Anesthetic management of the thoracic surgical patient may improve operative conditions, efficiency, and outcome. Overlap exists in the territories of responsibility between thoracic surgeons and anesthesiologists, highlighting the importance of communication and mutual understanding. This chapter provides a brief overview of the general conduct of anesthesia for pulmonary resections, followed by a discussion of selected concepts in thoracic anesthesia of utility to thoracic surgeons.
Barring extremes of pathophysiology (e.g., end-stage chronic obstructive pulmonary disease), lesion-related hazards (e.g., compression of vital structures), or important coexisting disease states, the conduct of anesthesia for pulmonary resection is largely dictated by the surgical approach. For thoracotomy, the most common strategy consists of general anesthesia with paralysis, an arterial catheter (and possibly a central venous catheter), double-lumen tube (DLT), thoracic epidural, and immediate postoperative extubation.
Before induction, antibiotics, sedatives, and nebulized bronchodilator treatments are administered as indicated and the epidural is positioned and tested. After induction, diagnostic bronchoscopy may be performed via a large (8–8.5 mm) endotracheal tube or laryngeal mask airway (LMA). Findings that may affect the lung isolation plan should be noted by the anesthesia team at this time. Lung isolation by DLT or bronchial blocker (BB) then is imposed, with confirmation of position by pediatric fiberoptic bronchoscopy. After lateral decubitus positioning, repeat bronchoscopy is recommended. Ventilator parameters must be adjusted with initiation of one-lung ventilation (OLV) to ensure adequate gas exchange and to prevent barotrauma. Surgical entrance of the pleural space permits direct evaluation of the quality of lung isolation. Suctioning of secretions may aid atelectasis. Blood products should be available and checked before hilar dissection. Cross-clamp of the pulmonary artery typically does not cause changes in central venous pressure (CVP) or hemodynamics in patients with adequate cardiopulmonary reserve, and oxygenation should improve (see below). On cross-clamping of the bronchus, unchanged ventilatory compliance should be confirmed. If available, bronchoscopic visualization of the stump is useful before stapling. A “leak test” is commonly used by providing 20 to 30 cm H2O of positive pressure ventilation to the submerged stump. Recruitment of any remaining lung is accomplished with incremental 5-second periods of 20 to 40 cm H2O of positive pressure (recruitment maneuvers). After closure and supine repositioning, a final bronchoscopy via a large tube or LMA may necessitate a tube exchange. Rapid emergence and extubation depend on the strategic use of short-acting anesthetic agents; limited narcotic use; full reversal of muscle relaxation; control of secretions, bronchospasm, and pain (thoracic epidural); and return of airway reflexes, sensorium, and adequate respiratory efforts. Respiratory mechanics are greatly aided by raising the head of the bed more than 30 degrees at emergence (see Chapter 8).
Variations on the foregoing and procedure- or lesion-specific issues are presented as “bullet points” at the conclusion of this chapter.
The anesthesiologist should equally invest in assessments of cardiopulmonary reserve and coexisting disease states, as discussed in Chapter 4. Beyond that, the broad goal of the preoperative anesthetic evaluation is to identify issues with an aim toward reducing perioperative risk through preemption or preparation. The history, physical examination, and review of radiographic information should be targeted to anticipate problems with induction, airway management, lung isolation, vascular access, and pain management. Acute processes (e.g., respiratory infections) or the need to optimize treatment of existing conditions occasionally may justify postponement, but the semielective nature of thoracic oncologic surgery often mandates a higher risk tolerance.
Induction risk is increased in patients with threatened major airways, tamponade physiology, difficult airway anatomy, and traumatic or emergency scenarios. Paraneoplastic or associated syndromes (e.g., carcinoid syndrome, myasthenia gravis, Eaton–Lambert syndrome) have specific management implications (see below). Factors predictive of desaturation during OLV may be identified in advance (Table 5-1).
Patient medication regimens, in general, should not be disrupted, with the exception of insulin, oral hypoglycemic agents, and anticoagulants. Guidelines for how long anticoagulants should be held before placing an epidural have been published in a consensus statement by the American Society of Regional Anesthesia1 (Table 5-2). Patients with ischemic cardiac disease may benefit from thoracic epidural analgesia (TEA) and invasive hemodynamic monitoring despite a minimally invasive surgical approach. In such patients, perioperative beta-adrenergic blockade should not be withheld for fear of bronchospasm. Avoiding inhalational anesthetics and narcotics by use of total intravenous anesthetic (TIVA) techniques, together with TEA, may enable early extubation despite severe obstructive lung disease.
ANTICOAGULANT | DURATION |
Aspirin/nonsteroidal anti-inflammatory drug/COX-2 | 0 |
Unfractionated heparin (5000 units subcutaneous) | 0 |
Unfractionated heparin (intravenous, therapeutic) | 4 h |
Low-molecular-weight heparin | |
High dose | 24 h |
Low dose | 12 h |
Coumadin | 3 d |
Selective ADP inhibitors | |
Clopidogrel (Plavix) | 7 d |
Ticlopidine (Ticlid) | 14 d |
Glycoprotein IIb/IIIa inhibitors | |
Tirofiban (Aggrastat) | 2 h |
Eptifibatide (Integrilin) | 4 h |
Abciximab (Reopro) | 36 h |
Invasive arterial blood pressure monitoring is indicated for most thoracotomies and many lesser procedures in which there is unstable or severe cardiac disease or the opportunity for catastrophic bleeding. Central venous blood pressure monitoring is significantly distorted by alterations in intrathoracic pressure during thoracic surgery, but such catheters are useful when venous access is problematic or for postoperative volume management. Central lines are best placed on the operative side because a pneumothorax in the nonoperative chest would be problematic during OLV.
Pulse oximetry is a standard, invaluable monitor but possesses limitations that deserve to be understood. Pulse oximetry does not measure oxygen saturation directly. Red (660 nm) and near-infrared (940 nm) light is emitted from diodes and attenuated by the tissue and blood through which it passes. The pulsatile portion of the signal is presumed to be arterial blood. The Spo2 value is derived from the ratio of pulse-added absorbances at 940 nm (oxygenated hemoglobin) and 660 nm (reduced hemoglobin). Motion, ambient light, and venous pulsations can introduce artifact. Other species of hemoglobin will introduce error. Carboxyhemoglobin falsely elevates SpO2. High methemoglobin levels lead to an Spo2 of 85% regardless of Pao2. Spo2 readings below 85% should not be considered precise.
Monitoring for myocardial ischemia is compromised during left-sided thoracic surgery by the inability to place electrocardiogram leads in physiologic positions. Even in right-sided surgery, the position of the heart relative to the chest is altered, reducing electrocardiographic sensitivity. Transesophageal echocardiography may prove useful as a monitor of ischemia as well as right-sided heart function in response to pulmonary artery cross-clamping but does not necessarily predict subsequent right-sided heart failure. Transesophageal echocardiography also may help to guide surgical decisions in the evacuation of loculated pericardial effusions or the assessment of pulmonary venous anastomoses after lung transplantation. Pulmonary artery catheters are used infrequently in thoracic surgery because of the potential pitfalls in interpretation,2 the risk of entrapment in resection staple lines, and the limited need for left-sided filling pressures or cardiac output determinations. Right-sided heart pressures (CVP) are generally of greater value.
Capnography has become a standard monitor and reliably confirms alveolar ventilation and cardiac output. The capnogram shape roughly correlates with the degree of obstructive disease (Fig. 5-1). The gradient between end-tidal CO2 and Paco2 is affected by the amount of dead space, which can be significant and variable in thoracic surgical patients. Acute changes in capnogram waveforms may signal bronchospasm, tube malposition, circuit disconnection, ventilator valve malfunction, or exhausted CO2 absorbant. Acute pulmonary embolism results in a sudden decrease in end-tidal CO2 (increased dead space). High end-tidal CO2 levels also can be the earliest sign of malignant hyperthermia. Effective chest compressions during cardiac arrest are reflected by the return of a capnogram.
In the lateral decubitus position, the head and neck should be supported in line with the spine, with attention to protecting the eyes and dependent ear. Lateral neck flexion may cause traction injury of the suprascapular nerve and postoperative shoulder pain. An axillary roll placed caudad to the axilla relieves pressure on the dependent humeral head and axillary nerves and vessels. Excessive abduction of the nondependent arm (>90 degrees to the torso) may cause brachial plexus injury or contribute to shoulder pain. Ulnar nerves at the elbow and the dependent peroneal nerve are vulnerable and should be padded.
Induction implies loss of consciousness. Inhalation of volatile anesthetics (e.g., sevoflurane or desflurane) can achieve induction with maintenance of spontaneous ventilation. Intravenous inductions (e.g., propofol, thiopental, or etomidate) usually produce apnea. Propofol is used most commonly for its favorable antiemetic and kinetic profile. As a continuous infusion, propofol (with or without narcotic) is a TIVA or a useful sedative in lower doses. Etomidate is a cardiostable induction agent, but is associated with greater nausea and may inhibit adrenal function. Ketamine has sympathomimetic and analgesic activity but may cause tachycardia, increased pulmonary artery pressure, and disturbing hallucinations. Ketamine is most useful for inducing patients with hypovolemia, tamponade, or bronchospasm or as an adjunct.
It is critically important to understand the negative effects of induction on venous return and the caliber of airways. Induction in the supine position causes a 20% reduction in functional residual capacity (FRC), amounting to roughly 500 mL. This reduction in FRC occurs irrespective of the agents used (excepting ketamine), the imposition of paralysis, or the preservation of spontaneous ventilation and it persists for some hours after emergence. Airway calibers and resistance to airflow may be affected correspondingly. Patients with variable obstruction of major airways (e.g., anterior mediastinal mass effect) may convert to life-threatening complete obstruction on induction.
Induction impairs venous return by removal of the thoracic pump effect, imposition of positive-pressure ventilation, and vasodilation from induction agents. Increased intrathoracic pressure from positive end-expiratory pressure (PEEP; from the ventilator or auto-PEEP) will further reduce the gradient for venous return. Dynamic hyperinflation of lungs from auto-PEEP may exert pressure on the heart (with a closed chest), further impairing diastolic filling. Induction thus may unmask tamponade-like effects from large pleural or pericardial effusions or anterior mediastinal masses. Great vessels may be held open by traction during spontaneous ventilation but partially collapse under the weight of a large tumor on induction. The common mechanistic denominator, venous return to the heart, must be considered and defended at the time of induction.
Collapse of threatened major conducting airways may occur as a result of the decrease in FRC, as well as loss of the traction effect of inspiration that accompanies induction. Such “dynamic obstruction” may result from intraluminal masses, tracheomalacia, or large anterior mediastinal masses. Predicting which patients will lose patency with induction remains a judgment based primarily on symptoms and radiographic studies. Generally, patients who are asymptomatic at full expiration while supine will tolerate an intravenous induction. Coughing or dyspnea with this maneuver or tracheal lumens of less than 50% of normal by computed tomographic (CT) scan are treated more conservatively at my institution. Upright and supine flow-volume loops will sensitively illustrate dynamic obstruction but are of limited predictive value for induction. Intermediate concern may prompt a spontaneous-breathing (inhalation) induction, or an awake, topically anesthetized fiberoptic examination before induction. If the latter reveals nonreassuring anatomy, the stenotic region should be stented with an endotracheal tube before induction. Rigid bronchoscopy, patient repositioning, and jet ventilation (JV) are potential rescue maneuvers that should be immediately available. Short-acting agents for induction and muscle relaxation should be used initially in the event that resumption of spontaneous ventilation is required. Distal tracheal/carinal obstruction should prompt a more conservative approach because of the greater difficulty of emergently stenting such lesions with a tube or rigid bronchoscope. It is possible to place a DLT into a bronchus as a stent under local anesthesia. Placement of an LMA with topical anesthesia to the pharynx offers a convenient approach for awake fiberoptic examination of the entire trachea (Fig. 5-2). Local anesthetic delivered through the working port of the bronchoscope then can anesthetize the vocal cords and trachea. Rapid absorption by mucosal surfaces makes it important to be cognizant of the recommended thresholds for local anesthetic toxicity.
Patients at risk include those with large pleural or pericardial effusions, hypovolemia, severe obstructive lung disease, or large masses compressing the heart or great veins. Preemptive vasoconstrictors, intravascular volume expansion, use of cardiostable induction agents, patient positioning, avoidance of an epidural test dose, and use of graded inspiratory volumes and long expiratory times will preserve venous return in most inductions. When clinical and/or radiographic/echocardiographic evidence suggests higher risk, induction with maintenance of spontaneous ventilation is a more conservative approach. Preinduction drainage of pericardial or pleural fluid under local anesthesia should be considered in symptomatic (dyspneic) patients. Lower-extremity large-bore intravenous access is essential for patients with superior vena cava syndrome. Tension pneumothorax should be considered in patients with bullous emphysema and recalcitrant hypotension after induction. Intra-arterial blood pressure monitoring before induction is indicated for all high-risk patients, as is a plan for resuscitation should cardiovascular collapse occur.
Induction risk is also increased for patients with unfavorable airway anatomy, significant symptoms of gastroesophageal reflux, bronchospasm, or unstable coronary or severe valvular heart disease. Not uncommonly, competing priorities mandate compromise of one or the other concern. While aspiration risk argues for a rapid-sequence intravenous induction, this avenue may be contraindicated by difficult airway anatomy. Patients with unfavorable upper airway anatomy predictive of a difficult intubation (e.g., morbid obesity or micrognathia) should have an airway established under local anesthesia (e.g., LMA or awake fiberoptic intubation) before induction unless it is documented or apparent that the patient can be ventilated by mask. Use of short-acting agents (e.g., propofol or succinylcholine) is prudent whenever the airway is in question.
Failure to fully expire the preceding tidal volume at inspiration results in air trapping or auto-PEEP (Fig. 5-3). Auto-PEEP may lead to hemodynamic instability (including cardiac arrest) and barotrauma from dynamic hyperinflation. During OLV, excessive auto-PEEP in the dependent lung impairs gas exchange by diverting pulmonary blood flow to the nondependent lung. The incidence and severity of auto-PEEP roughly correlate with the severity of obstructive disease (Fig. 5-4) and is further exacerbated by the higher airflow resistance of DLTs and by inappropriate ventilator settings.
Options for lung isolation remain threefold: DLTs, BBs, and endobronchial intubation.
The default choice for lung isolation in most cases is a left DLT for its ease of insertion, comfortable margin for error, and capacity to deflate either lung depending on which lumen is clamped. Auscultation may be used to confirm position, but direct visualization by fiberoptic bronchoscopy is increasingly a standard of care (Fig. 5-5). Up to 30% of patients with DLTs positioned by auscultation require repositioning when subsequently examined by fiberoptic bronchoscopy.3 If the left DLT passes into the right main stem bronchus instead of the left, the fiberoptic bronchoscope may be used as a stylette via the bronchial lumen to guide the tube into the left bronchus after withdrawal to the trachea. Reconfirmation of DLT position should be performed after turning the patient to the lateral decubitus position.
Right-sided DLTs have a fenestration in the bronchial lumen for the right upper lobe that must be aligned by fiberoptic bronchoscopy (Fig. 5-6). Common indications include any surgery in which pathology exists or any surgery intended in the left main stem bronchus (e.g., left pneumonectomy, sleeve resection, bronchopleural fistula repair, or left single-lung transplant). The likelihood of displacement is greater for a right-sided DLT than for a left-sided DLT. Because of this, some practitioners prefer to use a left-sided DLT (or a BB) for left pneumonectomy and to withdraw the apparatus before division of the bronchus. Right-sided DLTs cannot accommodate an anomalously short right main stem bronchus.
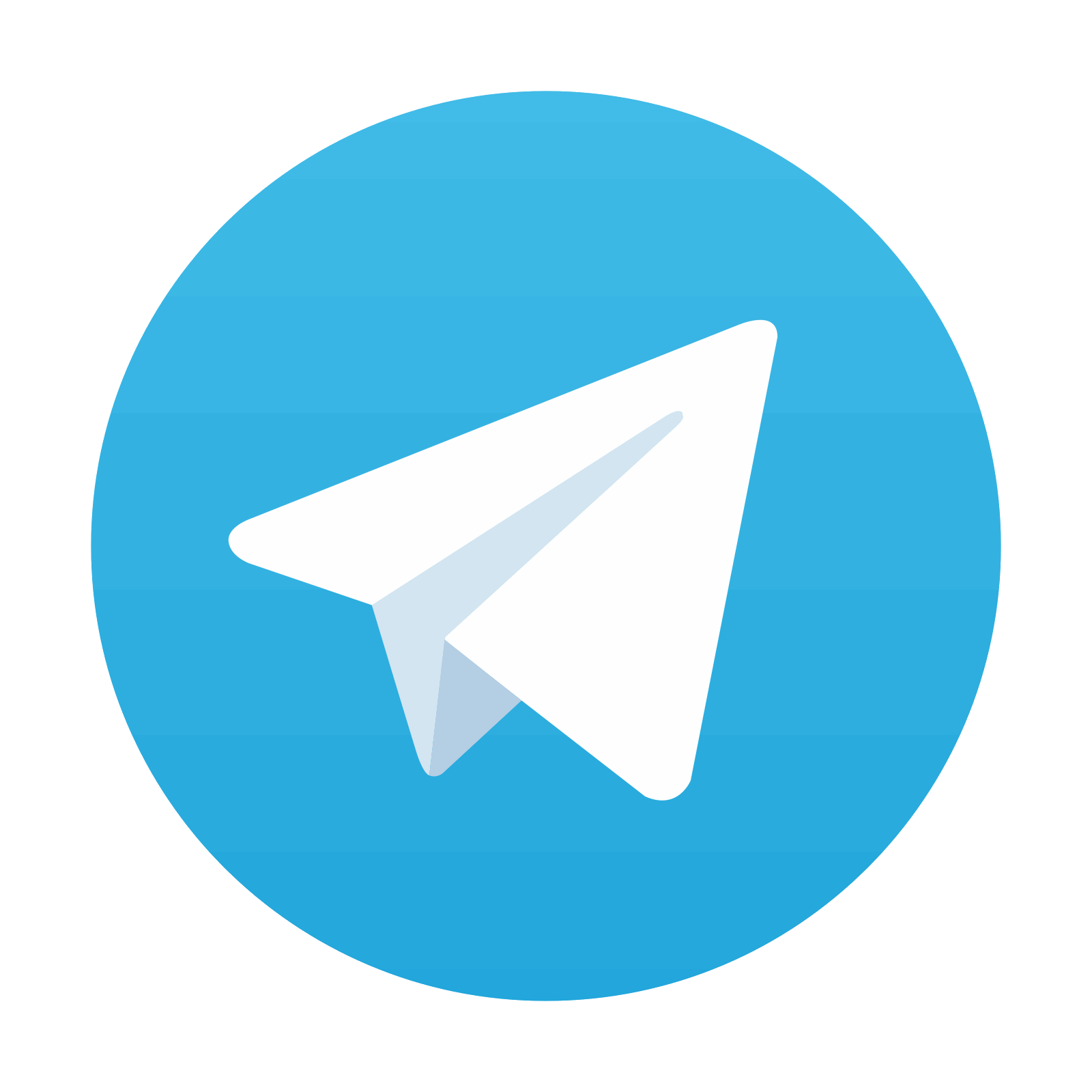
Stay updated, free articles. Join our Telegram channel

Full access? Get Clinical Tree
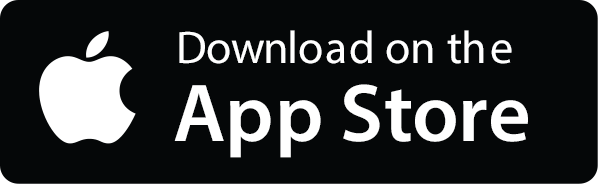
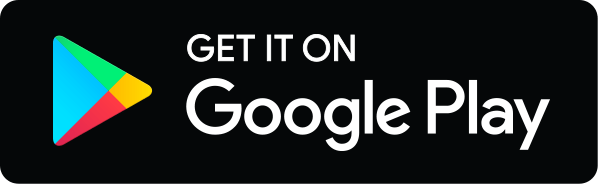