Imaging
Clinical and physiological information
Advantages
Weakness/drawbacks
Risks and unknown
X-ray
Etiological diagnosis
PEEP adjustment (recruitment)
Detection of complications
Available at bedside
Poor sensitivity and specificity
Large interobserver variability
Radiation exposure
On-demand or routine
CT scan
Etiological diagnosis
PEEP adjustment
(homogeneity, recruitability)
Higher sensitivity
Challenges in timely follow-up
Resolution dependency
Radiation exposure
Risk of transport
Ultrasound
Etiological diagnosis
PEEP adjustment (homogeneity, recruitability)
Differentiate new comorbidity
Heart and lung interaction
Evaluation of diaphragm function
Repeatability
No radiation exposure
Technically difficult
Challenges in quantification
Tolerance
Infection
EIT
PEEP adjustment (homogeneity, recruitability)
Pulmonary perfusion
Real-time monitoring
Intuitive visual aid
No radiation exposure
Technically difficult
Affected by thoracic shape/wall
Skin injury or discomfort
PET
Regional lung perfusion
Pulmonary vascular permeability
Metabolic activity of inflammatory lung cells
Combinable with other imaging
No radiation exposure
Technically difficult
Long duration of procedure
Risk of transport
Chest X-Ray
Overview
A chest radiograph (X-ray) is fundamentally important in the diagnosis and management of PARDS, having played a key diagnostic role since the original definition of ARDS [1–3]. It has also been recognized as an important modality to ascertain and check the correct position of medical devices as well as to detect complications such as air leak. However, its poor diagnostic sensitivity, appropriate frequency of routine chest X-ray in the PARDS management, and high interobserver variability have been subjects of debate.
Diagnosis
For more than two decades, the evaluation of the extension and distribution of ARDS lung opacities was limited to chest X-rays [1]. In the Berlin ARDS definition, the radiographic criterion is more explicit, specifying that it should include bilateral opacities, consistent with pulmonary edema not fully explained by effusions, lobar/lung collapse, or nodules [2]. In adult ARDS, when the radiological criteria of the previous ARDS definition were strictly applied (bilateral chest X-ray infiltrates), the sensitivity was good, but specificity was low. ARDS does not necessarily translate into the histologic appearance of diffuse alveolar damage. The primary argument to include bilateral infiltrates in the definition of ARDS was to allow for discrimination between localized processes such as lobar pneumonia and diffuse inflammatory processes [1]. However, the sensitivity of chest X-rays is low to detect pulmonary parenchymal inflammation and edema, which compounded by high interobserver variability further compromises the value of chest X-rays in PARDS [4, 5]. For this reason and others, the new PARDS criterion advocated the removal of the requirement for bilateral infiltrates from the definition [6].
Use in Management
Generally, a chest X-ray is repeated regularly to monitor the progression of the disease, detect complications, and check the correct position of tubes and catheters. The optimal frequency of chest X-rays in children with PARDS is not established. Although some adult studies reported a possible benefit of an on-demand chest X-ray strategy to decrease its utilization in mechanically ventilated patients without affecting quality of care or safety, a recent systematic review concluded that the safety of abandoning routine chest X-rays in patients admitted to the ICU remained uncertain [7, 8]. In the pediatric cohort, routine (once a day) chest X-rays have been associated with a higher likelihood of management change or intervention. However, given the advancement of other less-invasive diagnostic imaging techniques such as ultrasonography, the necessity of routine versus adoption of on-demand chest X-rays should be considered [9].
Automation of Imaging Diagnosis
In spite of its limitations, chest X-ray continues to be an important tool in the management of PARDS, owing to its simplicity, relatively low cost, and widespread availability. A recent large international epidemiological study suggests that the proportion of patients with bilateral infiltrates increased with increasing severity of PARDS, and these were associated with higher mortality compared to unilateral infiltrates [3]. Artificial intelligence has been used to assist in standardizing the interpretation of chest X-rays and may have a role in PARDS [10].
Chest Computed Tomography
Overview
Chest Computed Tomography (CT) plays an important role in the diagnosis and management of PARDS. Chest CT can provide a better understanding of the distribution of poorly aerated pulmonary regions of PARDS lung, and the effect of gravitational forces on disease distribution.
Diagnosis
Chest CT enables a precise assessment of lung aeration, consolidation, or overdistension through direct visual analysis or quantitative measurements of Hounsfield Unit in the single voxel [11]. Lung compartments at different degrees of aeration, from totally nonaerated to hyperinflated tissue, can be identified through analysis of voxels representing a certain region of the lung [12, 13]. Modern multislice CT scanners have even better sensitivity and may give us more insights into PARDS [11].
Use in Management
Role of chest CT as a monitoring tool to guide ventilator setting and recruitability assessment has been well studied in an adult population [11, 12, 14–16]; it has been recognized as the gold standard to target PEEP and VT by assessing recruitment and hyperinflation. Modern multislice CT can be helpful in assessing the results of various recruitment maneuvers and provide more precise diagnostic information compared to single-slice CT [11, 17, 18]. In the pediatric population, only a case series examined the feasibility of evaluation of lung aeration by chest CT following a recruitment maneuver [19]; hence, the clinical impact of assessment of lung aeration in PARDS remains unclear. Because chest CT carries important risks associated with radiation exposure and the need to transport a critically ill patient to the scanner [20–23], it is not routinely performed in PARDS.
Ultrasound
Overview
Lung ultrasound is widely used in contemporary pediatric practice due to its simplicity, reproducibility, and lack of exposure radiation. It can be used in differentiating PARDS from other conditions, and in the evaluation of treatment effects. However, ultrasound evidence in PARDS is still scarce with respect to its validity and technical standardization.
Diagnosis
Anatomical structures with gas-filled spaces do not transmit ultrasound waves. Therefore, one can only obtain real structural images from a consolidated lung or a lung surrounded by pleural effusion. Air in the lung can create “artifacts” with various patterns, which could help us to understand certain pathophysiological conditions. Normal healthy or hyperinflated lungs create a horizontal pattern of artifacts parallel to the pleural line called “A-lines,” while the partial loss of aeration leads to a longitudinal laser pattern of artifacts called “B-lines.” The patterns of spatial distributions and number of B-lines can be used to assess the grades of aeration. Particularly, we can see multiple B-lines in the ARDS lung with a heterogeneous and non-gravity-dependent distribution, pleural thickening, and diminished lung sliding [13, 24, 25]. Ultrasound images can be semiquantitatively assessed using the four sonographic findings: normal pattern, multiple spaced B-lines, coalescent B-lines, and consolidation [25].
Use in Management
Lung ultrasound can be used to monitor aeration changes and effects of therapy including recruitment maneuver. In adult patients, lung ultrasound has been well validated to detect conditions such as pleural effusions, cardiogenic pulmonary edema, alveolar–interstitial syndrome, ventilator-associated pneumonia, pleural effusion, and pneumothorax, and compares favorably to chest X-ray in critically ill patients, with very high diagnostic accuracy, specifically for both pneumothorax and pleural effusions [13, 24–29]. It can also be used to assess heart–lung interactions, including the intracardiac right-to-left shunting to guide therapeutic interventions in ARDS patients. Nonetheless, the evidence for routine use of lung ultrasound in the pediatric population is still somewhat limited [30, 31].
Lung reaeration by ultrasound can be assessed by tracking changes in sonographic findings, including evaluation of response to recruitment maneuvers. For instance, a patient with ARDS with diffuse loss of aeration has a higher level of PEEP-induced lung recruitment when compared to patients with focal loss of aeration. A-lines correspond to normally aerated or hyperinflated lung, well-spaced B lines correspond to moderate loss of aeration, coalescent B-lines correspond to severe loss of aeration, and a tissue-like pattern indicates complete loss of aeration.
Lung ultrasound can be performed at the bedside, thus avoiding the possible risks associated with transporting unstable children. It could also lead to a reduction in the number of chest X-rays and CT scans, thus reducing radiation exposure. Lung overinflation, technical difficulty with subcutaneous emphysema, the presence of large thoracic dressings (e.g., patients following chest trauma or burns), or marked obesity can limit the performance of lung ultrasound. Proper training is required to correctly perform and interpret the findings of a lung ultrasound examination. Lung ultrasound usually takes longer to perform compared to other imaging modalities, such as X-ray, an issue that could limit its tolerability by some of the sicker patients with PARDS.
Ultrasound of Diaphragm
Evaluation of the diaphragm by ultrasound has recently become a common practice in the PICU. Abnormal diaphragmatic imaging (i.e., thickness and motility) can be observed in conditions such as phrenic nerve injury or prolonged mechanical ventilation in PARDS [32].
Electric Impedance Tomography
Overview
Electric Impedance Tomography (EIT) is a noninvasive bedside monitoring technique that enables dynamic real-time evaluation of lung volume and regional lung changes. EIT quantifies relative changes in local impedance of lung; therefore, normal or pathologically stable structures such as pleural effusions are functionally muted and are not visualized. In other words, the lung is a good candidate for EIT because its impedance is closely related to the degree of parenchymal inflation. In children, typically, chest EIT can be performed by placing an electrode belt around the thorax, between the third and the sixth intercostal spaces. The resulting images represent the impedance changes occurring in a cross-section of the thorax [33–35].
Use in PARDS
EIT can detect existing heterogeneity in regional ventilation during mechanical ventilation and during recruitment maneuvers. Changes in EIT patterns can distinguish between recruited and derecruited lung regions. Decreased lung aeration in dependent regions and compensatory overdistension in nondependent regions can be seen in EIT during the progression of PARDS. EIT has also been studied to assess the regional distribution of pulmonary perfusion and its relationship with lung ventilation [36–39].
EIT has been well validated in an adult subjects against other imaging techniques that assess lung volume. However, several factors may affect its output and resolution, such as electrode position, conformational changes of chest wall, and diaphragm. Research focusing on improving image reconstruction algorithms, creating dedicated algorithms for specific clinical applications, improving its connectivity with modern mechanical ventilators (including noninvasive ventilators), and developing ways of reducing artifacts due to the changes in thoracic shape, is ongoing [40].
Positron Emission Tomography
Positron emission tomography (PET) is a functional imaging technique based on the administration of a molecule labeled with a radioactive isotope that decays with the emission of a positron. PET is versatile; it can quantify regional perfusion, pulmonary vascular permeability, ventilation, aeration, metabolic activity of inflammatory cells, enzyme activity, and pulmonary gene expression. Combining PET measurements of perfusion and shunt can identify redistribution of perfusion toward derecruited regions as the mechanism for the worsening oxygenation that is sometimes observed with recruitment maneuvers or application of PEEP. One should be cautious when using PaO2 as an index of PARDS severity or response to recruitment strategies if the distribution of perfusion is unknown [41–43].
While routine PET scanning of PARDS patients is not feasible, PET could be useful in selected cases to detect early PARDS development, or to monitor the efficacy of anti-inflammatory treatment. Combined availability of PET with other imaging modalities such as CT scan or Magnetic Resonance Imaging (MRI) could make multimodality imaging a valuable tool to further our understanding of PARDS.
Part Two: Nonimaging Monitoring
Parameters monitored in the management of children with acute respiratory distress syndrome (hemodynamic monitoring excluded)
Monitoring and measurement | Clinical indication and information | Equipment required | Specific considerations |
---|---|---|---|
Respiratory system variables derived from additional monitoring | |||
Lung compliance | Severity assessment | Pressure and tidal volume on the ventilator | Dynamic compliance Plateau pressure measure |
Esophageal manometry | Measuring transpulmonary pressure PEEP adjustment Prevention of ventilator induced lung injury | Esophageal balloon Pressure monitor or ventilator | Technical difficulty |
Work of breathing, pressure–rate product | Adequacy of support PEEP adjustment | Esophageal balloon Pressure monitor or ventilator | Technical difficulty |
Corrected minute ventilation | Severity assessment | Ventilator ABG or CBG | Standardized minute ventilation of children: Approximately 150 mL/kg/min Largely a research tool |
Functional residual capacity | Severity assessment PEEP adjustment | Ventilator with special function or dedicated device | Simple and automated bedside techniques required |
Ratio dead space/tidal volumes (VD/VT) | Severity assessment and disease progress Weaning success indicator | Volumetric capnography ABG or CBG | End-tidal CO2 should be used as a reliable surrogate for arterial or capillary CO2 when a correction method is validated Peripheral venous blood gas also does not accurately predict arterial gas |
Alveolar dead space fraction | Severity assessment and disease progress | Volumetric or time-based capnography ABG or CBG | |
Respiratory inductance plethysmograph | Estimating ventilator volume and work of breathing Apnea detection | Respiratory inductance plethysmography | Dual and single band techniques Challenging to control artifact in nonsedated patients |
3D computed imaging system | Estimating ventilator volume and work of breathing Apnea detection | Specially equipped bedside video cameras | Contactless automated techniques |
Oxygenation and ventilation parameters and severity scores | |||
P/F and OI | Severity assessment Diagnosis and prognosis assessment | ABG Ventilator | Requiring arterial blood gas sampling Patients need to be on ventilator for OI |
S/F ratio and OSI | Severity assessment Diagnosis and prognosis assessment | Ventilator | Patients need to be on ventilator for OSI |
Lung injury score | Severity assessment | Chest x-ray ABG Compliance | Noninvasive score using oxygen saturation for PaO2 |
Ventilation index | Severity assessment | ABG or CBG | Arterial blood gas required and patients need to be on ventilator |
Continuous arterial gas monitoring | Ventilator settings and adjustment | Specific arterial catheter | No widely available device |
Continuous CO2 monitoring | Accuracy of the support | Ventilator, monitor, or specific analyzer | Capnography or transcutaneous monitor can be used |
Respiratory muscle activity | |||
P0.1 | Respiratory drive Extubation readiness | Ventilator with specific function | Need to be on mechanical ventilation to measure non-invasive P0.1 |
Tension–time index | Respiratory drive Weaning strategy | Esophageal and gastric pressure catheter | Need to be on mechanical ventilation to measure non-invasive TTI |
PEmax, PImax Sniff Pdi, Gilbert index | Response of respiratory muscle training | Ventilator Pressure measuring device | Voluntary measurements of maximum Pdi: Sniff procedure Gilbert index: Relative contribution of the diaphragm to inspiration |
Electrical activity of diaphragm | Respiratory drive (phasic activity); synchronization PEEP adjustment (tonic activity) | Ventilator with specific function Special nasogastric tube | VT/EAdi: Neuroventilatory efficiency (NVE) |
Phrenic nerve stimulation | Function of the diaphragm | Needle and implanted wire stimulation (invasive, not recommended) Transcutaneous electrical stimulation Magnetic stimulation |
Respiratory Variables Derived from Additional Monitoring
Lung Compliance
In complement to the tidal volume and positive inspiratory pressure, these are continuously monitored on the ventilator; monitoring of respiratory system compliance can help assess PARDS progression. In clinical practice, the dynamic compliance measurement [Vt (ml/kg ideal body weight)/(PIP-PEEP (cmH2O)] (normal range 1.5–2 ml/kg/cmH2O) is preferred to static or quasistatic compliance measurements that are more difficult to obtain. Of note, although the PIP can be used to calculate compliance in patients undergoing pressure-controlled ventilation, the plateau pressure (Pplat) is preferred in patients undergoing volume-controlled ventilation. The Pplat should be measured during an end-inspiratory hold and in the absence of active spontaneous breathing [44].
Transpulmonary Pressure
During mechanical ventilation, transpulmonary pressure measurements can be estimated as the airway pressure (Paw) (a surrogate for alveolar pressure) minus the esophageal pressure (Pes) (a surrogate for pleural pressure). Transpulmonary pressure, the pressure across the lung that gives rise to pulmonary ventilation, is crucial to our understanding of respiratory mechanics and clinical decision makingregarding PEEP adjustment in PARDS. Although transpulmonary pressure has been well studied in the adult population, limited evidence exists in patients with PARDS [45–50]. We can calculate work of breathing from intrinsic PEEP or any cause of decreased respiratory system compliance by using the Campbell diagram, or the pressure–rate product (PRP; esophageal pressure change x respiratory rate) or pressure–time product [51]. The role of these variables considering different levels of PEEP and tidal volume on the partitioned respiratory mechanics, stress (i.e., transpulmonary pressure at the end of inspiration), and strain (the change in volume to the functional residual capacity) is unknown in PARDS.
Functional Residual Capacity
Optimizing Functional residual capacity (FRC) is critically important in the management of patients with PARDS because of the smaller elastic retraction forces and a lower relaxation volume that makes them more prone to airway collapse. FRC can be affected by the ventilator mode and settings such as PEEP, respiratory rate, and I:E ratio [52, 53]. Although assessing and understanding FRC could lead to a physiology-cased strategy for managing PARDS, its applicability has been limited due to lack of reliable and straightforward bedside measuring techniques [54].
Ratio Dead Space/Tidal Volumes, Alveolar Dead Space Fraction
Physiologic dead space is the sum of anatomical dead space (volume of the conducting airways not participating in gas exchange) and alveolar dead space (volume of gas in the respiratory zone that does not participate in gas exchange). Abnormalities of pulmonary blood flow and injury to the microcirculation are a characteristic feature of PARDS; hence, physiological dead space is expected to be elevated [55–61]. Capnography enables the calculation of the ratio of dead space to tidal volume, end-tidal alveolar dead space fraction (AVDSf), and ventilation index [62]. The ratio of dead space to tidal volume can be measured using volumetric capnography and PaCO2 from a properly timed blood sample. It can be calculated using such as the Enghoff modification of the Bohr equation [63]. Similar information can be obtained using the end-tidal AVDSf where end-tidal CO2 is substituted for the mixed expired CO2, although there may be situations when AVDSf may not be a good surrogate for VD/VT, such as in patients with lower airway obstruction.
Respiratory Inductive Plethysmography
Respiratory inductive plethysmography (RIP) measurements of timed respiratory mechanics, including tidal flow volume loops and thoraco-abdominal asynchrony, reflect respiratory system resistance and compliance. RIP is relatively simple to perform and only requires two elastic bands placed around the chest and abdomen (i.e., dual band measurement). Although it is not designed as a monitor and has possible drawbacks such as discomfort due to the placement of the probes, RIP may provide a useful measure of respiration in conscious patients, especially those undergoing noninvasive ventilation, such as high flow nasal cannula, where one would otherwise lack the ability to measure tidal volume [64–68].
3D Computed Imaging System
While respiratory rate (RR) and ventilator tidal volume can be easily measured by the mechanical ventilator for the intubated patient, assessment of minute ventilation and effort of breathing of the spontaneously breathing patient is more challenging. Many techniques of noninvasive respiration monitoring have been reported, but most of them are unsuitable for the clinical environment due to the size of the machine or poor patient tolerance [69]. A novel noninvasive contactless 3D imaging system that captures motion data for the anterior and lateral surfaces of the torso and is capable of measuring respiratory variables, including spontaneous tidal volume, has been developed [70].
Oxygenation and Ventilation Parameters and Severity Scores
P/F and S/F Ratio, Oxygenation and Oxygen Saturation Index
Initial degree of hypoxemia correlates well with outcomes in PARDS. Several indexes aiming at evaluating the degree of hypoxia in PARDS have been studied and are routinely used in clinical practice, such as P/F ratio (PaO2:FIO2 ratio), oxygen index (OI = FIO2 × Paw/PaO2; Paw: mean airway pressure), and oxygen saturation index (OSI: FIO2 × Paw/SpO2).
PaO2/FIO2 Ratio
Partial pressure of oxygen (PaO2)/fraction of inspired oxygen (FIO2) ratio has been widely used in the diagnosis and management of PARDS [71]. Given the challenges of placing arterial catheters in small children, especially when patients are not sedated or mechanically ventilated, PaO2 measurements are not routinely and practically obtainable. Therefore, the utility of P/F ratio in children has been questioned, particularly as a disease indicator or prognostic factor [59, 72–74].
Oxygen Index
OI has been used as an entry criterion and to stratify risk in PARDS studies. OI has an inherent advantage over P/F ratio in that it incorporates Paw (a measure of respiratory support) and may have a more reliable correlation than P/F ratio with mortality in children [72, 75–77]. In a single-center cohort study, it is suggested that OI could reflect the severity of oxygenation failure at any point in time in acute hypoxemic respiratory failure and does have an impact on length of mechanical ventilation and mortality. In a recent international epidemiological study, PARDS severity groups proposed by PALICC using OI measurements rather than P/F ratio have been shown to have important prognostic relevance, with patients with higher OI being at particularly high risk [3].
SpO2/FIO2 Ratio and Oxygen Saturation Index
Pulse oximetry is now widely used in PICUs, which has led to a reduced frequency of peripheral arterial catheter utilization [75, 78]. This is particularly true in patients suffering from mild or moderate PARDS. In children without an arterial line, PaO2 cannot be easily measured, so the P/F ratio and OI cannot be calculated. In other words, children with mild-to-moderate hypoxemia that would likely meet Berlin criteria may not be screened for PARDS due to the lack of a documented qualifying PaO2. Because the oxyhemoglobin dissociation curve is nearly linear when SpO2 is 80–97%, SpO2 has been proposed as a substitute for PaO2 in PARDS. A prospective study in adults with ARDS suggests that SpO2/FIO2 (S/F) ratio can be a reliable surrogate for the P/F ratio when SpO2 is ≤97% [79]. A PARDS retrospective study has shown similar results, suggesting a positive association with mortality [76]. This study also demonstrates that OSI, which substitutes SpO2 for PaO2 in the OI equation, is an acceptable surrogate for P/F ratio in PARDS, with a reasonably good sensitivity and specificity, and good correlation with mortality [76].
Lung Injury Score and Ventilation Index
Various physiological measurements and scoring systems have been proposed and assessed for grading severity of illness in PARDS and predicting mortality. The lung injury score (LIS), proposed by Murray et al., has been commonly utilized to assess severity of illness in studies of adult ARDS, and has also been validated in PARDS [76, 77]. A higher LIS could identify the need for deploying rescue therapies, and changes in LIS over time have also been used as a primary outcome to study the efficacy of certain interventions [80, 81]. A large cohort study of adult ARDS reported that both LIS and the Berlin definition severity categories were associated with increased in-hospital morbidity and mortality. However, the predictive validity of both scores was marginal and there was no additive value of LIS over the Berlin criteria [81]. Noninvasive LIS applying S/F for P/F ratio has also been validated in PARDS [77]. Ventilation index (VI) (PaCO2 (in mm Hg) × peak airway pressure (in cmH2O) × respiratory rate (breaths/min)/1000) has been shown to have prognostic value in PARDS [62, 82]. A small single-center study reported that higher VI (>65) could be a reliable prognostic indicator in PARDS.
Continuous Arterial Gas Monitoring
Peripheral blood gas sampling does not accurately predict arterial blood gases. Frequent blood gas sampling can be challenging, considering the risk of iatrogenic anemia, particularly in small infants. Arterial blood gases can be continuously monitored using special arterial catheters and have been studied in the research arena [83–89]. Although several prospective studies in neonates attest to the accuracy and reproducibility of an intra-arterial continuous monitoring device (Paratrend7; Diametrics Medical Inc., United Kingdom), it has subsequently been withdrawn from the market and no comparable devices exist as of today for this purpose [83, 85, 89].
Continuous CO2 Monitoring
Carbon dioxide can be noninvasively monitored by capnography when patients are on conventional mechanical ventilation. End-tidal CO2 monitoring can provide useful and predictive information, including dead space evaluation. However, that is not the case during high-frequency oscillatory ventilation. Although it has not been well studied in PARDS, transcutaneous CO2 monitoring can substitute arterial CO2 or mixed venous CO2 in continuous monitoring [90–96].
Respiratory Muscle Function
Respiratory muscle dysfunction is known to develop in mechanically ventilated patients. To that effect, some have advocated maintaining patient breathing effort during mechanical ventilation; yet due to the limited availability and knowledge of tools to monitor respiratory muscle function in PARDS, evidence regarding clinical effect and benefits of such a strategy are still very limited.
Neural Respiratory Drive (P0.1)
Respiratory drive measurement may be used to evaluate extubation readiness. Airway pressure (Paw) generated in the first 100 ms of inspiration (P0.1) has been used as an index of neural respiratory drive. This delay prevents any reaction from the patient in response to occlusion. It can be measured in most conventional mechanical ventilators and has been successfully used in critically ill ventilated children. However, the value of P0.1 in predicting extubation readiness has been reported with conflicting results [97–100].
Tension–Time Index
Understanding the effectiveness of breathing effort and load on the respiratory muscles is crucial when predicting extubation readiness. The Tension–time index (TTI) of the diaphragm (TTdi) is derived by relating the mean transdiaphragmatic pressure per breath (Pdi) to the maximal inspiratory transdiaphragmatic pressure (Pdimax) and the inspiratory time (Ti) to the total respiratory cycle time (Ttot) [101–103]. It can be a fraction of maximal effort that the diaphragm performs during the contraction time. The difference between esophageal (Pes) and gastric pressure (Pga) is used to calculate Pdi. Measurement of the TTdi requires the use of balloon catheters. A noninvasive TTI measurement (TTmus), based on airway pressure, has been developed and studied in children [104, 105]. One of the advantages of TTmus is that it reflects the contribution of all the inspiratory muscles, rather than being specific to the diaphragm muscle. Measurement of TTmus would be useful in a variety of settings such as in selecting a strategy for weaning from prolonged mechanical ventilation.
Pressure and Flow Recordings
A maximal static inspiratory and expiratory maneuver can be obtained in mechanically ventilated patients to evaluate global inspiratory (PImax) and expiratory (PEmax) muscle strength. PImax and PEmax can be used as a measure for respiratory muscle function and possibly to monitor the response of respiratory muscle training. They can also be measured during brief disconnection by using a handheld pressure-monitoring device, which requires patient cooperation [106].
Voluntary measurements of maximum Pdi can be obtained by having the patients inspire as forcefully as possible against a closed airway or by having the patient sniff forcibly. Sniff Pdi appears to be more reproducible than maximum inspiratory Pdi [107]. The Gilbert index can be used to determine the relative contribution of the diaphragm to inspiration [28]. The higher the index, the greater is the contribution of the diaphragm to the total inspiratory effort. One must keep in mind that the index can be a negative value with a paralyzed diaphragm. To estimate the energy expenditure of the diaphragm, the TTI and pressure–time product of the diaphragm can be calculated using Pdi. These indices are frequently used for research purposes, but without dedicated software, they are impractical for routine clinical use. However, one should keep in mind that Pdi is influenced by the positive pressure of the mechanical ventilator, so ideally, it should be measured during spontaneous breathing.
Electrical Activity of Diaphragm
Electrical Activity of Diaphragm (EAdi) is a method of monitoring respiratory muscle unloading and patient-ventilator synchrony. EAdi has been validated in clinical practice using a technology developed for the neurally adjusted ventilator assist (NAVA) mode of ventilation [108–115]. EAdi reflects the respiratory drive and is correlated with other indicators such as P0.1. EAdi has a wide range of application in PARDS management. Visual inspections of flow and pressure waveforms using EAdi can be used to detect patient–ventilator asynchrony. Increased value of EAdi during expiration period may suggest increased efforts to create larger end-expiratory lung volume [108, 116, 117]. EAdi may also help in the monitoring of respiratory muscle loading, patient–ventilator synchrony, and efficiency of breathing in critically ill patients. The ratio of actual EAdi to peak EAdi, observed during a 20-second inspiratory occlusion, is a measure of the patient’s breathing effort. A small ratio suggests excessive ventilatory support, whereas a larger ratio indicates inadequate unloading of the respiratory muscles. The ratio between VT and EAdi also represents neuroventilatory efficiency (NVE) of the diaphragm. An improved NVE can indicate that a patient generates adequate VT with a lower level of EAdi, while a higher value of EAdi suggests the opposite. NVE is affected by changes in diaphragm function and a patient’s load of breathing. Decreased value of a ratio between Pdi and EAdi (i.e., neuromechanical efficiency) indicates the development of diaphragm weakness, whereas an increase value suggests a recovery.
Phrenic Nerve Stimulation
Phrenic nerve stimulation can provide valuable information regarding the mechanical function of the diaphragm, and how its contractile force is transformed into pressure. Four techniques have been developed: needle stimulation and implanted wire stimulation are both invasive and currently not recommended, while transcutaneous electrical stimulation and magnetic stimulation have been more extensively studied and have minimal reported side effects [118, 119]. The integrity of the phrenic nerve in response to stimulation can be used to calculate phrenic nerve conduction time, which helps in the detection of phrenic nerve injury, and currently is extensively used in research settings [118–121].
Conclusion
Chest X-ray is currently mandatory for the diagnosis of PARDS and to detect complications such as air leak or equipment displacement but lacks interrater reliability. Various other imaging techniques are being studied in PARDS research but are not routinely performed. The monitoring of vital signs and ventilator parameters, including oxygenation and CO2 removal, is important in the assessment of PARDS severity and to guide management. The monitoring of various respiratory system parameters requires continued development so as to be useful in children, and further research is needed to assess their potential impact in patients with PARDS.

Full access? Get Clinical Tree
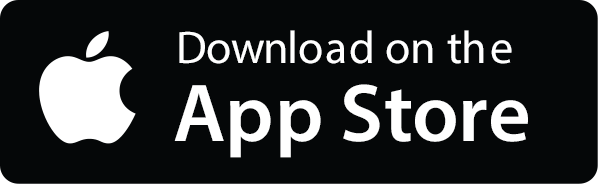
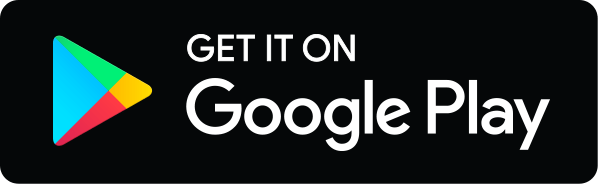