Abstract
This chapter discusses the roles of anesthesia and sedation for infants and children with pediatric heart disease. The goal is for providers that are caring for these patients to become comfortable understanding the myriad of options and techniques to support these patients. A variety of options will be explained and which situation is best for which options. It is essential that the team is able to carefully consider the risk/benefit ratio for any given patient for any given procedure.
Key Words
Anesthesia, Sedation, Perioperative Management, Anesthetic Risk
Pediatric cardiac anesthesiology is a dynamic field with its unique impact and challenges. Our field has grown from skilled anesthesiologists with an interest in cardiac patients, to the development in 2005 of the Congenital Cardiac Anesthesia Society, which eventually led to a proposal for formal training pathways in 2010. Our practice spans anesthesia and sedation for neonatal critical cardiac disease, palliation for children with cardiac disease with and without cardiopulmonary bypass (CPB), cardiac and lung transplantation, interventional cardiology, advanced imaging, patients with cardiac disease for noncardiac surgery, electrophysiology, and adult congenital heart disease. As outcomes from congenital cardiac surgery improve, over a million children and adults are living with corrected or palliated congenital heart disease; these patients with sometimes significantly altered physiology present challenges to the anesthesia community. Pediatric cardiac anesthesiologists are key members of the multidisciplinary team that cares for these patients.
Anesthesia Risk for Children With Congenital Heart Disease
Children with cardiac disease are at substantially increased risk when undergoing anesthesia when compared with children without cardiac disease. Specifically, infants with single-ventricle physiology, left ventricular outflow tract obstruction (LVOTO), cardiomyopathy, and pulmonary hypertension are at greatest risk.
A critical role of the cardiac anesthesiologist is to know and integrate these risk factors with the patient’s specific information to provide risk stratification and consultation. In many centers the number of needed procedures on patients with cardiac disease is greater than the availability of anesthesiologists who specialize in cardiac anesthesia, and therefore anesthesia for some lower-risk patients or procedures can be successfully performed by noncardiac anesthesiologists in consultation with cardiac anesthesiologists. Additionally, because anesthesia has significant risk for patients with cardiac disease, cardiac anesthesiologists often serve in the role of perioperative care coordinator, combining procedures to minimize anesthetics and improve care efficiency for our patients.
Preoperative Management
The cardiac anesthesiologist is part of the team that makes critical cardiac surgical planning decisions. Most centers have a surgical planning multidisciplinary conference to discuss upcoming surgeries and patients who may need repair. Patient history, physical examination, comorbidities, imaging, and preoperative testing results are reviewed and discussed. These data add to the anesthesiologist’s understanding of preoperative condition and physiology. The anesthesiologist’s attendance at this conference is essential for both obtaining information about upcoming cases and providing input for patient optimization before surgery.
Immediate standard anesthetic preoperative assessment includes a review of preoperative history, including symptoms, medications, allergies, and imaging studies. Additionally, the patient/family should be queried about anesthesia-associated issues, including recent infection, malignant hyperthermia, difficult airway, and nothing by mouth (NPO) timing.
Physical examination before cardiac anesthesia focuses on areas that may cause intraoperative challenges, as well as areas that may be modified before surgery. Head and neck examination looks for dysmorphism and possible difficult airway risk. Indeed, the incidence of difficult airway is higher in children with congenital cardiac disease, and difficult airway can complicate an already complex anesthesia for this patient population. Loose teeth should be noted and discussed with parents. Excessively loose primary teeth are often removed under anesthesia to prevent accidental dislodgement and aspiration. Nares should be examined for signs of acute viral infection, and chest examination should look for signs of respiratory compromise. Excessive pulmonary blood flow lesions put children at increased risk for reactive airways disease and infection; these pathologic conditions should be addressed and optimized before surgery. Acute viral infection is common in children, especially in winter months, and it may increase perioperative risk of pulmonary complications. Risk of pulmonary complications from viral infections should be weighed against the urgency of surgery. Although cardiac examination often confirms the findings associated with recent imaging studies, it should not be minimized. Abdominal examination can reveal distention, ascites associated with high venous pressure, and organomegaly from elevated right heart pressures or left-to-right (L-R) shunt. Pulses and perfusion are useful to assess before induction because children with compromised ventricular function are at risk for cardiovascular instability during induction and before placement of invasive lines. Palpation of the arterial pulse can provide real-time information about blood pressure when noninvasive cuff cycling is slower than the fluctuating clinical situation.
Because many of these patients have had many anesthetics, parents are often knowledgeable about anesthetic issues. They may have preferences about induction or information about previous positive or negative anesthetic experiences. Many centers include anesthetic evaluation and consultation during the preoperative visit performed in the days before surgery. This approach allows for a more deliberate assessment and communication than the immediate preoperative period. It provides time for anesthetic fine-tuning optimization, including shortened NPO times for patients with fluid-sensitive physiology or holding on the day of surgery of medications that may impact the anesthetic or hemodynamics.
There is a trend toward less preoperative laboratory study. In the relatively healthy outpatient, few laboratory values will impact preoperative planning. A hemoglobin test is needed before initiation of CPB to calculate hemodilution hemoglobin value. Because arterial blood gas level is typically obtained after induction and placement of invasive monitoring lines, hemoglobin value can be obtained at that time. Electrolytes, coagulation assays or tests to examine end-organ function are typically not indicated for outpatients unless there is high suspicion of abnormalities that will prompt preoperative changes in care or operative plan. Two required laboratory values are pregnancy test in menstruating females and preoperative type and screen for CPB cases. Because type and screen testing can take hours, it may need to be performed before the day of surgery to avoid unacceptable delays and length of anesthetic. For inpatients, as well as patients who have end-organ dysfunction, the appropriate additional laboratory tests are ordered after surgical and anesthetic review.
Intraoperative Management
General Principles
The principles underlying anesthetic management of children with congenital heart disease are based on an understanding of each disease process, pathophysiology, and a working knowledge of anesthetic effects and other pharmacologic interventions on a specific patient’s condition. Cardiac and great vessel structural abnormalities may result in shunt, outflow obstruction, regurgitation, common mixing, or a combination of lesions. Interactions between those lesions determine the clinical presentation and anesthetic considerations. Anesthesia and surgery will impact patient physiology, setting the stage for potential cardiovascular instability. Without being overly simplistic, once the defect characteristics, predominant physiology, and impact of surgery and anesthesia are understood, anesthesia for cardiac and noncardiac operations can be competently performed using a physiologic approach ( Table 20.1 ).
Condition | HR | Contractility | Preload | Pulmonary Vascular Resistance | Systemic Vascular Resistance |
---|---|---|---|---|---|
Shunts | |||||
L → R (all types) | Normal | Normal | ↑ | ↑ | ↓ |
Ventricular septal defect R → L | Normal | Normal | Normal | ↓ | ↑ |
Obstructive | |||||
Aortic stenosis | ↓ * | Normal-↑ | ↑ | Normal | ↑ |
Aortic coarctation | Normal | Normal | ↑ | Normal | ↓ |
Mitral stenosis | ↓ | Normal-↑ | ↑ | Normal-↓ | Normal |
Pulmonary stenosis | ↓ | ↑ | ↑ | ↓ | Normal |
Dynamic subaortic obstruction | ↓ * | ↓ * | ↑ | Normal | Normal-↑ |
Dynamic subpulmonic obstruction | ↓ | ↓ * | ↑ | ↓ | Normal |
Regurgitant | |||||
Aortic insufficiency | Normal-↓ | Normal-↓ | ↑ | Normal | Normal-↓ |
Mitral regurgitation | Normal | Normal-↓ | ↑ | Normal-↓ | ↓ |
Pulmonary insufficiency | Normal-↓ | Normal | ↑ | ↓ | Normal |
Tricuspid regurgitation | Normal | Normal | ↑ | ↓ | Normal |
Critical to understanding congenital cardiac physiology and anesthesia is the concept of shunting. A shunt is defined as an abnormal connection between two chambers or vessels that allows passage of blood. The direction and magnitude of shunting depends on defect size and pressure gradient between the chambers connected to that defect. Shunting is typically fixed in a restrictive defect, whereas it varies with the relative vascular resistances of the connected structures in a nonrestrictive defect.
Cyanotic patients with right-to-left (R-L) shunt have too little pulmonary blood flow. This makes them less tolerant of airway obstruction or apnea during sedation or anesthesia. Systemic vasodilation may worsen a R-L shunt and cyanosis. R-L shunts slow the rate of inhalation induction by delaying the equilibration of partial pressure of alveolar-to-inspired anesthetic. This delay is more pronounced with the less soluble inhalation agents.
Depending on the type and size of defect, lesions with L-R shunt are generally better tolerated under anesthesia. Nevertheless, depending on location of shunt, increasing L-R shunt by maneuvers that decrease pulmonary vascular resistance (PVR) may lead to some degree of systemic hypotension with reduction of coronary perfusion. Uncorrected L-R shunting and pulmonary overcirculation over the long term may produce changes that increase pulmonary vasculature reactivity, especially when weaning from bypass. The onset of intravenous (IV) anesthetic is slower in L-R shunting, whereas inhalational anesthetic pharmacokinetics remain unchanged.
Right heart obstructive lesions are either dynamic or fixed and may create R-L shunt where pulmonary to systemic defect is present. The degree of cyanosis will depend on the degree of right-sided obstruction, PVR, and systemic vascular resistance (SVR). It is advantageous to avoid systemic vasodilation. Reducing dynamic obstruction (by decreasing contractility) is helpful by reducing R-L shunting and improving cyanosis during anesthesia.
Patients with left heart obstructive lesions have decreased cardiac output and systemic perfusion. Consequently, they can rapidly decompensate with anesthesia and surgical manipulation. For the ductile-dependent left heart obstructive lesions, maintenance of prostaglandin infusion is necessary. An increase in the fraction of inspired oxygen (FiO 2 ) with concomitant decrease in PVR leads to steal from systemic circulation, which could be detrimental.
Management of regurgitant lesions (e.g., atrial-ventricular canal, Ebstein anomaly) includes reduction of regurgitant fraction by decreasing afterload (PVR for right-sided lesions, SVR for left-sided lesions), improvement in ventricular contractility, and heart rate increase.
Mixing lesions (e.g., single ventricle, total anomalous pulmonary venous return, tricuspid atresia, truncus arteriosus) are characterized by complete mixing of deoxygenated systemic venous blood and fully oxygenated pulmonary venous blood. Balance between systemic and pulmonary vascular resistances determines arterial blood desaturation. In a patient with single-ventricle physiology, PVR and SVR are the key determinants of hemodynamic stability. The desirable ratio of pulmonary blood flow to systemic perfusion (Q p :Q s ) of approximately 1 (balanced) leads to an oxygen saturation as measured by pulse oximetry (SpO 2 ) of approximately 80%. This delicate balance of PVR/SVR will place a patient with single-ventricle physiology at a higher risk of myocardial ischemia during induction of anesthesia and surgical manipulation. Factors affecting the balance of PVR/SVR are listed in Table 20.2 .
Factors Increasing PVR | Factors Decreasing PVR | Factors Increasing SVR | Factors Decreasing SVR |
---|---|---|---|
|
|
|
|
The anesthesiologist will work toward hemodynamic goals by manipulating factors affecting cardiac output, systemic and pulmonary flow, and oxygen delivery with consideration of the specific anatomy and physiology as well as factors affecting the arterial O 2 content, especially the hemoglobin level (see Table 20.1 ). Specific anesthetic strategies will be further discussed later in the chapter.
Physiologic Monitoring
Noninvasive monitors are placed before anesthesia induction. They include a five-lead electrocardiography system (one lead for each extremity and one precordial lead in V 5 position), two pulse oximetry probes (preductal and postductal), and an appropriately sized blood pressure cuff. A nasopharyngeal temperature probe is inserted after intubation and is most closely reflective of brain temperature. Depending on the child’s condition and planned procedure, additional monitoring is necessary: an indwelling arterial catheter, a central line, and a urinary catheter. A second temperature monitor is added (often rectal to reflect core temperature) when planning to go on bypass.
SpO 2 and capnography (end-tidal carbon dioxide [ETCO 2 ]) provide instantaneous feedback concerning adequacy of oxygenation and ventilation. Capnography is of great value and remains the gold standard to confirm tracheal intubation. SpO 2 and ETCO 2 direct ventilatory and hemodynamic adjustments to optimize Q p :Q s in patients with shunts and pulmonary artery bands. Differences in preductal and postductal SpO 2 values indicate shunting through a patent ductus arteriosus (PDA). SpO 2 and ETCO 2 can also be used to assess the adequacy of cardiac output. Low systemic arterial saturation or inability of the oximeter probe to register a pulse wave may be a sign of a very low cardiac output and high systemic resistances. SpO 2 values less than 80% often overestimate arterial oxygen saturation, which make other means of tissue oxygenation measurement necessary.
Near-infrared spectroscopy (NIRS) measures regional oxygen saturation. Whereas the oxygen saturation displayed by the pulse oximeter represents only the pulsatile component of light transmitted to tissues, signal received by the NIRS represents the nonpulsatile blood (75%) as well as pulsatile blood (20%). Normal range of regional cerebral oxygen saturation (cerebral rSO 2 ) for a noncyanotic patient is 60% to 85%. The somatic rSO 2 is generally 10% higher than the cerebral. Any fall of rSO 2 20% or more below the baseline obtained before induction warrants interventions aimed at improving oxygen delivery and/or decreasing oxygen consumption to restore rSO 2 ( Table 20.3 ). Numerous studies have indicated good correlation between rSO 2 and central venous oxygen saturation (SvO 2 ).
Factors Raising Cerebral NIRS | Factors Reducing Cerebral NIRS |
---|---|
↑ Hemoglobin | ↑ Oxygen consumption |
↑ PaO 2 | ↑ ICP (brain edema, decrease venous drainage) |
↑CPB flow or cardiac output | ↑ Sweep rate/hyperventilation |
↑ Mean arterial pressure (↑ cerebral perfusion pressure) | |
↓ Sweep rate (↑ CO 2 ) |
Continuous monitoring of arterial pressure is possible only through an indwelling intraarterial catheter. Previous procedures or currently planned procedure, such as radial artery cut-down, Blalock-Taussig shunt, or a subclavian flap for coarctation of the aorta repair, can impact the arterial pressure monitoring site. Other sites available for cannulation include ulnar, femoral, axillary, or umbilical arteries. Posterior tibial or the dorsalis pedis arterial catheters function poorly after CPB and do not reflect central aortic pressure when the temperature of the distal extremities remains low. The optimal position of the umbilical artery catheter tip is just above the aortic bifurcation between L3 and L4 or preferably, high above the diaphragm between T6 and T9. To decrease the risk of thrombosis of the renal and mesenteric arteries, the tip should not be left in the area of T12 to L2.
Major cardiovascular surgeries require some type of central venous pressure (CVP) monitoring. The CVP catheter is also used for administration of fluid, drugs, and blood products. Alternatively, directly placed transthoracic atrial lines may be used to deliver medications and to evaluate filling pressures during the separation from CPB and the postoperative period. Most often the right internal jugular is accessed. The left internal jugular is avoided in case of persistent left superior vena cava. Other percutaneous options include cannulation of subclavian, femoral, external jugular, or axillary veins. The use of real-time ultrasound guidance reduces the time and number of needle insertions required for successful venous cannulation and decreases the rate of complication compared to the use of anatomic landmarks. The greatest benefit was found for internal jugular cannulation. In case of preexisting peripherally inserted central catheters anesthesiologists should ascertain that the catheter is at least 3 French for rapid fluid and blood infusion, and that the tip is positioned centrally for infusion of vasoactive agents. For patients with single-ventricle physiology, superior vena cava thrombosis could be catastrophic, and femoral or atrial lines are sometimes the preferred options.
Another reliable source of central venous access is the umbilical vein. It is cannulated within the first week after birth. To use it as a central line, the optimal position of the tip should be in the right atrium or high in the inferior vena cava.
The utility of intraoperative echocardiographic assessment with Doppler color flow imaging in predicting outcome after repair of congenital heart defect was first demonstrated in 1989 by Ungerleider and associates, using an epicardial echocardiography probe. Since then, many authors have published strong evidence supporting transesophageal echocardiography (TEE) use for all cardiac defects requiring repair under CPB. Advances in probe technology make multiplane TEE use routine to confirm or revise the initial diagnosis and assess the heart function during the prebypass period, even in small neonates. In the postbypass period, TEE assesses repair adequacy, presence of residual defects, and ventricular filling and function. Bettex et al., in a series of 865 intraoperative TEE examinations for congenital heart defect surgeries, found that alteration in surgical management occurred in 12.7% of cases, including 7.3% repeat bypass run; alteration in medical management occurred in 19.4% of cases. The occurrence of airway compression or hemodynamic instability after insertion should prompt the removal of the TEE probe; using an intraoperative epicardial probe should be considered instead.
Preoperative Medication
For infants less than 6 month of age, no premedication is necessary. Children older than 9 months may require a dose of midazolam given orally 0.3 to 0.7 mg/kg to a maximum of 20 mg, 10 to 20 minutes before induction, to provide effective anxiolysis. If an IV access is available, then midazolam 0.05 mg/kg up to 4 mg may be given a few minutes before operating room transfer. On occasion, a child or adult with developmental delay and without IV access is given ketamine 4 to 5 mg/kg with glycopyrrolate 20 mcg/kg and midazolam 0.1 mg/kg via the intramuscular route; oral ketamine (5 mg/kg) and midazolam (0.5 mg/kg) is also an option for an older child with developmental delay or a patient who requires denser preoperative sedation.
Induction and Maintenance of Anesthesia
Although it is important to comprehend the complexities of shunt and vascular resistance changes, airway and ventilation effects on the cardiovascular system are of equal importance during induction and maintenance of anesthesia. Selection of an induction technique depends on the cardiac defect, the degree of cardiac dysfunction, and the level of preinduction sedation. Anesthetic agent titration is more important than specific anesthetic technique in patients with reasonable cardiac reserve. Successful and safe induction is possible with volatile agents such as sevoflurane and nitrous oxide or IV agents such as etomidate, fentanyl, midazolam, and ketamine.
Induction method choices include IV (when an IV catheter is present) and inhalational. Skillful airway management and adequacy of ventilation are essential during induction no matter what induction method is used.
The use of inhalation induction should be reserved for children with reasonable cardiac reserve. Sevoflurane is currently the only halogenated anesthetic used for induction in the United States. Sevoflurane may be combined with up to 50% of nitrous oxide during initial induction to speed induction and decrease patient response to pungent Sevoflurane odor. Sevoflurane increases the heart rate slightly, mildly depresses myocardial contractility, and lowers SVR and PVR. Depression of myocardial contractility and blood pressure with volatile agent use is more pronounced in neonates compared to older children and adults due to the effect of volatile agents on calcium channels in the immature cardiovascular system. Pronounced bradycardia is frequent during sevoflurane induction of patients with trisomy 21 with and without heart defects. Inhalation induction is generally well tolerated by most children, including some with cyanotic defects such as tetralogy of Fallot (TOF).
In situations in which a higher minimum alveolar concentration (MAC) is used during prolonged sevoflurane induction, cardiovascular compromise or collapse can occur, in particular when positive pressure ventilation is applied indiscriminately with excessive depth of anesthesia. Careful titration of anesthetic agent and depth is required.
After anesthetic induction, IV access is established or augmented as appropriate. A nondepolarizing muscle relaxant is usually administered, and an IV opioid and/or inhalation agent is chosen for maintenance of anesthesia. The child is preoxygenated before intubation, and a nasal or oral endotracheal tube is carefully positioned. Some degree of alveolar preoxygenation is recommended, even in an infant whose systemic perfusion might be jeopardized by PVR decrease. This maneuver delays desaturation during intubation. If the child arrives in the operating room with an endotracheal tube in place, it should be changed if the benefit of a new tube outweighs the reintubation risk.
Intravenous induction is preferable when a child arrives with a well-functioning preexisting IV catheter. For neonates and sick children, IV opioid plus relaxant induction is most prevalent. In patients with limited cardiac reserve, inhalation agents are less well tolerated as a primary anesthetic, especially after a CPB. Titrated doses of fentanyl or sufentanil are excellent induction and maintenance anesthetics for this group of patients because these opioids generally have a low impact on hemodynamics. After repair of a congenital heart defect, the hemodynamic effects of fentanyl at a dose of 25 mcg/kg with pancuronium given to infants in the postoperative period show no change in left atrial pressure, PVR, and cardiac index. There is only a small decrease in SVR and mean arterial pressure (MAP). Higher doses of fentanyl at 50 to 75 mcg/kg with pancuronium result in a slightly greater fall in arterial pressure and heart rate. Fentanyl has also been shown to block stimulus-induced pulmonary vasoconstriction and contributes to pulmonary circulation stability in neonatal congenital diaphragmatic hernia repair. Thus fentanyl use may be extrapolated post CPB in newborns with pulmonary vascular responsiveness. The ultra–short-acting opioid remifentanil has not shown any significant advantage over fentanyl. It is often possible to titrate low-dose inhalational agents with opioids and maintain hemodynamic stability. The combination of fentanyl/midazolam as an alternative to fentanyl/low-dose volatile agents is frequently used for maintenance of anesthesia. But this results in lower cardiac output due to a decrease in heart rate. Despite a widespread safety margin exhibited by opioids, it should be noted that patients with marginally compensated hemodynamic function sustained by endogenous catecholamines may decompensate with higher-dose opioids.
Isoflurane or sevoflurane may be used for maintenance of anesthesia. Inhalation agents can provide both anesthesia and vasodilation when a vaporizer is connected to the circuit during CPB. Some studies have found volatile agents to be neuroprotectors on CPB. Isoflurane and sevoflurane maintain the ratio Q p :Q s when the controlled ventilation is adequate.
Dexmedetomidine is a highly selective alpha-2 adrenergic receptor agonist that produces sedation. It is useful as an adjunct to a volatile agent or narcotic-based anesthetic to decrease surgical stress response. The reduction in central sympathetic outflow can lead to bradycardia and hypotension. Dexmedetomidine bolus of 0.5 mcg/kg over 10 minutes, followed by an infusion of 0.5 mcg/kg/h attenuates hemodynamic and neuroendocrine response to surgery and CPB. Dexmedetomidine is associated with a reduction in the incidence of postbypass ventricular and supraventricular tachyarrythmias. Because of its antiarrhythmic effects, it is not an ideal sedative for electrophysiologic studies.
Ketamine used to be popular for anesthetic induction in patients with cyanotic conditions because it increases SVR and diminishes the magnitude of R-L shunting. Administration of ketamine can be IV or intramuscular. Ketamine also increases salivation. It exerts a central nervous system–mediated sympathetic activation and catecholamine release, which makes ketamine an attractive anesthetic for patients with decreased function. However, it is a direct myocardial depressant and should be used with caution in very sick patients with exhausted sympathetic responses.
Another option for induction in hemodynamically unstable patients is etomidate. This imidazole derivative does not change the hemodynamic parameters at clinical doses. Etomidate has the undesirable effect of possible adrenal suppression, particularly when repeated doses are used.
Cardiopulmonary Bypass
For cardiac anesthesiologists, CPB is not a passive time. It can test the quality of interteam communication, even during a routine case. This is especially true when monitors or laboratory test results show anomalies (e.g., decreased cerebral and/or somatic NIRS, abnormal arterial blood gas values, worsening of lactic acidosis, elevated central venous pressure) and when problems occur as a result of poor myocardial protection or residual defects. Good communication is essential during separation from CPB and the decision-making process regarding mechanical circulatory support when things do not go according to plan (e.g., native cardiac output not sufficient to terminate CPB, difficult to control arrhythmia).
The major advances in pediatric CPB are improved circuitry, technology, and management strategies to reduce systemic inflammatory response and improve organ protection. Oxygenators specifically designed for neonates were developed and combined with an integrated arterial filter. There has been a wider adoption of mast-mounted pumps that are closer to the patient to shorten the length of the tubing line. These improvements provided significant reduction in prime volume and foreign surface area.
Hemodilution
Hemodilution to a hematocrit value of 24% or higher is associated with reduced lactate levels and better neurologic outcomes. Hemodilution to a hematocrit value of 25% versus a value of 35% during hypothermic CPB in infant surgery for two-ventricle repair shows no difference in hemodynamics during the procedure.
Temperature
The three ranges of hypothermia used are mild (30°C to 35°C), moderate (22°C to 30°C), and deep (<22°C). No proven benefit has been associated with normothermic CPB. Hypothermic CPB is used to preserve organ function during cardiac surgery. The scientific rationale for the use of hypothermia rests primarily on temperature-mediated reduction of metabolism. Whole body oxygen consumption metabolic rate, including cerebral, decreases by a factor of 2 to 2.5 for every 10°C reduction. Hypothermia significantly decreases excitatory amino acids release, suggesting an additional mechanism for protective effect.
Deep hypothermia is generally reserved for neonates, infants, and children requiring complex cardiac repair. Deep hypothermic circulatory arrest (DHCA) allows removal of atrial and/or aortic cannula. Using this technique increases precision and ease of surgical repair through a bloodless and cannula-free operative field. Duration of DHCA should be under 41 minutes to reduce permanent neurologic damage, according to the Boston Circulatory Arrest Trial. During DHCA it is recommended to cool evenly and slowly over at least 20 minutes to reduce risk of neurologic injury. The head must be covered with ice packs to avoid brain rewarming during DHCA.
Antegrade Cerebral Perfusion
Also known as regional cerebral perfusion, antegrade cerebral perfusion (ACP) may be used in lieu of DHCA. An arterial cannula flows into the innominate artery after direct insertion or via a prosthetic graft. Only the brain is actively perfused. To prevent the risk of cerebral hypoperfusion and hypoxemia, an ACP flow rate of at least 40 mL/kg/min is necessary for adequate flow to reach the left hemisphere via the circle of Willis. Furthermore, ACP times are frequently longer than DHCA, so adequate cerebral flow, deep hypothermia, and monitoring of NIRS are essential for brain protection.
pH-Stat Strategy
pH-stat blood gas management is preferred for deep hypothermic CPB, particularly during cooling. Hypothermia-induced alkalosis is corrected by adding CO 2 to the circuit or by reducing CO 2 removal. This strategy provides cerebral vasodilation and a more even cerebral cooling. Review of published data suggests better neurodevelopmental outcomes for pH-stat compared to alpha-stat management in pediatric cardiac surgery and possibly a reduction in intensive care unit (ICU) stay.
Perfusion Flow
Full bypass perfusion flow rate for a 2- to 7-kg patient is 120 to 200 mL/kg/min. For patients above 20 kg, it is 50 to 75 mL/kg/min. Monitors of adequate CPB flow include MAP, SVO 2 , NIRS, lactate, and acid-base status. Vasodilators such as phentolamine, nitroprusside, or nitroglycerine may be used during hypothermic bypass to promote even organ perfusion, prevent hypertension on bypass, improve oxygen delivery, minimize acidosis, and improve patient outcome. During separation from bypass, excessive vasodilation may be reversed with low-dose vasopressin infusion if necessary.
Discontinuation of Cardiopulmonary Bypass
When weaning from CPB, blood volume is assessed by direct cardiac visualization and monitoring right and/or left atrial filling pressures. When filling pressures are adequate, patient fully warm, acid-base status normalized, and heart rate adequate and in sinus rhythm, CPB venous drainage is decreased, and the patient is weaned from bypass. The arterial cannula is temporarily left in place so that infusion of residual pump blood can be used to optimize filling pressures. Myocardial function is assessed by direct cardiac visualization, left and/or right atrial pressures, percutaneous jugular venous pressure, or echocardiography. After complex congenital heart defect repair the anesthesiologist and surgeon may have difficulty separating a patient from CPB. Under these circumstances the differential diagnosis may include an inadequate surgical result with a residual defect, pulmonary artery hypertension, right or left ventricular dysfunction, arrhythmia, bleeding, or any of these combined. TEE may be used to provide an intraoperative image of structural or functional abnormalities and to assist in the evaluation of the postoperative cardiac repair. Strategies will involve identification and correction of reason for failure to wean, including consideration of return to hypothermia and repair of residual defect. Leaving the operating room with a significant residual structural defect adversely affects survival and increases patient morbidity.
If the patient is unable to come off bypass due to early postoperative cardiac failure and has severe metabolic acidosis and high inotropic and/or pressor requirement, postoperative mechanical support with extracorporeal membrane oxygenation (ECMO) may be considered. Typically the same aortic and right atrial cannulae are used for conversion from bypass to ECMO.
Ultrafiltration
Conventional ultrafiltration or modified ultrafiltration (MUF) is used to remove excess total body water and reduce proinflammatory factors in almost all pediatric cardiac surgical centers in the United States. Conventional ultrafiltration is performed during bypass, whereas MUF is performed for 10 to 15 minutes after the discontinuation of bypass ( Figs. 20.1 and 20.2 ). MUF filters the patient’s blood volume and improves hemodynamic and pulmonary performance. After the patient is weaned from CPB, blood is removed from the patient via aortic cannula, fed through an ultrafilter, and then returned via venous cannula to the right atrium. A constant left or right atrial pressure is maintained for hemodynamic stability. Ultrafiltration is carried out with end points being either time of 15 to 20 minutes or hematocrit value of approximately 40%.


Anticoagulation and Hemostasis
CPB is a significant thrombogenic stimulus, requiring anticoagulation with heparin. The effect of heparin is followed by activated clotting time (ACT) monitoring. The effect of heparin is primarily due to antithrombin III coupling; because there are age-related and quantitative differences in procoagulants and inhibitors, heparin dosing may be variable. Maintaining an ACT above 400 seconds is recommended. Heparin is neutralized by protamine after CPB and MUF, but protamine excess may actually contribute to postoperative bleeding.
Bleeding after CPB is not an unusual occurrence. Neonates and young infants with congenital heart disease have low circulating levels of procoagulants and inhibitors. Thrombogenic and dilutional effects of CPB further contribute to hemostatic abnormalities. Furthermore, DHCA causes increased clotting and fibrinolytic activities. There are pharmacologic interventions aimed at reducing bleeding after CPB. Desmopressin acetate and antifibrinolytics aminocaproic acid or tranexamic acid have been tried with variable success. However, the most impressive results have been demonstrated with the use of aprotinin. It is a proteinase inhibitor with antifibrinolytic properties at low concentrations and a kallikrein inhibitor at higher levels. Aprotinin is not available in the United States at the time of this writing due to studies associating it with renal failure in adults.
When postoperative bleeding occurs, the surgeon should first attempt to identify any source of surgical bleeding. Next, adequate protamine reversal of heparin is assessed by ACT and laboratory evaluation. In general, standard coagulation tests show prolongation of prothrombin time, partial thromboplastin time, hypofibrinogenemia, and dilution of other procoagulants, as well as prolonged bleeding time in patients with and without bleeding. The most common reason for persistent bleeding is platelet dysfunction. Administration of platelets is warranted in those bleeding situations. Routine administration of blood products to correct laboratory coagulation abnormalities in the absence of bleeding is not indicated. If bleeding persists after platelets have been given, reassessment and a repeat of platelet infusion or cryoprecipitate administration may be beneficial, whereas fresh frozen plasma has not been found to be effective. When facing persistent bleeding despite repeated previously described interventions, recombinant activated factor VII may be used as a last resort.
Patient Transport and Handover to Intensive Care Unit
After surgery, when the patient is deemed stable for transport, report should be given to the ICU staff before departing from the operating room. All transport equipment is checked beforehand to confirm proper functioning. The patient is carefully transferred to the transport bed. The trip to the ICU requires full, continuous monitoring without any interruption of vasoactive medication infusion. The patient is manually ventilated at the appropriate FiO 2 . If there is pulmonary hypertension that requires the use of nitric oxide post bypass, nitric oxide should not be interrupted during transport.
The complex nature of pediatric cardiac defects and procedures necessitates a structured and efficient handover process to ensure patient safety and continuity of care ( Box 20.1 , Fig. 20.3 ). A systematic review by Segall et al. offers the following recommendations: “(1) standardize processes (e.g., using checklists and protocols); (2) complete urgent clinical tasks before information transfer; (3) allow only patient-specific discussions during verbal handovers; (4) require that all relevant team members be present; and (5) provide training in team skills and communication.” It is imperative that each institution develops a handover process tailored to its setting. The sequence of events during a routine cardiac surgery done under CPB is listed in Box 20.2 .
All Inpatient Transfers to Operating Room (Complete #1)
1
Preoperatively a
a Completed the morning of surgery before patient leaves assigned unit.
- •
PICU RN to obtain a blank copy of the clinical handoff tool
- •
PICU RN to call NICU or Floor RN and obtain verbal handoff/report
- •
Identify/ensure that CHD-specific clinical pathway has been implemented and is available (when applicable)
2
Postoperatively
- •
Anesthesia/CV surgeon/PICU attending/APP/RN/RT (all present)
- •
Patient is identified, “time-out” performed at bedside
(Allow ≈ 5 min for admitting RN to attach patient to monitor and perform rapid CV examination → ensure hemodynamic stability, move to next step)
- •
Diagnosis, past medical/surgical history, preoperative medications, planned procedure
- •
Surgical or catheterization approach/technique
- •
CPB, cross-clamp time(s)
- •
Ventilation (identification of high-risk airway), vasoactive drops, rhythm concerns (pacemaker settings), bleeding concerns, IV access
- •
Recently administered blood products, medications, and time of last dose of ABX
- •
Discuss goals for care and patient management in the immediate postoperative period
- •
Answer any questions
ABX, Antibiotics; APP, advanced practice provider; CHD, congenital heart disease; CPB, cardiopulmonary bypass; CV, cardiovascular; IV, intravenous; NICU, neonatal intensive care unit; PICU, pediatric intensive care unit; RN, registered nurse; RT, respiratory therapist.

- •
Standard monitors
- •
NIRS cerebral + somatic
- •
Induction/IV/intubation
- •
Maintenance of anesthesia
- •
IV augmentation + antibiotic if not already administered
- •
Arterial line
- •
Baseline laboratory values (ABG, ACT, TEG)
- •
Aminocaproic acid/tranexamic acid
- •
Central venous line
- •
Gastric suction, then TEE
- •
Median sternotomy
- •
Heparin + ACT
- •
Aortic cannulation
- •
Venous cannulation
- •
CPB initiation + cooling
- •
Aortic cross-clamp + cardioplegia
- •
Surgical repair ± additional cardioplegia
- •
Cross-clamp release
- •
Ionotrope ± vasodilator
- •
Rewarming, pacing wires, atrial lines
- •
TEE
- •
End CPB (hemodynamically stable, warm, adequate laboratory values)
- •
MUF
- •
Protamine, then ACT
- •
Hemostasis + chest closure
- •
Transport to ICU and handover
ABG, Arterial blood gas; ACT, activated clotting time; CPB, cardiopulmonary bypass; ICU, intensive care unit; IV, intravenous; MUF, modified ultrafiltration; NIRS, near-infrared spectroscopy; TEG, thromboelastography; TEE, transesophageal echocardiography.
Specific Anesthetic Considerations
According to the Society of Thoracic Surgeons Congenital Heart Surgery Database, nine congenital heart operations constitute approximately 50% of reported cases. It is beyond the scope of this chapter to address anesthetic approach to all surgical procedures performed on children with congenital heart defects. Instead, we will concentrate on most of these nine defects with principles applicable to the vast majority of procedures.
Anesthesia for Simple Open-Heart Procedures
“Simple” open-heart procedures include those defects that involve relatively straightforward surgical repair, uncomplicated hemodynamics, minimal to moderate postoperative intensive care, and mechanical ventilation or no mechanical ventilation. Simple is a relative term. A case that may be classified as simple may unexpectedly become more complicated ( Table 20.4 , Box 20.3 ). Examples of simple open-heart procedures include repairs of atrial septal defect (ASD), ventricular septal defect (VSD), TOF, and atrioventricular (AV) canal defect in otherwise healthy children with good cardiopulmonary reserve. ASD closure is often performed in the cardiac catheterization laboratory, so it is less often repaired surgically. Patients may present with a range of symptoms from no medical management, minimal management with afterload reduction or diuretic, to congestive heart failure with minimal reserve. The latter will require a different level of care, despite the relatively simple surgical procedure.
Cardiac Lesion | Low Risk | Moderate Risk | High Risk |
---|---|---|---|
Structural lesions |
|
|
|
Pulmonary hypertension |
|
| |
Miscellaneous |
|
|
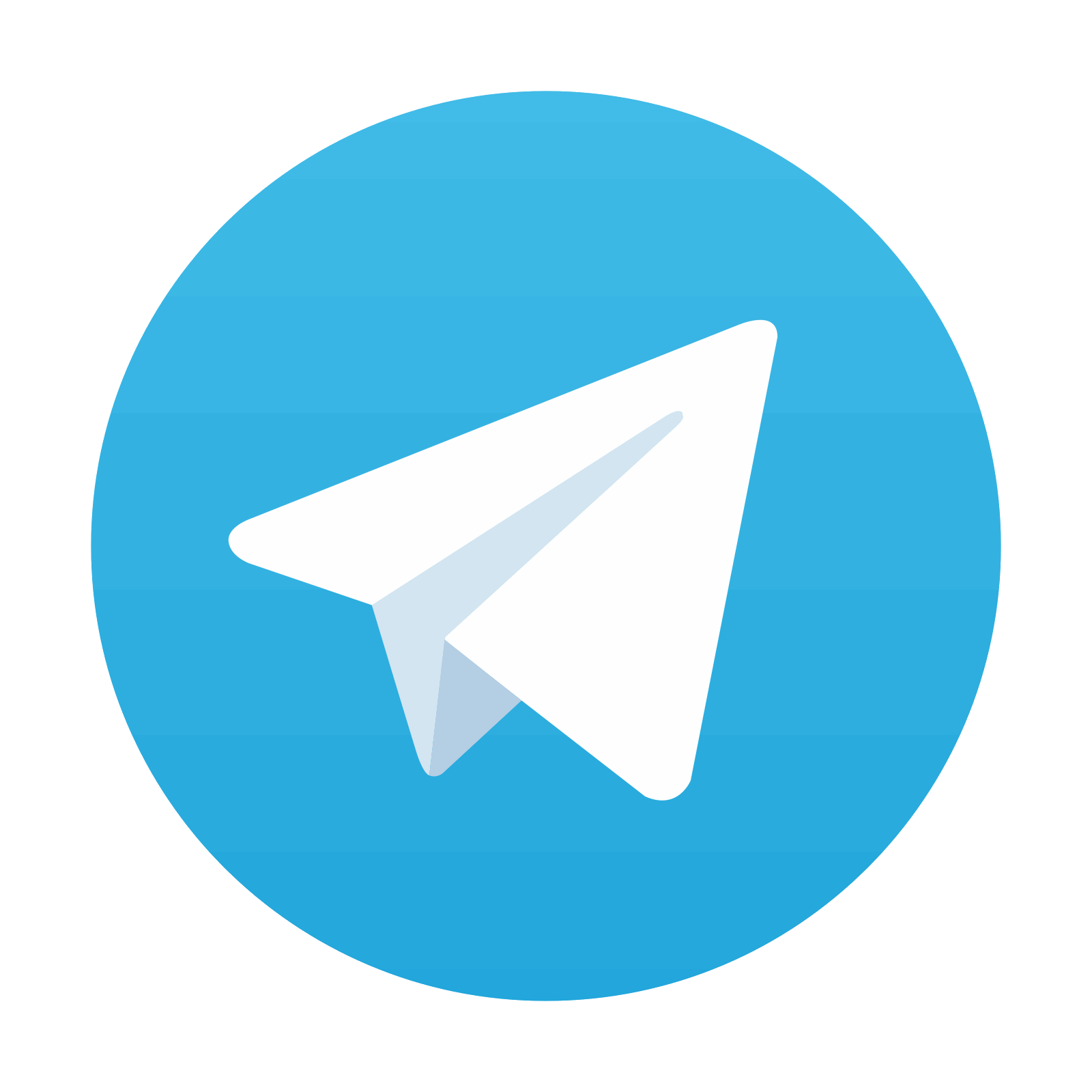
Stay updated, free articles. Join our Telegram channel

Full access? Get Clinical Tree
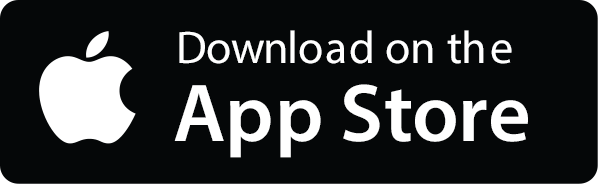
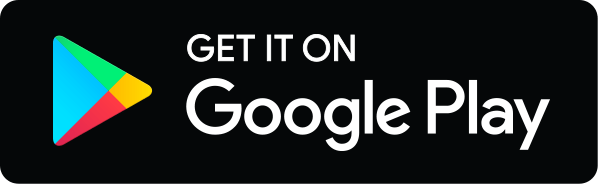
