it significantly prolonged the survival time (5). (d) Host-derived IL-5 promotes experimental MPE and may be involved in the pathogenesis of human MPE (6). (e) A sundilac derivative with antiangiogenic capabilities halted experimental pleural effusion formation and intrapleural tumor dissemination, through down-regulation of pleural vascular permeability (7). (f) Angiopoietin/Tie2 axis blockade significantly reduced pleural fluid volume and pleural tumor foci (8). (g) Zoledronic acid, an aminobiphosphonate, treatment resulted in significant reductions in pleural fluid accumulation and tumor dissemination, while it significantly prolonged survival (9). (h) The knockout of osteopontin in the lung cancer cells significantly reduces the formation of MPE, but does not inhibit in vivo tumor growth of the cancer cells in mice (10). (i) Elevated expressions of aquaporin-1 and VEGF-protein are associated with increased volume of malignant effusion (11). Studies such as those outlined above can provide insights into possible treatment for malignant effusions in humans.
In another model of malignant pleural effusion in mice, male thymic BALL/c nude mice (Animal Production Area of the National Cancer Institute, Frederick Cancer Research Facility, Frederick, Maryland) are used (12). The tumor cells (1 × 10s/300/<µL of HBSS lung adenocarcinoma cell line PC14PE6 or the squamous cell line H226) are injected into the lateral tail vein of unanesthetized nude mice. The mice develop colonies of the adenocarcinoma in the lungs as early as 4 weeks after tumor inoculation and all recipients of the adenocarcinoma line, but not the squamous cell line, develop bloody pleural effusions (13).
The role of VEGF in the production of malignant pleural effusion has been investigated using the model in the above paragraph (13). Yano et al. (14) found that the intravenous injection of PC14, the adenocarcinoma cell line, resulted in multiple lung lesions with invasion of the pleura, and produced a pleural effusion containing high levels of VEGF. The level of expression of VEGF messenger ribonucleic acid (mRNA) and protein by the cell lines directly correlated with the amount of pleural effusion. When the PC 14 cells were transfected with the antisense VEGF-165 gene, the tumor invasion of the pleura was not altered, but the amount of pleural effusion was decreased. When the H226 cells were transfected with either sense VEGF-165 or sense VEGF-121 genes, their direct implantation, but not their intravenous injection, led to localized vascular hyperpermeability and pleural effusion. This study suggested that in order for a metastatic malignancy to produce a pleural effusion, the tumor must both invade the pleura and express high levels of VEGF (14).
These same researchers then attempted to block the formation of malignant pleural effusion in their model by administering VEGF inhibitors (13). The inhibitor studied, PTK 787, is a specific inhibitor of VEGF receptor tyrosine kinase phosphorylation. The administration of PTK 787 at a dose of 100 mg/kg nearly eliminated the formation of pleural effusions, but doses of 10 and 50 mg/kg had no significant effect. PTK 787 appeared to decrease the formation of pleural fluid by reducing vascular permeability rather than by inhibiting tumor growth or pleural invasion. This experiment suggested that PTK 787 or other inhibitors of VEGF could be useful for the treatment of malignant pleural effusions.
Nagamachi et al. (15) assessed the effects of injecting various human non-small cell lung carcinoma (NSCLC) cell lines into nude mice. They injected 2 × 106 cells from eight different cell lines into the left pleural space. Seven of the eight cell lines were tumorigenic and growth rates were relatively high in four of the cell lines (PC14, Lu65, Lu99A, and H157) (15). Pleural effusions were not mentioned with these cell types. However, when mice were injected with the PC9 cells, they developed malignant pleural effusions (15). It was concluded that the PC9 cells could be used for a model of malignant pleural effusion (15).
In Rats. Ohta et al. (16) have developed a model of malignant pleural effusion in immune deficient rats that also uses the PC-14 adenocarcinoma cell line. These researchers reported that the injection of 1 × 107 cells into the subpleural space of the parietal pleura or into the pneumonectomy space resulted in disseminated malignancy in 8/8 animals and the development of a pleural effusion in 5/8 animals with a positive pleural fluid cytology in two (16). Interestingly, these workers showed that the intraperitoneal injection of monoclonal antihuman VEGF antibody
at a dose of 250 µ twice weekly prevented the development of the pleural effusion but did not affect the dissemination of the tumor (16).
at a dose of 250 µ twice weekly prevented the development of the pleural effusion but did not affect the dissemination of the tumor (16).
Ebright et al. (17) injected 1 × 107 A549 cells of the human lung adenocarcinoma into nude male rats and reported that by 5 weeks, three of nine rats had developed pleural effusions. All the rats had tumor nodules on the lung and pericardium (17). In this model the intrapleural administration of NV1020, which is a novel, multimutated, replication-restricted herpes simplex virus, significantly inhibited the growth of the tumor (17).
Prosst et al. (18) used a similar model to assess the usefulness of thoracoscopic fluorescence in the diagnosis of pleural malignancies. These workers injected human lung cancer cells (adenocarcinoma 82/5) into the right pleural cavity through the lateral diaphragm and found that after 5 to 7 weeks the entire pleural cavity was affected with the malignancy (18). They did not mention whether the animals developed pleural effusions. They used this model to demonstrate that fluorescence enhanced the diagnostic capabilities of thoracoscopy (18).
In Rabbits. The intravenous injection of VX2 tumor cells in rabbits can lead to the development of a pleural effusion (19,20). The VX2 is rabbit papilloma tumor. Tumors are created in rabbits by the intravenous injection of approximately 25,000 VX2 cells obtained from an alive donor rabbit (19,20). When the rabbits are sacrificed 28 days after the injection, approximately 15% have a pleural effusion (20). The problem with this model is that the production of each pleural effusion is expensive because pleural effusions develop in only 15% of the animals. We have tried to create malignant effusions in rabbits by injecting the VX2 cells directly into the pleural space but were unsuccessful.
Melanoma
Melanoma metastatic to the lung can be produced by injecting murine melanoma cell lines into the lateral tail vein of syngeneic mice (21). In this model, syngeneic mice receive 5 × 104 B16-BL6 or B16-F10 melanoma cells in a total volume of 0.2 mL. At sacri-fice 21 days after the cells were injected, Wang et al. (21) reported that all 20 mice injected with the two different cell lines developed lung metastases. The injection of B16-BL6 cells resulted in a pleural effusion in 8/10 with a median volume of 250 µ whereas the injection of the B16-F10 cells resulted in a pleural effusion in 9/10 mice with a mean volume of 320 /<µL. If the melanoma cells were administered to syngeneic mice lacking the gene for nitric oxide synthase (NOS) II, only approximately 50% of the animals developed pleural effusions and those that did had a lower volume (21). Immunohistochemical analyses indicated that absence of NOS II expression was correlated with decreased VEGF expression and tumor-associated vascular formation.
Fibrosarcoma
The intrapleural injection of fibrosarcoma can lead to a pleural fibrosarcoma with pleural effusion. Yasutake et al. (22) injected 1 × 105 Meth A fibrosarcoma cells intrapleurally in syngeneic BALB/c mice. Al the injected mice in this model developed pleural fibrosarcoma and all developed pleural effusions with a mean volume of 733 /<µL. The mean survival of the injected mice was only 8.7 days after injection. Interestingly, when heat-killed cells of Lactobacillus casei were injected on day 3 or day 6, the mean survival was increased beyond 40 days and the amount of pleural fluid was only 14 /<µL (22). Intrapleural injection of antibodies against tumor necrosis factor alpha (TNF-α) completely eliminated the antitumor effect of the heat-killed cells of L. casei (22). The results of this study suggest that TNF-α can act as an antitumor drug in this model.
ASBESTOS AND MESOTHELIOMA
There have been many papers published on the relationship between asbestos and mesothelioma. Wagner (23) first reported in 1962 that in rats the intrapleural administration of chrysotile and crocidolite led to the production of mesothelioma. Then in 1965, Smith et al. (24) demonstrated that the intrapleural injection of amosite in hamsters led to the development of mesothelioma. Subsequently, there have been many papers written on the induction of mesotheliomas in animals. Asbestos has been administered intrapleurally, intratracheally, intraperitoneally, and by inhalation. In the following section, the results will be briefly described when asbestos is administered through these different routes.
Intrapleural Injections
The intrapleural injection of asbestos leads to the development of mesothelioma of the pleural space. Most studies have been performed in rats (23, 25,26,27,28,29,30), although there has been at least one report in hamsters
(24). When asbestos fibers are injected intrapleurally, approximately 30% to 60% of the animals develop a pleural mesothelioma (26,30). The tumors develop approximately 18 months after the intrapleural injection (26). There is a dose response, with larger doses being associated with higher incidence of tumor (30). When different types of asbestos are compared, the intrapleural injection of 25 mg chrysotile or crocidolite produce mesotheliomas in approximately 60% whereas the same dose of amosite produces tumors in 35% (31). The main difference in these types of asbestos is that mesotheliomas occur approximately 200 days later for amosite than for the other two types (31).
(24). When asbestos fibers are injected intrapleurally, approximately 30% to 60% of the animals develop a pleural mesothelioma (26,30). The tumors develop approximately 18 months after the intrapleural injection (26). There is a dose response, with larger doses being associated with higher incidence of tumor (30). When different types of asbestos are compared, the intrapleural injection of 25 mg chrysotile or crocidolite produce mesotheliomas in approximately 60% whereas the same dose of amosite produces tumors in 35% (31). The main difference in these types of asbestos is that mesotheliomas occur approximately 200 days later for amosite than for the other two types (31).
The propensity of a fiber type to produce a mesothelioma is dependent to a large extent on its geometric configuration. Long, thin fibers appear particularly likely to produce mesothelioma in man (27). The chemical composition of the asbestos fiber also influences whether its intrapleural injection will induce mesothelioma. If more than 80% of the magnesium is leached from chrysotile fibers, the proportion of animals developing mesotheliomas is dramatically lower than with untreated chrysotile (28). The rate of pleural mesothelioma will be more than doubled if pleural inflammation is induced by the intrapleural injection of carrageenan several months after the initial injection of asbestos (31).
Of all fibers tested, the most potent inducer of pleural mesothelioma is erionite (25,32). Wagner et al. (32) injected 20 mg of Oregon erionite, Karain Rock fiber, chrysotile, or nonfibrous zeolite intrapleurally to rats, and the incidence of mesothelioma was as follows: erionite 40/40 (100%), Karain Rock fiber 38/40 (95%), chrysotile 19/40 (48%), and nonfibrous zeolite 2/40 (5%) (32). Erionite in the air has been implicated in the epidemic of mesotheliomas in Turkey (33).
If normal rat mesothelial cells are treated in vitro with chrysotile fibers, mesotheliomas will develop rapidly when these cells are injected subcutaneously into nude mice. Fleury-Feith et al. (34) demonstrated that when untreated cells were injected, tumors developed in 3/5 animals at a median of 22 weeks after injection. However, if the cells were treated repeatedly with chrysotile, tumors developed in 5/5 animals at a median of only 1 week after injection (34).
Inhaled Asbestos
It is more difficult in general to produce tumors by the inhalation of asbestos than by intrapleural administration. There have been numerous reports of the effects of inhaled asbestos on rats (35,36,37,38,39,40,41), and one on baboons (42). Wagner et al. (40) exposed rats to asbestos clouds 7 hours/day, 5 days/week for 1 year. Even when asbestos is administered with such intensive regimens, the incidence of mesothelioma is less than it is after one intrapleural (41) or one intraperitoneal injection (38) of asbestos. When rats are given inhaled asbestos for 1 year, less than 10% develop mesothelioma, but approximately 30% develop lung tumors (40). Multivariate fibers analysis of multiple inhalational experiments has shown that the measure most highly correlated with tumor incidence is the concentration of structures 20 µ or more in length (35). Potency appears to increase with increasing length, with fibers longer than 40 µ being approximately 500 times more potent than fibers between 5 and 40 /<m (35). When different types of asbestos are compared, chrysotile is less potent than amphiboles in inducing mesothelioma, but comparable in producing lung tumors (35). The most effi-cient agent at inducing mesothelioma after inhalation is erionite (32). Wagner et al. (32) demonstrated that the inhalation of Oregon erionite induced mesotheliomas in 27 of 28 (96%) rats and decreased their mean survival time from 738 days to 504 days.
It has also been demonstrated that inhaled asbestos can induce mesotheliomas in primates. Webster et al. (42) exposed baboons 6 hours daily for 5 days a week to amosite asbestos, except for 3 weeks of the year when the chamber had to be serviced. The animals were exposed for up to 900 days. Five of 10 animals developed malignant mesothelioma between 6 and 10 years after the initial exposure, and the remaining animals all developed asbestosis (42).
Intratracheal Injections
There have also been a few reports on the effects of asbestos administered intratracheally in hamsters (43,44), dogs (45), and monkeys (45). It is less burdensome to administer the asbestos intratracheally than to do inhalational treatments nearly daily for a year or more. However, in general it is difficult to induce mesotheliomas with intratracheal asbestos. Pylev (43) administered 10 mg of chrysotile twice a month to hamsters and reported that only 1 of 41 animals developed a malignant mesothelioma, although 25% of the animals developed a malignant lung tumor. The same researcher also tried to induce tumors in three monkeys with the intratracheal administration of 400 to 600 mg asbestos and reported that no tumors developed during 17 to 22 months of follow-up (43).
However, mesotheliomas were easier to induce in rats as the intratracheal injection of commercial chrysotile and commercial anthophyllite induced mesothelioma in 65.5% and 41.4%, respectively. Adachi et al. (44) administered amosite and crocidolite asbestos (2 mg/ dose) intratracheally once a week for 5 weeks to Syrian hamsters and observed them for the following 2 years. Only 1 of the 40 animals that were exposed developed a mesothelioma. The study with the highest incidence of mesothelioma after intratracheal asbestos was reported by Humphrey et al. (45). In this study crocidolite, 4.75 mg/kg/week, was administered for 3 weeks every year for 4 years to 10 dogs. Seven of the dogs also smoked nine cigarettes per day, 5 days/week. They reported that pleural mesotheliomas developed in 6 of the 10 animals (60%), including 2 of the 3 that did not smoke (45). The animals with the mesotheliomas died between 6.5 and 9 years after the initial exposure. Four of the animals with mesothelioma had concomitant adenocarcinomas.
Intraperitoneal Injections
In general, it takes a smaller amount of asbestos administered intraperitoneally compared with that administered intrapleurally to produce a mesothelioma in the cavity where the asbestos was injected (25). The tumors after intraperitoneal injections usually occur in the peritoneal cavity. There have been many studies on the induction of mesothelioma by the intraperitoneal injection of asbestos, primarily because it is the easiest way to administer asbestos. The intraperitoneal injection of asbestos produces tumors sooner (˜9 to 10 months) compared with intrapleural injections (˜18 months) (26). Moreover, the order in which fibers induce mesotheliomas is the same after intrapleural and intraperitoneal injection. Erionite is the most potent, followed by chrysotile, crocidolite, and then amosite (25). Interestingly, silicon carbide is more potent at inducing peritoneal mesotheliomas than are any of the asbestos types (46).
The length of the fiber appears to be important in producing mesothelioma after intraperitoneal injections as well as intrapleural injections (47). Fibers longer than 20 µ and thinner than 0.95 µ were most likely to induce peritoneal mesotheliomas. When animals develop malignant peritoneal mesotheliomas after the intraperitoneal injection of asbestos, the first manifestation is the development of ascites (48). The ascitic fluid can then be injected into other animals so that the antitumor effects of different chemotherapeutic agents can be assessed (49).
ASBESTOS PLEURAL EFFUSIONS
Clinically, pleural effusions develop in patients who have been exposed to asbestos. The time between the initial exposure and the appearance of the pleural effusion is usually several decades. Attempts have been made to duplicate this asbestos pleural effusion in animals. When animals are observed for prolonged periods after asbestos is administered intrapleurally or inhaled, no benign effusions are observed. The intrapleural administration of 5 mL of 1% crocidolite asbestos suspension (50) into the pleural spaces of rabbits does result in a pleural effusion of 1.5 mL at 4 hours. The pleural fluid white blood cell (WBC) count and the chemotactic activity were higher in the animals given asbestos than in animals given saline (50). It was subsequently shown that interleukin 8 (IL-8) was responsible for much of the chemotactic activity (51). The relationship of this model to the asbestos effusion in man is not clear because the time course is so different.
Sahn and Antony (52) demonstrated that the intrapleural injection of 150 mg of UICC chrysotile B asbestos into rabbits resulted in a pleural effusion that reached its maximal volume of 3.8 ± 0.5 mL at 7 days and gradually decreased after that time. At 3, 6, and 8 months there was generally less than 1.5 mL pleural fluid present (52). The peak cellular influx (WBCs = 27,000 cells/mm3) occurred at 24 hours when there were predominantly neutrophils in the pleural fluid. By 72 hours, macrophages were the predominant cells, whereas at 120 hours, lymphocytes were the predominant cells. The animals developed pleural plaques by 7 days and the pleural plaques were fully developed by 28 days. If the animals were made neutropenic before the asbestos was injected, there was never a macrophage influx. Moreover, the neutropenic animals did not develop pleural plaques, but rather developed marked fibrosis of the pleural space (52).
EMPYEMA
In general, there are only a limited number of good human studies on the treatment of parapneumonic effusion and empyema (53). One reason for this is that there is tremendous diversity in patients who have parapneumonic effusions and empyema. To compensate for this diversity, patients should be stratified according to the severity of their illness and their comorbidities (54). However, once strati-fication is accomplished, a given medical center sees only a few patients in each category per year. Therefore, good studies take a long time or they need to be
multicentered. Unfortunately, multicentered studies are expensive and difficult.
multicentered. Unfortunately, multicentered studies are expensive and difficult.
In view of these factors, one would think that there would be many studies in animals to help answer questions such as the following: (a) What is the role of therapeutic thoracentesis in the management of parapneumonic effusions? (b) What is the role of fibrinolytics in the management of loculated parapneumonic effusions? (c) Is the penetration of all antibiotics into the pleural space similar? (d) Is there a role for intrapleural antibiotics in the management of pleural infections?
Surprisingly, there has been little work done with experimental empyema. This may be related to the fact that it is difficult to produce an empyema in an animal without killing it. For example, if Staphylococcus aureus, Escherichia coli, or Bacteroides fragilis is injected into the pleural space of guinea pigs, the animals either survive without developing empyema or die of overwhelming sepsis (55).
At least six different models of experimental empyema have been developed.
Direct Injection into Dogs. This model was the first model developed to help study the empyema epidemic that occurred in United States servicemen during World War I. Graham and Bell (56) in 1918 injected 30 mL of pure broth cultures of a virulent strain of hemolytic streptococci into the pleural cavity of dogs. The first dog injected with this amount of broth died within 12 hours, but had 200 mL of serofibrinous fluid with a myriad of streptococci and a few necrotic leukocytes. This exudate was quite similar to that which was recovered from early human cases of streptococcus empyema. When these investigators subsequently injected smaller amounts of the broth into the animals, the survival improved. In their primary experiment, 20 dogs were injected with 1 to 3 mL of the pure broth. Then 4 to 24 hours after the injection, open drainage of the pleural space was established with a chest tube in 10 of the dogs, whereas the remaining dogs did not receive a chest tube. The dogs were paired and the stronger dog of the pair was selected for the open drainage. Nine of the 10 dogs that received the open drainage died, whereas only 7 of the 10 dogs without the drainage died. The only dog with the open drainage that survived pulled its chest tube out the day following the operation. In each pair, the dog that received the chest tube died sooner than the control dog (56). This experiment demonstrated that open drainage was detrimental to the dog. The results from this experiment were extrapolated to man and the practice of early open tube drainage of empyemas was discontinued.
Rabbits with Turpentine-Induced Pleural Effusions. This second model was developed by Sahn and Potts (57) in the late 1970s. In this model a sterile exudative pleural effusion was induced in rabbits by intrapleural injection of 0.3 mL turpentine. An empyema was produced when 1 × 109 Streptococcus pneumoniae organisms were injected into the pleural fluid 96 hours after the turpentine was injected (58). Within 3 hours of injecting the bacteria, the pleural fluid pH fell to a mean of 7.05 and the pleural fluid glucose fell to below 46 mg/dL. By 6 hours postinjection, the mean pleural fluid WBCs exceeded 75,000/ mm3. Subsequently, Shohet et al. (59) used this model to study empyemas due to Klebsiella pneumoniae. They injected 1 × 109 K. pneumoniae 96 hours after the turpentine was injected and demonstrated that at 1, 3, and 5 days postinoculation, the pleural fluid glucose was below 10 mg/dL and the pleural fluid pH was below 7.00 (59). It has also been demonstrated with this model that clarithromycin, moxifloxacin, and levofloxacin diffuse rapidly into the pleural fluid after they are administered intravenously. The area under the curve for all three of these antibiotics in the pleural fluid is higher than their area under the curve in simultaneously obtained serum (60,61).
One must question how closely this model mirrors empyema in humans because without antibiotic therapy or tube thoracostomy the pleural effusions resolve within 10 days, and at autopsy there are no significant findings in the pleural cavity or lungs (58). In addition, although this model has been used to study the penetration of antibiotics into the pleural space (59,60,61), the pleural thickening induced by the turpentine probably influenced the results.
Guinea Pigs with Umbilical Tape. The third model was developed in the mid-1980s to assess the factors that influence the development of an empyema when bacteria are injected into the pleural space (55). In this model, bacteria are injected into the pleural space after umbilical tape is placed in the pleural space to act as a foreign body to promote infection. If umbilical tape is not placed, the animals die of sepsis and do not develop an empyema (55). After the guinea pigs are anesthetized with an intramuscular injection of ketamine hydrochloride, 55 mg/kg, and acepromazine maleate, 0.5 mg/kg, a small skin incision is made at the level of the sixth to ninth intercostal space and the pleural space is bluntly entered. Then a piece of cotton umbilical tape 1 cm long and 1/8 in. wide is placed in the pleural space.
Using this model, Mavroudis et al. (55) demonstrated that the development of an empyema is
dependent upon the organisms injected. None of the animals injected with B. fragilis developed empyema, whereas empyema developed in 33% of those injected with B. fragilis plus S. aureus and 31% of those injected with E. coli plus B. fragilis (62). These investigators also used this model to demonstrate that the more organisms injected into the pleural space, the more likely the animal was to develop an empyema. For example, if 1 × 108 E. coli plus B. fragilis were injected, 10/10 animals developed an empyema, whereas if 1 × 106 of these organisms were injected, only 5/10 animals developed an empyema (55). If blood were injected in conjunction with the bacteria, the likelihood of developing an empyema was not increased. Lastly, guinea pigs that developed pneumonia were more likely to have an empyema.
dependent upon the organisms injected. None of the animals injected with B. fragilis developed empyema, whereas empyema developed in 33% of those injected with B. fragilis plus S. aureus and 31% of those injected with E. coli plus B. fragilis (62). These investigators also used this model to demonstrate that the more organisms injected into the pleural space, the more likely the animal was to develop an empyema. For example, if 1 × 108 E. coli plus B. fragilis were injected, 10/10 animals developed an empyema, whereas if 1 × 106 of these organisms were injected, only 5/10 animals developed an empyema (55). If blood were injected in conjunction with the bacteria, the likelihood of developing an empyema was not increased. Lastly, guinea pigs that developed pneumonia were more likely to have an empyema.
The primary problem with this model is that it requires a foreign body in the pleural space that does not mimic the clinical situation in humans.
Rabbits with Bacteria in Agar. The fourth model was developed in the late 1990s by Sasse et al. (63). In this rabbit model, 1 × 109 Pasteurella multocida cultured in agar (rather than broth) were injected into the pleural space of rabbits. Agar, rather than broth, was used so that the mixture would remain in the pleural space longer. Procaine penicillin G was administered once per day starting 24 hours after the initial injection to prevent death from sepsis. With this model, the rabbits developed an empyema; 24 hours postinjection, the mean pleural fluid pH was 7.01, the mean glucose was 10 mg/dL, the mean lactic acid dehydrogenase (LDH) was 70 times higher than the upper normal limit for serum, and the Gram’s stain and culture of the pleural fluid were positive (63). At 96 hours postinjection, the Gram’s stain and culture of the pleural fluid were usually negative, but gross pus remained in the pleural space. Ten days postinjection, at least 50% of the animals had gross pus in their pleural space. Approximately 60% of the rabbits survived for 14 days and at autopsy most animals had pus in their pleural space. The typical rabbit at autopsy at 14 days would have its entire right chest filled with thick pus. In addition, the right lung would be completely collapsed with a thick peel of collagenous material covering the visceral pleura. Adhesions between the visceral and parietal pleura were uncommon.
This model closely mimics the empyema that occurs in humans. The process is not cured with antibiotics alone. The pleural fluid becomes infected, then loculated, and a rind is formed over the visceral pleura. The loculation in this model is less than that seen in human empyema.
This model has been used in an attempt to answer several questions concerning the management of empyema. One question that was investigated was whether therapeutic thoracentesis was a reasonable alternative to tube thoracostomy in the management of rabbits with empyema (64). Rabbits were randomized to receive daily therapeutic thoracentesis starting at 48 hours, chest tubes at 48 hours, or neither therapeutic thoracentesis nor tube thoracostomy (controls). The animals in the chest tube group had their chest tubes attached to a Heimlich valve and had their chest tubes aspirated at 12-hour intervals. In this study, the mortality rate in the therapeutic thoracentesis group (0/16) was significantly less (p = 0.02) than the mortality rate in the other two groups combined (9/33). When the surviving animals were sacrificed at 10 days, the gross empyema score in the therapeutic thoracentesis group was significantly lower (p < 0.05) than that in the chest tube group or the control group (64). This study suggests that therapeutic thoracentesis possibly has a role in the management of patients with empyema.
A second question addressed was whether the timing of the chest tube insertion is important in the treatment of empyema. Rabbits were randomized to receive no chest tube or a chest tube 24, 48, or 72 hours after receiving the P. multocida intrapleurally (Fig. 4.1) (65). The rabbits that received the chest tube at 24 or 48 hours had significantly better results
than did the rabbits that received the chest tube at 72 hours or those that did not receive a chest tube at all (65). This study demonstrates that a relatively short delay in initiating tube thoracostomy adversely affects the outcome in these animals with an empyema.
than did the rabbits that received the chest tube at 72 hours or those that did not receive a chest tube at all (65). This study demonstrates that a relatively short delay in initiating tube thoracostomy adversely affects the outcome in these animals with an empyema.
The third question addressed with this model was whether all antibiotics penetrate empyemic pleural fluid similarly (66). Twenty-four hours after the intrapleural injection of P. multocida, Teixeira et al. (66) injected either penicillin 24,000 units/kg, clindamycin 9 mg/kg, gentamicin 1 mg/kg, metronidazole 37 mg/kg, vancomycin 15 mg/kg, or ceftriaxone 30 mg/kg intravenously. Antibiotic levels in samples of pleural fluid and serum, collected serially for up to 480 minutes, were determined using a bioassay. In this study, the degree to which antibiotics penetrated the pleural fluid was highly variable (Figure 12.2) (66). Metronidazole penetrated most easily, followed by penicillin, clindamycin, vancomycin, ceftriaxone, and gentamicin. This variance in the penetration of antibiotics into empyemic pleural fluid should be taken into consideration when antibiotic therapy is chosen in patients with empyema.
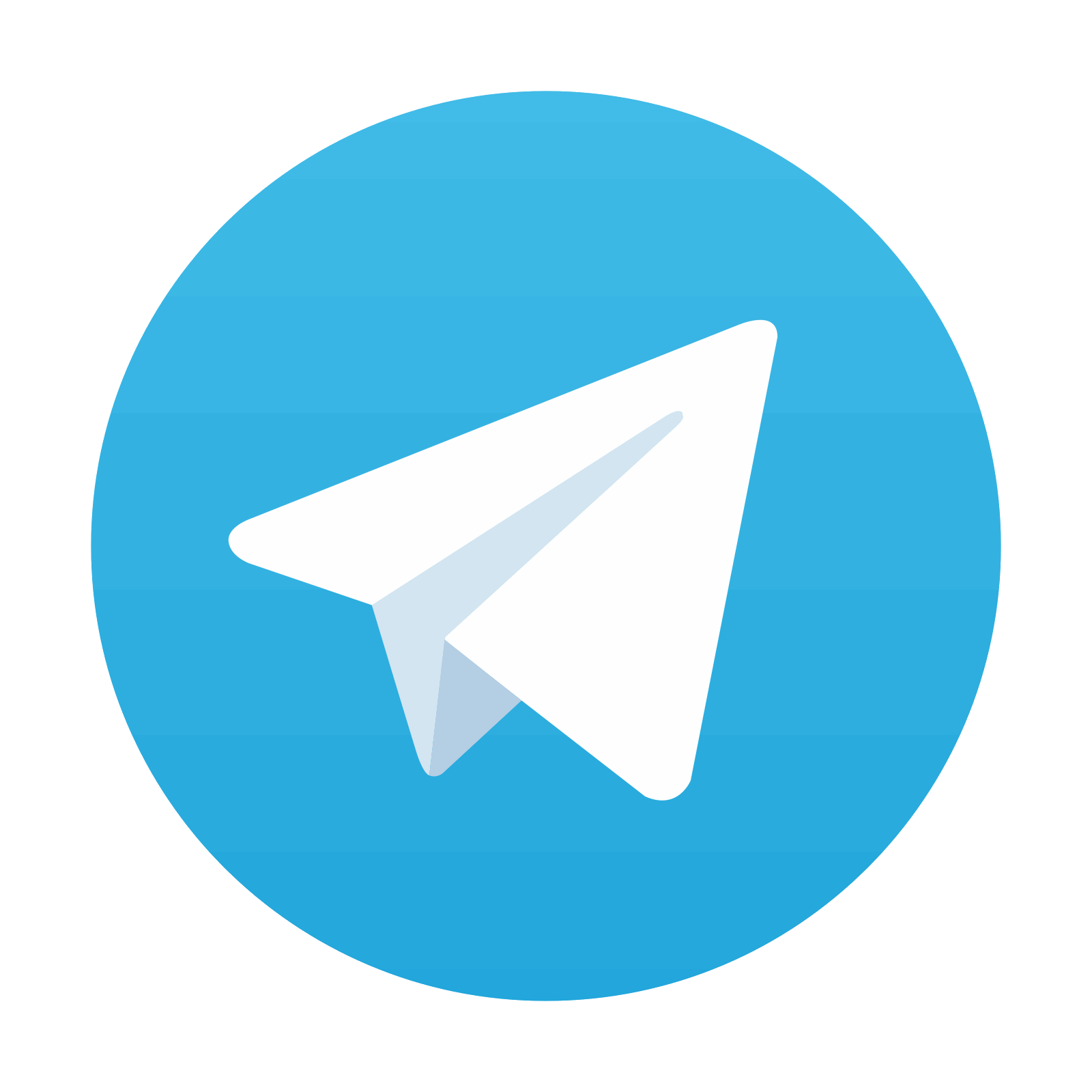
Stay updated, free articles. Join our Telegram channel

Full access? Get Clinical Tree
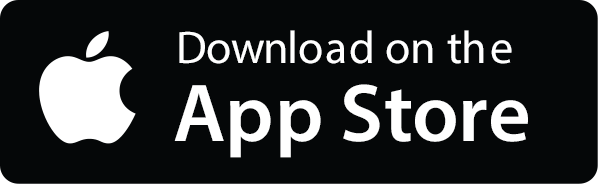
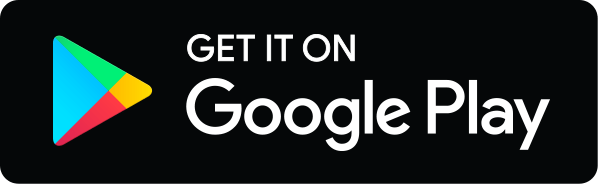